Recent cell printing systems for tissue engineering
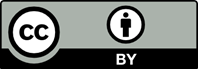
Three-dimensional (3D) printing in tissue engineering has been studied for the bio mimicry of the structures of human tissues and organs. Now it is being applied to 3D cell printing, which can position cells and biomaterials, such as growth factors, at desired positions in the 3D space. However, there are some challenges of 3D cell printing, such as cell damage during the printing process and the inability to produce a porous 3D shape owing to the embedding of cells in the hydrogel-based printing ink, which should be biocompatible, biodegradable, and non-toxic, etc. Therefore, researchers have been studying ways to balance or enhance the post-print cell viability and the print-ability of 3D cell printing technologies by accommodating several mechanical, electrical, and chemical based systems. In this mini-review, several common 3D cell printing methods and their modified applications are introduced for overcoming deficiencies of the cell printing process.
1. Whitaker M, 2014, The history of 3D printing in healthcare, Annals of The Royal College of Surgeons of England Bulletin, vol.96: 228-229.
https://doi.org/10.1308/147363514X13990346756481
2. Griffith LG, Naughton G, 2002, Tissue engineering - Current challenges and expanding opportunities, Science, vol.295(5557): 1009-1014.
https://doi.org/10.1126/ science.1069210
3. Hollister SJ, 2005, Porous scaffold design for tissue engineering, Nature Materials, vol.4(7): 518-524.
https://doi.org/10.1038/nmat1421
4. Whitford WG, 2016, Bioinks for 3D Bioprinting: A development parameters review, Immunome Research, vol.12(S2): 24. https://doi.org/10.4172/1745-7580.c1.004
5. Loo Y, Lakshmanan A, Ni M, et al. 2015, Peptide bioink: Self-assembling nanofibrous scaffolds for three-dimensional organotypic cultures, Nano letters, vol.15(10): 6919-6925. https://doi.org/10.1021/acs.nanolett.5b02859
6. Mironov V, Reis N, Derby B, 2006, Bioprinting: A Beginning, Tissue engineering, vol.12: 4.
https://doi.org/10.1089/ten.2006.12.631
7. Sun W, Lal P, 2002, Recent development on computer aided tissue engineering - A review, Computer Methods and Programs in Biomedicine, vol.67(2): 85-103.
https://doi.org/10.1016/S0169-2607(01)00116-X
8. Sachlos E, Czernuszka JT, 2003, Making tissue engineering scaffolds work. Review: The application of solid freeform fabrication technology to the production of tissue engineering scaffolds, European Cells & Materials, vol.5: 29-39. https://doi.org/10.22203/eCM.v005a03
9. Hutmacher DW, Sittinger M, Risbud MV, 2004, Scaffold-based tissue engineering: Rationale for computer-aided design and solid free-form fabrication systems, Trends in Biotechnology, vol.22(7): 354-362.
https://doi.org/10.1016/j.tibtech.2004.05.005
10. Fedorovich NE, Schuurman W, Wijnberg HM, et al. 2012, Biofabrication of osteochondral tissue equivalents by printing topologically defined, cell-laden hydrogel scaf-folds, Tissue Engineering Part C: Methods, vol.18(1): 33-44. https://doi.org/10.1089/ten.tec.2011.0060
11. Guillotin B, Guillemot F, Cell patterning technologies for organotypic tissue fabrication, Trends in Biotechnology, vol.29(4): 183-190.
https://doi.org/10.1016/j.tibtech.2010.12.008
12. Seol YJ, Kang HW, Lee SJ, et al. 2014, Bioprinting technology and its applications, European Journal Cardio-Thoracic Surgery, vol.46(3): 342-348.
https://doi.org/10.1093/ejcts/ezu148
13. Wilson WC, Boland T, 2003, Cell and Organ Printing 1: Protein and Cell Printers, The Anatomical Record Part A, 272A: 491-496. https://doi.org/10.1002/ar.a.10057
14. Dababneh AB, Ozbolat IT, 2014, Bioprinting technology: A current state-of-the-art review, Journal of Manufacturing Science and Engineering, vol.136(6): 061016.
https://doi.org/10.1115/1.4028512
15. Seol YJ, Kang HW, Lee SJ, et al. 2014, Bioprinting technology and its applications, European Journal of Cardio-Thoracic Surgery, 1-7, ezu148.
16. Murphy SV, Atala A, 2014, 3D bioprinting of tissues and organs, Nature Biotechnology, vol.32(8): 773-785.
https://doi.org/10.1038/nbt.2958
17. Guillemot F, Guillotin B, Catros S, et al. 2010, High-throughput biological laser printing: droplet ejection mechanism, integration of a dedicated workstation, and bioprinting of cells and biomaterials, Cell and Organ Printing, 95-113.
18. Roda A, Guardigli M, Russo C, et al. 2000, Protein microdeposition using a conventional ink-jet printer, Biotechniques, vol.28(3): 492–496.
19. Barron J, Ringeisen B, Kim H, et al. 2004, Application of laser printing to mammalian cells, Thin Solid Films, vol.453(383): 383–387.
https://doi.org/10.1016/j.tsf.2003.11.161
20. Hon K, Li L, Hutchings I, 2008, Direct writing technology—advances and developments, CIRP Annals - Manufacturing Technology, vol.57(2): 601–620.
21. Guillotin B, Souquet A, Catros S, et al, 2010, Laser assisted bioprinting of engineered tissue with high cell density and microscale organization, Biomaterials, vol.31(28): 7250–7256.
https://doi.org/10.1016/j.biomaterials.2010.05.055
22. Krishnan UM, Sethuraman S, 2013, The integration of nanotechnology and biology for cell engineering: Promises and challenges, Nanomaterials and Nanotechnology, vol.3(Godište 2013): 3-19.
23. Xu T, Gregory A, Molnar P, et al. 2006, Viability and electrophysiology of neural cell structures generated by the inkjet printing method, Biomaterials, vol.27(19): 3580–3588. https://doi.org/10.1016/j.biomaterials.2006.01.048
24. Xu T, Jin J, Gregory C, et al. 2005, Inkjet printing of viable mammalian cells, Biomaterials, vol.26(1): 93–99. https://doi.org/10.1016/j.biomaterials.2004.04.011
25. Moon S, Hasan K, Song S, et al. 2010, Layer by layer three-dimensional tissue epitaxy by cell-laden hydrogel droplets, Tissue Engineering Part C: Methods, vol.16(1): 157–166. https://doi.org/10.1089/ten.tec.2009.0179
26. Xu T, Zhao W, Zhu J, 2013, Complex heterogeneous tissue constructs containing multiple cell types prepared by inkjet printing technology, Biomaterials, vol.34(1): 130–139.
https://doi.org/10.1016/j.biomaterials.2012.09.035
27. Calvert P, 2001, Inkjet printing for materials and devices, Chemistry of Materials, vol.13(10): 3299–3305.
https://doi.org/10.1021/cm0101632
28. Knowlton S, Onal S, Yu CH, et al. 2015, Bioprinting for cancer research, Trends in Biotechnology, vol.33(9): 504-513. https://doi.org/10.1016/j.tibtech.2015.06.007
29. Ozbolat I, Yu Y, 2013, Bioprinting toward organ fabrication: Challenges and future trends, IEEE Transactions on Biomedical Engineering, vol.60(3): 691–699.
https://doi.org/10.1109/TBME.2013.2243912
30. Nair K, Yan K, Sun W, 2007, A multi-level numerical model for quantifying cell deformation in encapsulated alginate structures, Joruanl of Mechanics of Materials and Structures, vol.2(6): 1121–1139.
https://doi.org/10.2140/jomms.2007.2.1121
31. Shim J, Lee J, Kim J, et al. 2012, Bioprinting of a mechanically enhanced three-dimensional dual cell-laden construct for osteochondral tissue engineering using a multi-head tissue/organ building system, Journal of Micromechanics and Microengineering, vol.22(8): 085014.
https://doi.org/10.1088/0960-1317/22/8/085014
32. Yu Y, Zhang, Y, Martin J, et al. 2013, Evaluation of cell viability and functionality in vessel-like bioprintable cell-laden tubular channels, Journal of Biomechanical Engineering, vol.135(9): 091011.
https://doi.org/10.1115/1.4024575
33. Tirella A, Vozzi F, Vozzi G. 2011, PAM2 (Piston Assisted Microsyringe): A New Rapid Prototyping Technique for Biofabrication of Cell Incorporated Scaffolds, Tissue Engineering Part C: Methods, vol.17(2): 229–237.
https://doi.org/10.1089/ten.tec.2010.0195
34. Koo Y, Kim G, 2016, New strategy for enhancing in situ cell viability of cell printing process via piezoelectric transducer-assisted three-dimensional printing, Biofabrication, vol.8(2): 025010.
https://doi.org/10.1088/1758-5090/8/2/025010
35. Chang CC, Boland ED, Williams SK, et al. 2011, Direct‐write bioprinting three‐dimensional biohybrid systems for future regenerative therapies, Journal of Biomedical Materials Research Part B: Applied Biomaterials, vol.98(1): 160-170. https://doi.org/10.1002/jbm.b.31831
36. Ahn S, Lee H, Bonassar LJ, et al. 2012, Cells (MC3T3-E1)-Laden Alginate Scaffolds Fabricated by a Modified Solid-Freeform Fabrication Process Supple-mented with an Aerosol Spraying, Biomacromolecules, vol.13(9): 2997-3003. https://doi.org/10.1021/bm3011352
37. Ahn S, Lee H, Puetzer J, et al. 2012, Fabrication of cell-laden three-dimensional alginate-scaffolds with an aerosol crosslinking process, Journal of Materials Chemistry, vol.22(36): 18735-18740.
https://doi.org/10.1039/c2jm33749e
38. Ahn S, Lee H, Kim G, 2013, Functional cell-laden alginate scaffolds consisting of core/shell struts for tissue regeneration, Carbohydrate Polymers, vol.98(1): 936-942. https://doi.org/10.1016/j.carbpol.2013.07.008
39. Xu T, Jalota S, Manley B, et al. 2005, Drop-on Demand Printing of Cell and Materials for Designer Hybrid Cardiovascular Biomaterials, Society for Imaging Science and Technology, NIP & Digital Fabrication Conference, 178-178
40. Boland T, Tao X, Damon BJ, et al. 2007, Drop-on-demand printing of cells and materials for designer tissue constructs, Materials Science and Engineering: C, vol.27(3): 372-376.
https://doi.org/10.1016/j.msec.2006.05.047
41. Xiong R, Zhang Z, Chai W, et al. 2015, Freeform drop-on-demand laser printing of 3D alginate and cellular constructs, Biofabrication, vol.7(4): 045011.
https://doi.org/10.1088/1758-5090/7/4/045011
42. You F, Wu X, Zhu N, et al. 2016, 3D Printing of Porous Cell-Laden Hydrogel Constructs for Potential Applications in Cartilage Tissue Engineering, ACS Biomaterials Science & Engineering, vol.2(7): 1200-1210.
https://doi.org/10.1021/acsbiomaterials.6b00258
43. Gao Q, He Y, Fu J-z, et al. 2015, Coaxial nozzle-assisted 3D bioprinting with built-in microchannels for nutrients delivery, Biomaterials, vol.61: 203-215.
https://doi.org/10.1016/j.biomaterials.2015.05.031
44. Perez RA, Kim M, Kim TH, et al. 2013, Utilizing core–shell fibrous collagen-alginate hydrogel cell delivery system for bone tissue engineering, Tissue Engineering Part A, vol.20(1-2): 103-114.
https://doi.org/10.1089/ten.tea.2013.0198
45. Faulkner-Jones A, Fyfe C, Cornelissen DJ, et al. 2015, Bioprinting of human pluripotent stem cells and their directed differentiation into hepatocyte-like cells for the generation of mini-livers in 3D, Biofabrication, vol.7(4): 044102. https://doi.org/10.1088/1758-5090/7/4/044102
46. Ahn SH, Lee HJ, Lee JS, et al. 2015, A novel cell printing method and its application to hepatogenic differentiation of human adipose stem cell-embedded mesh structures, Science Reports-UK, vol.5:13427
47. You F, Wu X, Chen X, 2016, 3D Printing of Porous Alginate/gelatin Hydrogel Scaffolds and Their Mechanical Property Characterization, International Journal of Polymeric Materials and Polymeric Biomaterials, In-Press.
48. Pati F, Jang J, Ha DH, et al. 2014, Printing three-dimensional tissue analogues with decellularized extracellular matrix bioink, Nature Communications, vol.5: 3935.
https://doi.org/10.1038/ncomms4935
49. Yoon H, Lee JS, Yim H, et al. 2016, Development of cell-laden 3D scaffolds for efficient engineered skin substitutes by collagen gelation, RSC Advances, vol.6(26): 21439-21447. https://doi.org/10.1039/C5RA19532B
50. Ahn S, Lee H, Lee EJ, et al. 2014, A direct cell printing supplemented with low-temperature processing method for obtaining highly porous three-dimensional cell-laden scaffolds. J Mater Chem B, vol.2(18): 2773-2782.
https://doi.org/10.1039/c4tb00139g
51. Yeo M, Kim G, 2015, Fabrication of cell-laden electrospun hybrid scaffolds of alginate-based bioink and PCL microstructures for tissue regeneration, Chemical Engineering Journal, vol.275: 27-35.
https://doi.org/10.1016/j.cej.2015.04.038
52. Yeo M, Ha J, Lee H, et al. 2016, Fabrication of hASCs-laden structures using extrusion-based cell printing supplemented with an electric field, Acta biomaterialia, vol.38: 33-43.
https://doi.org/10.1016/j.actbio.2016.04.017
53. Shim J-H, Lee J-S, Kim JY, et al. 2012, Bioprinting of a mechanically enhanced three-dimensional dual cell-laden construct for osteochondral tissue engineering using a multi-head tissue/organ building system, Journal of Micromechanics and Microengineering, vol.22(8): 085014.
https://doi.org/10.1088/0960-1317/22/8/085014
54. Lee H, Ahn S, Bonassar LJ, et al. 2013, Cell (MC3T3‐E1)‐Printed Poly (ϵ‐caprolactone)/Alginate Hybrid Scaffolds for Tissue Regeneration, Macromolecular Rapid Communications, vol.34(2): 142-149.
https://doi.org/10.1002/marc.201200524
55. Park JY, Shim JH, Choi SA, et al. 2015, 3D printing technology to control BMP-2 and VEGF delivery spatially and temporally to promote large-volume bone regeneration, Journal of Materials Chemistry B, vol.3(27): 5415-5425. https://doi.org/10.1039/C5TB00637F
56. Yeo M, Lee H, Kim GH, 2016, Combining a micro/nano-hierarchical scaffold with cell printing of myoblasts induces cell alignment and differentiation favorable to skeletal muscle tissue regeneration, Biofabrication, vol.8(3): 035021.
https://doi.org/10.1088/1758-5090/8/3/035021
57. Mota C, Puppi D, Chiellini F, et al. 2015, Additive manufacturing techniques for the production of tissue engineering constructs, Journal of Tissue Engineering and Regenerative Medicine, vol.9(3): 174-190.
https://doi.org/10.1002/term.1635
58. Lutolf MP, Gilbert PM, Blau HM, 2009, Designing materials to direct stem-cell fate, Nature, vol.462(7272): 433-441. https://doi.org/10.1038/nature08602
59. Chung JH, Naficy S, Yue Z, et al. 2013, Bio-ink properties and printability for extrusion printing living cells, Biomaterials Science, vol.1(7): 763-773.
https://doi.org/10.1039/c3bm00012e
60. Chang CC, Boland ED, Williams SK, et al. 2011, Direct-write bioprinting three-dimensional biohybrid systems for future regenerative therapies, Journal of Biomedical Materials Research Part B: Applied Biomaterials, vol.98B(1): 160-170. https://doi.org/10.1002/jbm.b.31831
61. Khalil S, Sun W, 2009, Bioprinting Endothelial Cells With Alginate for 3D Tissue Constructs, Journal of Biomechanical Engineering, vol.131(11): 111002-111007. https://doi.org/10.1115/1.3128729
62. Cui XF, Breitenkamp K, Finn MG, et al. 2012, Direct Human Cartilage Repair Using Three-Dimensional Bioprinting Technology, Tissue Engineering Part A, vol.18(11-12): 1304-1312.
https://doi.org/10.1089/ten.tea.2011.0543
63. Nair K, Gandhi M, Khalil S, et al. 2009, Characterization of cell viability during bioprinting processes, Biotechnology Journal, vol.4(8): 1168-1177.
https://doi.org/10.1002/biot.200900004
64. Xu T, Gregory CA, Molnar P, et al. 2006, Viability and electrophysiology of neural cell structures generated by the inkjet printing method, Biomaterials, vol.27(19): 3580-3588. https://doi.org/10.1016/j.biomaterials.2006.01.048
65. Phillippi JA, Miller E, Weiss L, et al. 2008, Microenvironments Engineered by Inkjet Bioprinting Spatially Direct Adult Stem Cells Toward Muscle‐and Bone‐Like Subpopulations, Stem Cells, vol.26(1): 127-134.
https://doi.org/10.1634/stemcells.2007-0520
66. Cohen DL, Lo W, Tsavaris A, et al. 2010, Increased Mixing Improves Hydrogel Homogeneity and Quality of Three-Dimensional Printed Constructs, Tissue Engineering Part C: Methods, vol.17(2): 239-248.
https://doi.org/10.1089/ten.tec.2010.0093
67. Andersen T, Auk-Emblem P, Dornish M, 2015, 3D cell culture in alginate hydrogels, Microarrays, vol.4(2): 133-161. https://doi.org/10.3390/microarrays4020133
68. Gaebel R, Ma N, Liu J, et al. 2011, Patterning human stem cells and endothelial cells with laser printing for cardiac regeneration, Biomaterials, vol.32(35): 9218-9230. https://doi.org/10.1016/j.biomaterials.2011.08.071
69. Wu P, Ringeisen B, 2010, Development of human umbilical vein endothelial cell (HUVEC) and human umbilical vein smooth muscle cell (HUVSMC) branch/stem structures on hydrogel layers via biological laser printing (BioLP), Biofabrication, vol.2(1): 014111.
https://doi.org/10.1088/1758-5082/2/1/014111
70. Ker ED, Nain AS, Weiss LE, et al. 2011, Bioprinting of growth factors onto aligned sub-micron fibrous scaffolds for simultaneous control of cell differentiation and alignment, Biomaterials, vol.32(32): 8097-8107.
https://doi.org/10.1016/j.biomaterials.2011.07.025
71. Ilkhanizadeh S, Teixeira AI, Hermanson O, 2007, Inkjet printing of macromolecules on hydrogels to steer neural stem cell differentiation, Biomaterials, vol.28(27): 3936-3943. https://doi.org/10.1016/j.biomaterials.2007.05.018
72. Lee W, Debasitis JC, Lee VK, et al. 2009, Multi-layered culture of human skin fibroblasts and keratinocytes through three-dimensional freeform fabrication, Biomaterials, vol.30(8): 1587-1595.
https://doi.org/10.1016/j.biomaterials.2008.12.009
73. Duan B, Kapetanovic E, Hockaday LA, et al. 2014, Three-dimensional printed trileaflet valve conduits using biological hydrogels and human valve interstitial cells, Acta Biomaterialia, vol.10(5): 1836-1846.
https://doi.org/10.1016/j.actbio.2013.12.005
74. Duan B, Hockaday LA, Kang KH, et al. 2013, 3D bioprinting of heterogeneous aortic valve conduits with alginate/gelatin hydrogels, Journal of Biomedical Materials Research Part A, vol.101(5): 1255-1264.
https://doi.org/10.1002/jbm.a.34420
75. Gaetani R, Doevendans PA, Metz CH, et al. 2012, Cardiac tissue engineering using tissue printing technology and human cardiac progenitor cells, Biomaterials, vol.33(6): 1782-1790.
https://doi.org/10.1016/j.biomaterials.2011.11.003
76. Lee H, Kim G, 2014, Enhanced cellular activities of polycaprolactone/alginate-based cell-laden hierarchical scaffolds for hard tissue engineering applications, Journal of Colloid and Interface Science, vol.430: 315-325.
https://doi.org/10.1016/j.jcis.2014.05.065
77. Fedorovich NE, De Wijn JR, Verbout AJ, et al. 2008, Three-dimensional fiber deposition of cell-laden, viable, patterned constructs for bone tissue printing, Tissue Engineering Part A, vol.14(1): 127-133.
https://doi.org/10.1089/ten.a.2007.0158
78. Cui X, Boland T, 2009, Human microvasculature fabrication using thermal inkjet printing technology, Biomaterials, vol.30(31): 6221-6227.
https://doi.org/10.1016/j.biomaterials.2009.07.056
79. Norotte C, Marga FS, Niklason LE, et al. 2009, Scaffold-free vascular tissue engineering using bioprinting, Biomaterials, vol.30(30): 5910-5917.
https://doi.org/10.1016/j.biomaterials.2009.06.034
80. Marga F, Jakab K, Khatiwala C, et al. 2012, Toward engineering functional organ modules by additive manufacturing, Biofabrication, vol.4(2): 022001.
https://doi.org/10.1088/1758-5082/4/2/022001
81. Jakab K, Norotte C, Damon B, et al. 2008, Tissue engineering by self-assembly of cells printed into topologically defined structures, Tissue Engineering Part A, vol.14(3): 413-421. https://doi.org/10.1089/tea.2007.0173
82. Lee HJ, Kim YB, Ahn SH, et al. 2015, A New Approach for Fabricating Collagen/ECM‐Based Bioinks Using Preosteoblasts and Human Adipose Stem Cells, Advanced Healthcare Materials, vol.4(9): 1359-1368.
https://doi.org/10.1002/adhm.201500193