Utilising inkjet printed paraffin wax for cell patterning applications
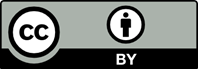
We describe a method to prepare patterned environments for eukaryotic cells by inkjet printing paraffin wax onto a substrate. This technique bypasses the requirement to create a master mould, typically required with the use of polydimethylsiloxane techniques and the printed structure could be immediately used to guide cell proliferation. In a space of 2–3 hours, the desired pattern could be created with computer assisted design, printed and have cells seeded onto the scaffold, which could reduce the cycle time of prototyping micropattern designs. Human dermal fibroblasts and RN22 Schwann cells were seen to proliferate within the fabricated patterns and survive for more than 7 days. Additionally, the wax constructs could be readily removed from the substrate at any stage after cell seeding with the cells continuing to proliferate. Thus, we report on a simple but novel approach for the controlled physical positioning of live cells by wax inkjet printing.
1. Théry M, 2010, Micropatterning as a tool to decipher cell morphogenesis and functions. Journal of Cell Science, vol.123: 4201–4213. http://dx.doi.org/10.1242/jcs.075150
2. Kumar S and LeDuc P R, 2009, Dissecting the molecular basis of the mechanics of living cells. Experimental Mechanics, vol.49(1): 11–23. http://dx.doi.org/10.1007/s11340-007-9063-7
3. Chen C S, Mrksich M, Huang S, et al., 1997, Geometric control of cell life and death. Science, vol.276: 1425–1428.
http://dx.doi.org/10.1126/science.276.5317.1425
4. Dike L E, Chen C S, Mrksich M, et al., 1999, Geometric control of switching between growth, apoptosis, and differentiation during angiogenesis using micropatterned substrates. In Vitro Cellular & Developmental Biology-Animal, vol.35(8): 441–448. http://dx.doi.org/10.1007/s11626-999-0050-4
5. McBeath R, Pirone D M, Nelson C M, et al., 2004, Cell shape, cytoskeletal tension, and RhoA regulate stem cell lineage commitment. Developmental Cell, vol.6(4): 483–495. http://dx.doi.org/10.1016/S1534-5807(04)00075-9
6. Banerjee S, Sknepnek R and Marchetti M C, 2014, Optimal shapes and stresses of adherent cells on patterned substrates. Soft Matter, vol.10(14): 2424–2430. http://dx.doi.org/10.1039/c3sm52647j
7. Geiger B, Spatz J P and Bershadsky A D, 2009, Environmental sensing through focal adhesions. Nature Reviews Molecular Cell Biology, vol.10: 21–33. http://dx.doi.org/10.1038/nrm2593
8. Duclos G, Garcia S, Yevick H G, et al., 2014, Perfect nematic order in confined monolayers of spindle-shaped cells. Soft Matter, vol.10(14): 2346–2353. http://dx.doi.org/10.1039/c3sm52323c
9. Alvarado J, Mulder B M and Koenderink G H, 2014, Alignment of nematic and bundled semiflexible polymers in cell-sized confinement. Soft Matter, vol.10(14): 2354–2364. http://dx.doi.org/10.1039/c3sm52421c
10. Tomba C, Braïni C, Wu B, et al., 2014, Tuning the adhesive geometry of neurons: length and polarity control. Soft Matter, vol.10(14): 2381–2387. http://dx.doi.org/10.1039/c3sm52342j
11. Röttgermann P J F, Alberola A P and Rädler J O, 2014, Cellular self-organization on micro-structured surfaces. Soft Matter, vol.10(14): 2397–2404. http://dx.doi.org/10.1039/c3sm52419a
12. Hampe N, Jonas T, Wolters B, et al., 2014, Defined 2-D microtissues on soft elastomeric silicone rubber using lift-off epoxy-membranes for biomechanical analyses. Soft Matter, vol.10(14): 2431–2443. http://dx.doi.org/10.1039/c3sm53123f
13. Schwarz U S, Nelson C M and Silberzan P, 2014, Pro-teins, cells, and tissues in patterned environments. Soft Matter, vol.10(14): 2337–2340. http://dx.doi.org/10.1039/c4sm90028f
14. Fujii T, 2002, PDMS-based microfluidic devices for biomedical applications. Microelectronic Engineering, vol.61–62: 907–914. http://dx.doi.org/10.1016/S0167-9317(02)00494-X
15. Duffy D C, McDonald J C, Schueller O J A, et al., 1998, Rapid prototyping of microfluidic systems in poly(dimethylsiloxane). Analytical Chemistry, vol.70(23): 4974– 4984. http://dx.doi.org/10.1021/ac980656z
16. Liu J, Enzelberger M and Quake S, 2002, A nanoliter rotary device for polymerase chain reaction. Electrophoresis, vol.23: 1531–1536. http://dx.doi.org/10.1002/1522-2683(200205)23:10<1531::AID-ELPS1531>3.0.CO;2-D
17. Linder V, Wu H, Jiang X, et al., 2003, Rapid prototyping of 2D structures with feature sizes larger than 8 μm. Analytical Chemistry, vol.75(10): 2522–2527. http://dx.doi.org/10.1021/ac026441d
18. McDonald J C, Chabinyc M L, Metallo S J, et al., 2002, Prototyping of microfluidic devices in poly(dimethyl-siloxane) using solid-object printing. Analytical Chemi-stry, vol.74(7): 1537–1545. http://dx.doi.org/10.1021/ac010938q
19. Love J C, Wolfe D B, Jacobs H O, et al., 2001, Micro-scope projection photolithography for rapid prototyping of masters with micron-scale features for use in soft li-thography. Langmuir, vol.17(19): 6005–6012. http://dx.doi.org/10.1021/la010655t
20. Kwon K W, Choi J C, Suh K Y, et al., 2011, Multiscale fabrication of multiple proteins and topographical structures by combining capillary force lithography and microscope projection photolithography. Langmuir,
vol.27(7): 3238–3243. http://dx.doi.org/10.1021/la2000156
21. Müller A, Meyer J, Paumer T, et al., 2014, Cytoskeletal transition in patterned cells correlates with interfacial energy model. Soft Matter, vol.10(14): 2444–2452. http://dx.doi.org/10.1039/c3sm52424h
22. Sanjana N E and Fuller S B, 2004, A fast flexible ink-jet printing method for patterning dissociated neurons in culture. Journal of Neuroscience Methods, vol.136(2): 151–163. http://dx.doi.org/10.1016/j.jneumeth.2004.01.011
23. Lu Y, Shi W, Jiang L, et al., 2009, Rapid prototyping of paper-based microfluidics with wax for low-cost, portable bioassay. Electrophoresis, vol.30(9): 1497–1500. http://dx.doi.org/10.1002/elps.200800563
24. Carrilho E, Martinez A W and Whitesides G M, 2009, Understanding wax printing: A simple micropatterning process for paper-based microfluidics. Analytical Che-mistry, vol.81(16): 7091–7095. http://dx.doi.org/10.1021/ac901071p
25. Renault C, Koehne J, Ricco A J, et al., 2014, Three-dim-ensional wax patterning of paper fluidic devices. Langmuir, vol.30(23): 7030–7036. http://dx.doi.org/10.1021/la501212b
26. Yun Y H, Lee B K, Choi J S, et al., 2011, A glucose sensor fabricated by piezoelectric inkjet printing of conducting polymers and bienzymes. Analytical Science, vol.27(4): 375-379. http://dx.doi.org/10.2116/analsci.27.375
27. Setti L, Fraleoni-Morgera A, Ballarin B, et al., 2005, An amperometric glucose biosensor prototype fabricated by thermal inkjet printing. Biosensors and Bioelectronics, vol.20(10): 2019–2026. http://dx.doi.org/10.1016/j.bios.2004.09.022
28. Wang T, Cook C and Derby B, 2009, Fabrication of a glucose biosensor by piezoelectric inkjet printing: Proceedings of the Third International Conference on Sensor Technologies and Applications, 2009 (SENSORCOM-M’09), 82–85. http://dx.doi.org/10.1109/SENSORCOMM.2009.20
29. Bietsch A, Zhang J, Hegner M, et al., 2004, Rapid functionalization of cantilever array sensors by inkjet printing. Nanotechnology, vol.15(8): 873–880. http://dx.doi.org/10.1088/0957-4484/15/8/002
30. Selimović Š, Dokmeci M R and Khademhosseini A, 2013, Research highlights. Lab on a Chip, vol.13(3): 325–327. http://dx.doi.org/10.1039/c2lc90145e
31. Kaigala G V, Ho S, Penterman R, et al., 2007, Rapid prototyping of microfluidic devices with a wax printer. Lab on a Chip, vol.7(3): 384–387. http://dx.doi.org/10.1039/b617764f