Decellularized extracellular matrix for three-dimensional bioprinted in vitro disease modeling
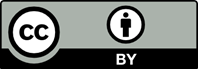
Precise in vitro models in tissue engineering have attracted the attention of researchers seeking to understand physiological consequences from native tissues as well as the mechanism of diseases in vitro. To construct delicate native tissue-like in vitro models, a proper combination of biomimetic materials and a biofabrication strategy is required. Conventional biomaterials, such as collagens, laminins, and synthetic polymers, have been widely adapted in tissue recapitulation; however, they lack tissue specificity in the context of biophysical properties and native-like extracellular matrix composition. The lack of tissue specificity accounts for the pathophysiological discrepancy between preclinical model and actual human patient. Thus, biomaterials should be improved for attaining physiological similarity between disease models and patients. Additionally, a biofabrication technique is essential for building mature cellular or tissue structures with a sophisticated bioassembly process. Among the biofabrication techniques, bioprinting stands as a promising approach for constructing three-dimensional (3D) cellular structures using specific cell types and biomaterials. Combining multifunctional bioinks and bioprinting is expected to enhance tissue specificity with regard to structural recapitulation. From this viewpoint, decellularized extracellular matrix (dECM) bioink has been increasingly used to achieve tissue specificity and manufacturability in 3D bioprinting. Progress in this domain requires the clarification of tissue-specific decellularization method and the development of a proper 3D bioprinting method, in conjunction with the improvement of the compatibility between dECM and bioprinting. In this review, we introduce the production methods and characteristics of dECM in the context of tissue specificity and examine state-of-the-art dECM-incorporated 3D-bioprinted in vitro models for disease investigation. We also recommend a strategy for improving dECM for use in therapeutic studies based on simulations of the pathophysiological microenvironment.
- Nikolic M, Sustersic T, Filipovic N. In vitro models and on-chip systems: biomaterial interaction studies with tissues generated using lung epithelial and liver metabolic cell lines. Front Bioeng Biotechnol. 2018;6:120. doi: 10.3389/fbioe.2018.00120
- Rothbauer M, Rosser JM, Zirath H, Ertl P. Tomorrow today: organ-on-a-chip advances towards clinically relevant pharmaceutical and medical in vitro models. Curr Opin Biotechnol. 2019;55:81-86. doi: 10.1016/j.copbio.2018.08.009
- Wang YI, Carmona C, Hickman JJ. Multiorgan microphysiological systems for drug development: strategies, advances, and challenges. Adv Healthc Mater. 2018;7(2):1701000. doi: 10.1002/adhm.201701000
- Wikswo JP. The relevance and potential roles of microphysiological systems in biology and medicine. Exp Biol Med. 2014;239(9):1061-1072. doi: 10.1177/1535370214542068
- Shyam R, Reddy LVK, Palaniappan A. Fabrication and characterization techniques of in vitro 3D tissue models. Int J Mol Sci. 2023;24(3):1912. doi: 10.3390/ijms24031912
- Bae M, Yi H-G, Jang J, Cho D-W. Microphysiological systems for neurodegenerative diseases in central nervous system. Micromachines. 2020;11(9):855. doi: 10.3390/mi11090855
- Brovold M, Almeida JI, Pla-Palacín I, et al. Naturally-derived biomaterials for tissue engineering applications. Novel Biomater Regen Med. 2018:421-449. doi: 10.1007/978-981-13-0947-2_23
- Joyce K, Fabra GT, Bozkurt Y, Pandit A. Bioactive potential of natural biomaterials: identification, retention and assessment of biological properties. Signal Transduct Target Ther. 2021;6(1):122. doi: 10.1038/s41392-021-00512-8
- Groll J, Boland T, Blunk T, et al. Biofabrication: reappraising the definition of an evolving field. Biofabrication. 2016;8(1):013001. doi: 10.1088/1758-5090/8/1/013001
- Moroni L, Burdick JA, Highley C, et al. Biofabrication strategies for 3D in vitro models and regenerative medicine. Nat Rev Mater. 2018;3(5):21-37. doi: 10.1038/s41578-018-0006-y
- Devillard CD, Marquette CA. Vascular tissue engineering: challenges and requirements for an ideal large scale blood vessel. Front Bioeng Biotechnol. 2021;9:721843. doi: 10.3389/fbioe.2021.721843
- Cesare G. Muscle tissue engineering. Nukavarapu SP, Freeman JW, Laurencin CT, eds. Regenerative Engineering of Musculoskeletal Tissues and Interfaces. Amsterdam, Netherlands: Elsevier; 2015:239-268. doi: 10.1016/C2014-0-02826-2
- Zhang X, Chen X, Hong H, Hu R, Liu J, Liu C. Decellularized extracellular matrix scaffolds: recent trends and emerging strategies in tissue engineering. Bioact Mater. 2022;10:15-31. doi: 10.1016/j.bioactmat.2021.09.014
- Kim BS, Kim H, Gao G, Jang J, Cho D-W. Decellularized extracellular matrix: a step towards the next generation source for bioink manufacturing. Biofabrication. 2017;9(3):034104. doi: 10.1088/1758-5090/aa7e98
- Kim BS, Das S, Jang J, Cho D-W. Decellularized extracellular matrix-based bioinks for engineering tissue-and organ-specific microenvironments. Chem Rev. 2020;120(19): 10608-10661. doi: 10.1021/acs.chemrev.9b00808
- Han W, Singh NK, Kim JJ, et al. Directed differential behaviors of multipotent adult stem cells from decellularized tissue/organ extracellular matrix bioinks. Biomaterials. 2019;224:119496. doi: 10.1016/j.biomaterials.2019.119496
- Hynes RO, Naba A. Overview of the matrisome—an inventory of extracellular matrix constituents and functions. Cold Spring Harb Perspect Biol. 2012;4(1):a004903. doi: 10.1101/cshperspect.a004903
- Uhlén M, Fagerberg L, Hallström BM, et al. Tissue-based map of the human proteome. Science. 2015;347(6220):1260419. doi: 10.1126/science.1260419
- Murphy SV, Atala A. 3D bioprinting of tissues and organs. Nat. Biotechnol. 2014;32(8):773-785. doi: 10.1038/nbt.2958
- Tan B, Gan S, Wang X, Liu W, Li X. Applications of 3D bioprinting in tissue engineering: advantages, deficiencies, improvements, and future perspectives. J Mater Chem B. 2021;9(27):5385-5413. doi: 10.1039/D1TB00172H
- Kačarević ŽP, Rider PM, Alkildani S, et al. An introduction to 3D bioprinting: possibilities, challenges and future aspects. Materials. 2018;11(11):2199. doi: 10.3390/ma11112199
- Zhang YS, Haghiashtiani G, Hübscher T, et al. 3D extrusion bioprinting. Nat Rev Methods Primers. 2021;1(1):75. doi: 10.1038/s43586-021-00073-8
- Li X, Liu B, Pei B, et al. Inkjet bioprinting of biomaterials. Chem Rev. 2020;120(19):10793-10833. doi: 10.1021/acs.chemrev.0c00008
- Guillotin B, Souquet A, Catros S, et al. Laser assisted bioprinting of engineered tissue with high cell density and microscale organization. Biomaterials. 2010;31(28): 7250-7256. doi: 10.1016/j.biomaterials.2010.05.055
- Ramesh S, Harrysson OL, Rao PK, et al. Extrusion bioprinting: recent progress, challenges, and future opportunities. Bioprinting. 2021;21:e00116. doi: 10.1016/j.bprint.2020.e00116
- Binder KW, Allen AJ, Yoo JJ, Atala A. Drop-on-demand inkjet bioprinting: a primer. Gene Ther Regul. 2011;6(01):33-49. doi: 10.1142/S1568558611000258
- Ventura RD. An overview of laser-assisted bioprinting (LAB) in tissue engineering applications. Med Lasers. 2021;10(2):76-81. doi: 10.25289/ML.2021.10.2.76
- Pati F, Jang J, Ha D-H, et al. Printing three-dimensional tissue analogues with decellularized extracellular matrix bioink. Nat Commun. 2014;5(1):3935. doi: 10.1038/ncomms4935
- Hubmacher D, Apte SS. The biology of the extracellular matrix: novel insights. Curr Opin Rheumatol. 2013;25(1):65. doi: 10.1097/BOR.0b013e32835b137b
- Huggins ML. The structure of fibrous proteins. Chem Rev. 1943;32(2):195-218. doi: 10.1021/cr60102a002
- Parry DA, Squire JM. Fibrous Proteins: Structures and Mechanisms. New York City, USA: Springer; 2017. doi: 10.1007/978-3-319-49674-0
- Linsenmayer T. Collagen. In: Hay ED, ed. Cell Biology of Extracellular Matrix. 2nd ed. New York, NY: Springer; 1991:7-44. doi: 10.1007/978-1-4615-3770-0
- Kristensen J, Karsdal M. Elastin. Karsdal MA, eds. Biochemistry of Collagens, Laminins, and Elastin: Structure, Function, and Biomarkers. Cambridge, Massachusetts, USA: Elsevier; 2016. doi: 10.1016/C2015-0-05547-2
- Rouse JG, Van Dyke ME. A review of keratin-based biomaterials for biomedical applications. Materials. 2010;3(2):999-1014. doi: 10.3390/ma3020999
- Kwiatkowska D, Kwiatkowska-Korczak J. Adhesive glycoproteins of the extracellular matrix. Postepy Hig Med Dosw. 1999;53(1):55-74.
- Bonnans C, Chou J, Werb Z. Remodelling the extracellular matrix in development and disease. Nat Rev Mol Cell Biol. 2014;15(12):786-801. doi: 10.1038/nrm3904
- Mendibil U, Ruiz-Hernandez R, Retegi-Carrion S, Garcia- Urquia N, Olalde-Graells B, Abarrategi A. Tissue-specific decellularization methods: rationale and strategies to achieve regenerative compounds. Int J Mol Sci. 2020;21(15):5447. doi: 10.3390/ijms21155447
- Bowers SL, Banerjee I, Baudino TA. The extracellular matrix: at the center of it all. J Mol Cell Cardiol. 2010;48(3): 474-482. doi: 10.1016/j.yjmcc.2009.08.024
- Das S, Gao G, Lee JY, Jang J, Cho D-W. Decellularized tissue matrix-based 3D tissue modeling. In: Cho D-W, ed. Biofabrication and 3D Tissue Modeling. Burlington House, Piccadilly, London: The Royal Society of Chemistry; 2019. doi: 10.1039/9781788012683-00148
- Brown BN, Badylak SF. Extracellular matrix as an inductive scaffold for functional tissue reconstruction. Transl Res. 2014;163(4):268-285. doi: 10.1016/j.trsl.2013.11.003
- Song JJ, Ott HC. Organ engineering based on decellularized matrix scaffolds. Trends Mol Med. 2011;17(8):424-432. doi: 10.1016/j.molmed.2011.03.005
- Sakai T, Larsen M, Yamada KM. Fibronectin requirement in branching morphogenesis. Nature. 2003;423(6942):876-881. doi: 10.1038/nature01712
- Hwang DG, Choi Y-m, Jang J. 3D bioprinting-based vascularized tissue models mimicking tissue-specific architecture and pathophysiology for in vitro studies. Front Bioeng Biotechnol. 2021;9:685507. doi: 10.3389/fbioe.2021.685507
- Barros CS, Franco SJ, Müller U. Extracellular matrix: functions in the nervous system. Cold Spring Harb Perspect Biol. 2011;3(1):a005108. doi: 10.1101/cshperspect.a005108
- Lau LW, Cua R, Keough MB, Haylock-Jacobs S, Yong VW. Pathophysiology of the brain extracellular matrix: a new target for remyelination. Nat Rev Neurosci. 2013;14(10):722-729. doi: 10.1038/nrn3550
- Choi BH. Role of the basement membrane in neurogenesis and repair of injury in the central nervous system. Microsc Res Tech. 1994;28(3):193-203. doi: 10.1002/jemt.1070280304
- Fawcett JW, Oohashi T, Pizzorusso T. The roles of perineuronal nets and the perinodal extracellular matrix in neuronal function. Nat Rev Neurosci. 2019;20(8):451-465. doi: 10.1038/s41583-019-0196-3
- Bekku Y, Saito M, Moser M, et al. Bral2 is indispensable for the proper localization of brevican and the structural integrity of the perineuronal net in the brainstem and cerebellum. J Comp Neurol. 2012;520(8):1721-1736. doi: 10.1002/cne.23009
- Ricks CB, Shin SS, Becker C, Grandhi R. Extracellular matrices, artificial neural scaffolds and the promise of neural regeneration. Neural Regen Res. 2014;9(17):1573. doi: 10.4103/1673-5374.141778
- Sood D, Tang-Schomer M, Pouli D, et al. 3D extracellular matrix microenvironment in bioengineered tissue models of primary pediatric and adult brain tumors. Nat Commun. 2019;10(1):4529. doi: 10.1038/s41467-019-12420-1
- Henke E, Nandigama R, Ergün S. Extracellular matrix in the tumor microenvironment and its impact on cancer therapy. Front Mol Biosci. 2020;6:160. doi: 10.3389/fmolb.2019.00160
- Silva AC, Pereira C, Fonseca ACR, Pinto-do-Ó P, Nascimento DS. Bearing my heart: the role of extracellular matrix on cardiac development, homeostasis, and injury response. Front Cell Dev Biol. 2021;8:621644. doi: 10.3389/fcell.2020.621644
- Rienks M, Papageorgiou A-P, Frangogiannis NG, Heymans S. Myocardial extracellular matrix: an ever-changing and diverse entity. Circ Res. 2014;114(5):872-888. doi: 10.1161/CIRCRESAHA.114.302533
- Lockhart M, Wirrig E, Phelps A, Wessels A. Extracellular matrix and heart development. Birth Defects Res, Part A. 2011;91(6):535-550. doi: 10.1002/bdra.20810
- Buijtendijk MF, Barnett P, van den Hoff MJ. Development of the human heart. Am J Med Genet, Part C. 2020;184(1):7-22. doi: 10.1002/ajmg.c.31778
- Park H-J, De Jesus Morales KJ, Bheri S, Kassouf BP, Davis ME. Bidirectional relationship between cardiac extracellular matrix and cardiac cells in ischemic heart disease. Stem Cell. 2021;39(12):1650-1659. doi: 10.1002/stem.3445
- Bedossa P, Paradis V. Liver extracellular matrix in health and disease. J Pathol. 2003;200(4):504-515. doi: 10.1002/path.1397
- Martinez-Hernandez A, Amenta PS. Morphology, localization and origin of the hepatic extracellular matrix. In: Zern MA, Reid L, eds. Extracellular Matrix: Chemistry, Biology, and Pathobiology with Emphasis on the Liver. New York, NY: Marcel Dekker; 1993:255-327. doi: 10.1007/BF01606580
- Rojkind M, Giambrone M-A, Biempica L. Collagen types in normal and cirrhotic liver. Gastroenterology. 1979;76(4): 710-719. doi: 10.1016/S0016-5085(79)80170-5
- Martinez-Hernandez A. The hepatic extracellular matrix. I. Electron immunohistochemical studies in normal rat liver. Lab Invest. 1984;51(1):57-74. https://europepmc.org/article/med/6376944
- Schuppan D. Structure of the extracellular matrix in normal and fibrotic liver: collagens and glycoproteins. Semin Liver Dis. 1990;10(1):1-10. doi: 10.1055/s-2008-1040452
- Wells RG. The role of matrix stiffness in regulating cell behavior. Hepatology. 2008;47(4):1394-1400. doi: 10.1002/hep.22193
- Gressner A. Hepatic fibrogenesis: the puzzle of interacting cells, fibrogenic cytokines, regulatory loops, and extracellular matrix molecules. Z Gastroenterol. 1992;30:5-16.
- McGuire RF, Bissell DM, Boyles J, Joseph Roll F. Role of extracellular matrix in regulating fenestrations of sinusoidal endothelial cells isolated from normal rat liver. Hepatology. 1992;15(6):989-997. doi: 10.1002/hep.1840150603
- Tan Z, Sun H, Xue T, et al. Liver fibrosis: therapeutic targets and advances in drug therapy. Front Cell Dev Biol. 2021;9:730176. doi: 10.3389/fcell.2021.730176
- Hussey GS, Dziki JL, Badylak SF. Extracellular matrix-based materials for regenerative medicine. Nat Rev Mater. 2018;3(7):159-173. doi: 10.1038/s41578-018-0023-x
- de Hilster RHJ, Sharma PK, Jonker MR, et al. Human lung extracellular matrix hydrogels resemble the stiffness and viscoelasticity of native lung tissue. Am J Physiol. 2020;318(4):L698-L704. doi: 10.1152/ajplung.00451.2019
- Silver FH, Birk DE. Molecular structure of collagen in solution: comparison of types I, II, III and V. Int J Biol Macromol. 1984;6(3):125-132. doi: 10.1016/0141-8130(84)90052-7
- Lever R, Page C. Glycosaminoglycans, airways inflammation and bronchial hyperresponsiveness. Pulmon Pharmacol Therap. 2001;14(3):249-254. doi: 10.1006/pupt.2001.0296
- Burgstaller G, Oehrle B, Gerckens M, White ES, Schiller HB, Eickelberg O. The instructive extracellular matrix of the lung: basic composition and alterations in chronic lung disease. Eur Respir J. 2017;50(1):1601805. doi: 10.1183/13993003.01805-2016
- Shifren A, Mecham RP. The stumbling block in lung repair of emphysema: elastic fiber assembly. Proc Am Thorac Soc. 2006;3(5):428-433. doi: 10.1513/pats.200601-009AW
- Kranenburg AR, Willems-Widyastuti A, Mooi WJ, et al. Enhanced bronchial expression of extracellular matrix proteins in chronic obstructive pulmonary disease. Am J Clin Pathol. 2006;126(5):725-735. doi: 10.1309/JC477FAEL1YKV54W
- Salazar LM, Herrera AM. Fibrotic response of tissue remodeling in COPD. Lung. 2011;189:101-109. doi: 10.1007/s00408-011-9279-2
- Chakir J, Shannon J, Molet S, et al. Airway remodeling-associated mediators in moderate to severe asthma: effect of steroids on TGF-β, IL-11, IL-17, and type I and type III collagen expression. J Allergy Clin Immunol. 2003;111(6):1293-1298. doi: 10.1067/mai.2003.1557
- Huang D, Gibeley SB, Xu C, et al. Engineering liver microtissues for disease modeling and regenerative medicine. Adv Funct Mater. 2020;30(44):1909553. doi: 10.1002/adfm.201909553
- Parmaksiz M, Dogan A, Odabas S, Elçin AE, Elçin YM. Clinical applications of decellularized extracellular matrices for tissue engineering and regenerative medicine. Biomed Mater. 2016;11(2):022003. doi: 10.1088/1748-6041/11/2/022003
- Mouw JK, Ou G, Weaver VM. Extracellular matrix assembly: a multiscale deconstruction. Nat Rev Mol Cell Biol. 2014;15(12):771-785. doi: 10.1038/nrm3902
- Choudhury D, Tun HW, Wang T, Naing MW. Organ-derived decellularized extracellular matrix: a game changer for bioink manufacturing? Trends Biotechnol. 2018;36(8):787-805. doi: 10.1016/j.tibtech.2018.03.003
- Park W, Gao G, Cho D-W. Tissue-specific decellularized extracellular matrix bioinks for musculoskeletal tissue regeneration and modeling using 3D bioprinting technology. Int J Mol Sci. 2021;22(15):7837. doi: 10.3390/ijms22157837
- Heath DE. A review of decellularized extracellular matrix biomaterials for regenerative engineering applications. Regen Eng Transl Med. 2019;5:155-166. doi: 10.1007/s40883-018-0080-0
- Kim JJ, Park JY, Nguyen VVT, et al. Pathophysiological reconstruction of a tissue‐specific multiple‐organ on‐a‐chip for Type 2 diabetes emulation using 3D cell printing. Adv Funct Mater. 2023;33(22):2213649. doi: 10.1002/adfm.202213649
- Yang X, Ma Y, Wang X, et al. A 3D‐bioprinted functional module based on decellularized extracellular matrix bioink for periodontal regeneration. Adv Sci. 2022;10(5):2205041. doi: 10.1002/advs.202205041
- Kim H, Jang J-H, Han W, et al. Extracellular matrix-based sticky sealants for scar-free corneal tissue reconstruction. Biomaterials. 2023;292:121941. doi: 10.1016/j.biomaterials.2022.121941
- Chae S, Yong U, Park W, et al. 3D cell-printing of gradient multi-tissue interfaces for rotator cuff regeneration. Bioact Mater. 2023;19:611-625. doi: 10.1016/j.bioactmat.2022.05.004
- Cho A-N, Jin Y, An Y, et al. Microfluidic device with brain extracellular matrix promotes structural and functional maturation of human brain organoids. Nat Commun. 2021;12(1):4730. doi: 10.1038/s41467-021-24775-5
- Giobbe GG, Crowley C, Luni C, et al. Extracellular matrix hydrogel derived from decellularized tissues enables endodermal organoid culture. Nat Commun. 2019;10(1):5658. doi: 10.1038/s41467-019-13605-4
- Mandrycky C, Wang Z, Kim K, Kim D-H. 3D bioprinting for engineering complex tissues. Biotechnol Adv. 2016;34(4): 422-434. doi: 10.1016/j.biotechadv.2015.12.011
- Abaci A, Guvendiren M. Designing decellularized extracellular matrix‐based bioinks for 3D bioprinting. Adv Healthc Mater. 2020;9(24):2000734. doi: 10.1002/adhm.202000734
- De Santis MM, Alsafadi HN, Tas S, et al. Extracellular‐matrix‐reinforced bioinks for 3D bioprinting human tissue. Adv Mater. 2021;33(3):2005476. doi: 10.1002/adma.202005476
- de Paula AGP, de Lima JD, Bastos TSB, et al. Decellularized extracellular matrix: the role of this complex biomaterial in regeneration. ACS Omega. 2023;8(25):22256-22267. doi: 10.1021/acsomega.2c06216
- Wu L-C, Kuo Y-J, Sun F-W, et al. Optimized decellularization protocol including α-Gal epitope reduction for fabrication of an acellular porcine annulus fibrosus scaffold. Cell Tissue Bank. 2017;18:383-396. doi: 10.1007/s10561-017-9619-4
- Stahl EC, Bonvillain RW, Skillen CD, et al. Evaluation of the host immune response to decellularized lung scaffolds derived from α-Gal knockout pigs in a non-human primate model. Biomaterials. 2018;187:93-104. doi: 10.1016/j.biomaterials.2018.09.038
- Warren CJ, Sawyer SL. Identifying animal viruses in humans. Science. 2023;379(6636):982-983. doi: 10.1126/science.ade6985
- Denner J. Porcine endogenous retroviruses and xenotransplantation, 2021. Viruses. 2021;13(11):2156. doi: 10.3390/v13112156
- Naso F, Gandaglia A. Can heart valve decellularization be standardized? A review of the parameters used for the quality control of decellularization processes. Front Bioeng Biotechnol. 2022;10:830899. doi: 10.3389/fbioe.2022.830899
- Rocha DN, Ferraz-Nogueira JP, Barrias CC, Relvas JB, Pêgo AP. Extracellular environment contribution to astrogliosis— lessons learned from a tissue engineered 3D model of the glial scar. Front Cell Neurosci. 2015;9:377. doi: 10.3389/fncel.2015.00377
- Galarza S, Crosby AJ, Pak C, Peyton SR. Control of astrocyte quiescence and activation in a synthetic brain hydrogel. Adv Healthc Mater. 2020;9(4):1901419. doi: 10.1002/adhm.201901419
- Kasravi M, Ahmadi A, Babajani A, et al. Immunogenicity of decellularized extracellular matrix scaffolds: a bottleneck in tissue engineering and regenerative medicine. Biomater Res. 2023;27(1):1-24. doi: 10.1186/s40824-023-00348-z
- Chakraborty J, Roy S, Ghosh S. Regulation of decellularized matrix mediated immune response. Biomater Sci. 2020;8(5):1194-1215. doi: 10.1039/C9BM01780A
- Jiang Y, Li R, Han C, et al. Extracellular matrix grafts: from preparation to application. Int J Mol Med. 2021;47(2):463- 474. doi: 10.3892/ijmm.2020.4818
- Gilpin A, Yang Y. Decellularization strategies for regenerative medicine: from processing techniques to applications. Biomed Res Int. 2017;2017:9831534. doi: 10.1155/2017/9831534
- Basara G, Ozcebe SG, Ellis BW, Zorlutuna P. Tunable human myocardium derived decellularized extracellular matrix for 3D bioprinting and cardiac tissue engineering. Gels. 2021;7(2):70. doi: 10.3390/gels7020070
- Jang J, Kim TG, Kim BS, Kim S-W, Kwon S-M, Cho D-W. Tailoring mechanical properties of decellularized extracellular matrix bioink by vitamin B2-induced photo-crosslinking. Acta Biomater. 2016;33:88-95. doi: 10.1016/j.actbio.2016.01.013
- He W, Wang H, Zhang X, et al. Construction of a decellularized spinal cord matrix/GelMA composite scaffold and its effects on neuronal differentiation of neural stem cells. J Biomater Sci. 2022;33(16):2124-2144. doi: 10.1080/09205063.2022.2102275
- Shin YJ, Shafranek RT, Tsui JH, Walcott J, Nelson A, Kim D-H. 3D bioprinting of mechanically tuned bioinks derived from cardiac decellularized extracellular matrix. Acta Biomater. 2021;119:75-88. doi: 10.1016/j.actbio.2020.11.006
- Gao G, Park W, Kim BS, et al. Construction of a novel in vitro atherosclerotic model from geometry‐tunable artery equivalents engineered via in‐bath coaxial cell printing. Adv Funct Mater. 2021;31(10):2008878. doi: 10.1002/adfm.202008878
- Rabbani M, Zakian N, Alimoradi N. Contribution of physical methods in decellularization of animal tissues. J Med Signals Sens. 2021;11(1):1-11. https://doi.org/10.4103/jmss.JMSS_2_20
- DeQuach JA, Yuan SH, Goldstein LS, Christman KL. Decellularized porcine brain matrix for cell culture and tissue engineering scaffolds. Tiss Eng Part A. 2011; 17(21-22):2583-2592. doi: 10.1089/ten.tea.2010.0724
- Gregory E, Baek IH, Ala-Kokko N, et al. Peripheral nerve decellularization for in vitro extracellular matrix hydrogel use: a comparative study. ACS Biomater Sci Eng. 2022;8(6):2574-2588. doi: 10.1021/acsbiomaterials.2c00034
- Kim J, Park JY, Kong JS, Lee H, Won JY, Cho D-W. Development of 3D printed Bruch’s membrane-mimetic substance for the maturation of retinal pigment epithelial cells. Int J Mol Sci. 2021;22(3):1095. doi: 10.3390/ijms22031095
- Kundu J, Michaelson A, Talbot K, Baranov P, Young MJ, Carrier RL. Decellularized retinal matrix: natural platforms for human retinal progenitor cell culture. Acta Biomater. 2016;31:61-70. doi: 10.1016/j.actbio.2015.11.028
- Lee J, Hong J, Kim W, Kim GH. Bone-derived dECM/ alginate bioink for fabricating a 3D cell-laden mesh structure for bone tissue engineering. Carbohydr Polym. 2020;250:116914. doi: 10.1016/j.carbpol.2020.116914
- Sun Y, Yan L, Chen S, Pei M. Functionality of decellularized matrix in cartilage regeneration: a comparison of tissue versus cell sources. Acta Biomater. 2018;74:56-73. doi: 10.1016/j.actbio.2018.04.048
- Gilbert TW, Sellaro TL, Badylak SF. Decellularization of tissues and organs. Biomaterials. 2006;27(19):3675-3683. doi: 10.1016/j.biomaterials.2006.02.014
- Neishabouri A, Soltani Khaboushan A, Daghigh F, Kajbafzadeh A-M, Zolbin MM. Decellularization in tissue engineering and regenerative medicine: evaluation, modification, and application methods. Front Bioeng Biotechnol. 2022;10:805299. doi: 10.3389/fbioe.2022.805299
- Ketchedjian A, Jones AL, Krueger P, et al. Recellularization of decellularized allograft scaffolds in ovine great vessel reconstructions. Ann Thorac Surg. 2005;79(3):888-896. doi: 10.1016/j.athoracsur.2004.09.033
- White LJ, Taylor AJ, Faulk DM, et al. The impact of detergents on the tissue decellularization process: a ToF-SIMS study. Acta Biomater. 2017;50:207-219. doi: 10.1016/j.actbio.2016.12.033
- Pellegata AF, Asnaghi M, Stefani I, et al. Detergent-enzymatic decellularization of swine blood vessels: insight on mechanical properties for vascular tissue engineering. Biomed Res Int. 2013;2013:918753. doi: 10.1155/2013/918753
- Fernández-Pérez J, Ahearne M. The impact of decellularization methods on extracellular matrix derived hydrogels. Sci Rep. 2019;9(1):14933. doi: 10.1038/s41598-019-49575-2
- Yang B, Zhang Y, Zhou L, et al. Development of a porcine bladder acellular matrix with well-preserved extracellular bioactive factors for tissue engineering. Tissue Eng Part C. 2010;16(5):1201-1211. doi: 10.1089/ten.tec.2009.0311
- Poornejad N, Schaumann LB, Buckmiller EM, et al. The impact of decellularization agents on renal tissue extracellular matrix. J Biomater Appl. 2016;31(4): 521-533. doi: 10.1177/0885328216656099
- Choi JS, Kim BS, Kim JY, et al. Decellularized extracellular matrix derived from human adipose tissue as a potential scaffold for allograft tissue engineering. J Biomed Mater Res Part A. 2011;97(3):292-299. doi: 10.1002/jbm.a.33056
- Narciso M, Otero J, Navajas D, Farré R, Almendros I, Gavara N. Image-based method to quantify decellularization of tissue sections. Int J Mol Sci. 2021;22(16):8399. doi: 10.3390/ijms22168399
- Dimou Z, Michalopoulos E, Katsimpoulas M, et al. Evaluation of a decellularization protocol for the development of a decellularized tracheal scaffold. Anticancer Res. 2019;39(1):145-150. doi: 10.21873/anticanres.13090
- Jin Y, Lee JS, Kim J, et al. Three-dimensional brain-like microenvironments facilitate the direct reprogramming of fibroblasts into therapeutic neurons. Nat Biomed Eng. 2018;2(7):522-539. doi: 10.1038/s41551-018-0260-8
- Kim BS, Kwon YW, Kong J-S, et al. 3D cell printing of in vitro stabilized skin model and in vivo pre-vascularized skin patch using tissue-specific extracellular matrix bioink: a step towards advanced skin tissue engineering. Biomaterials. 2018;168:38-53. doi: 10.1016/j.biomaterials.2018.03.040
- Byron A, Humphries JD, Humphries MJ. Defining the extracellular matrix using proteomics. Int J Exp Pathol. 2013;94(2):75-92. doi: 10.1111/iep.12011
- Mi H, Muruganujan A, Thomas PD. PANTHER in 2013: modeling the evolution of gene function, and other gene attributes, in the context of phylogenetic trees. Nucleic Acids Res. 2012;41(D1):D377-D386. doi: 10.1093/nar/gks1118
- Shao X, Taha IN, Clauser KR, Gao YT, Naba A. MatrisomeDB: the ECM-protein knowledge database. Nucleic Acids Res. 2020;48(D1):D1136-D1144. doi: 10.1093/nar/gkz849
- Chen Y-W, Lin Y-H, Lin T-L, Lee K-XA, Yu M-H, Shie M-Y.3D-biofabricated chondrocyte-laden decellularized extracellular matrix-contained gelatin methacrylate auxetic scaffolds under cyclic tensile stimulation for cartilage regeneration. Biofabrication. 2023;15(4):045007. doi: 10.1088/1758-5090/ace5e1
- Simsa R, Rothenbücher T, Gürbüz H, et al. Brain organoid formation on decellularized porcine brain ECM hydrogels. PLoS One. 2021;16(1):e0245685. doi: 10.1371/journal.pone.0245685
- Ijima H, Nakamura S, Bual R, Shirakigawa N, Tanoue S. Physical properties of the extracellular matrix of decellularized porcine liver. Gels. 2018;4(2):39. doi: 10.3390/gels4020039
- Goh S-K, Bertera S, Olsen P, et al. Perfusion-decellularized pancreas as a natural 3D scaffold for pancreatic tissue and whole organ engineering. Biomaterials. 2013;34(28):6760-6772. doi: 10.1016/j.biomaterials.2013.05.066
- Mironov V, Visconti RP, Kasyanov V, Forgacs G, Drake CJ, Markwald RR. Organ printing: tissue spheroids as building blocks. Biomaterials. 2009;30(12):2164-2174. doi: 10.1016/j.biomaterials.2008.12.084
- Chen X, Zhang YS, Zhang X, Liu C. Organ-on-a-chip platforms for accelerating the evaluation of nanomedicine. Bioact Mater. 2021;6(4):1012-1027. doi: 10.1016/j.bioactmat.2020.09.022
- Brodal P. The Central Nervous System: Structure and Function. Oxford, England: Oxford University Press; 2004. https://global.oup.com/academic/product/the-central-nervous-system-9780190228958?cc=kr&lang=en&
- Lancaster MA, Renner M, Martin C-A, et al. Cerebral organoids model human brain development and microcephaly. Nature. 2013;501(7467):373-379. doi: 10.1038/nature12517
- Saha K, Keung AJ, Irwin EF, et al. Substrate modulus directs neural stem cell behavior. Biophys J. 2008;95(9):4426-4438. doi: 10.1529/biophysj.108.132217
- Yi B, Xu Q, Liu W. An overview of substrate stiffness guided cellular response and its applications in tissue regeneration. Bioact Mater. 2022;15:82-102. doi: 10.1016/j.bioactmat.2021.12.005
- Portenoy RK. Cancer pain: pathophysiology and syndromes. Lancet. 1992;339(8800):1026-1031. doi: 10.1016/0140-6736(92)90545-e
- Yi H-G, Jeong YH, Kim Y, et al. A bioprinted human-glioblastoma-on-a-chip for the identification of patient-specific responses to chemoradiotherapy. Nat Biomed Eng. 2019;3(7):509-519. doi: 10.1038/s41551-019-0363-x
- Park W, Bae M, Hwang M, Jang J, Cho D-W, Yi H-G. 3D cell-printed hypoxic cancer-on-a-chip for recapitulating pathologic progression of solid cancer. JoVE. 2021;(167):e61945. doi: 10.3791/61945
- Slanzi A, Iannoto G, Rossi B, Zenaro E, Constantin G. In vitro models of neurodegenerative diseases. Front Cell Dev Biol. 2020;8:328. doi: 10.3389/fcell.2020.00328
- Cetin S, Knez D, Gobec S, Kos J, Pišlar A. Cell models for Alzheimer’s and Parkinson’s disease: at the interface of biology and drug discovery. Biomed Pharmacother. 2022;149:112924. doi: 10.1016/j.biopha.2022.112924
- Osaki T, Shin Y, Sivathanu V, Campisi M, Kamm RD. In vitro microfluidic models for neurodegenerative disorders. Adv Healthc Mater. 2018;7(2):1700489. doi: 10.1002/adhm.201700489
- Kong JS, Huang X, Choi YJ, et al. Promoting long‐term cultivation of motor neurons for 3D neuromuscular junction formation of 3D in vitro using central‐nervous‐tissue‐derived bioink. Adv Healthc Mater. 2021;10(18):2100581. doi: 10.1002/adhm.202100581
- Kim W, Kim G. Bioprinting 3D muscle tissue supplemented with endothelial-spheroids for neuromuscular junction model. Appl Phys Rev. 2023;10(3):031410. doi: 10.1063/5.0152924
- Lee SJ, Esworthy T, Stake S, et al. Advances in 3D bioprinting for neural tissue engineering. Adv Biosyst. 2018;2(4):1700213. doi: 10.1002/adbi.201700213
- Daneman R, Prat A. The blood–brain barrier. Cold Spring Harb Perspect Biol. 2015;7(1):a020412. doi: 10.1101/cshperspect.a020412
- Potjewyd G, Moxon S, Wang T, Domingos M, Hooper NM. Tissue engineering 3D neurovascular units: a biomaterials and bioprinting perspective. Trends Biotechnol. 2018;36(4):457-472. doi: 10.1016/j.tibtech.2018.01.003
- Mossink B, Verboven AH, van Hugte EJ, et al. Human neuronal networks on micro-electrode arrays are a highly robust tool to study disease-specific genotype-phenotype correlations in vitro. Stem Cell Rep. 2021;16(9):2182-2196. doi: 10.1016/j.stemcr.2021.07.001
- Nabel EG. Cardiovascular disease. N Engl J Med. 2003; 349(1):60-72. doi: 10.1056/NEJMra035098
- Wilkins E, Wilson L, Wickramasinghe K, et al. European Cardiovascular Disease Statistics 2017. Brussels, Belgium: European Heart Network; 2017. https://researchportal.bath.ac.uk/en/publications/ european-cardiovascular-disease-statistics-2017
- Zhang WJ, Liu W, Cui L, Cao Y. Tissue engineering of blood vessel. J Cell Mol Med. 2007;11(5):945-957. doi: 10.1111/j.1582-4934.2007.00099.x
- Abramson DI. Blood Vessels and Lymphatics. Cambridge, Massachusetts, USA: Elsevier; 2013. doi: 10.1016/C2013-0-12391-4
- Bogseth A, Ramirez A, Vaughan E, Maisel K. In vitro models of blood and lymphatic vessels—connecting tissues and immunity. Adv Biol. 2023;7(5):2200041. doi: 10.1002/adbi.202200041
- Abdelsayed G, Ali D, Malone A, et al. 2D and 3D in-vitro models for mimicking cardiac physiology. Appl Eng Sci. 2022;12:100115. doi: 10.1016/j.apples.2022.100115
- Ferri N, Siegl P, Corsini A, Herrmann J, Lerman A, Benghozi R. Drug attrition during pre-clinical and clinical development: understanding and managing drug-induced cardiotoxicity. Pharmacol Ther. 2013;138(3):470-484. doi: 10.1016/j.pharmthera.2013.03.005
- Ho D, Zhao X, Gao S, Hong C, Vatner DE, Vatner SF. Heart rate and electrocardiography monitoring in mice. Curr Protoc Mouse Biol. 2011;1(1):123-139. doi: 10.1002/9780470942390.mo100159
- Wolfe JT, He W, Kim M-S, et al. 3D-bioprinting of patient-derived cardiac tissue models for studying congenital heart disease. Front Cardiovasc Med. 2023;10:1162731. doi: 10.3389/fcvm.2023.1162731
- Faulkner-Jones A, Zamora V, Hortigon-Vinagre MP, et al. A bioprinted heart-on-a-chip with human pluripotent stem cell-derived cardiomyocytes for drug evaluation. Bioengineering. 2022;9(1):32. doi: 10.3390/bioengineering9010032
- Das S, Kim S-W, Choi Y-J, et al. Decellularized extracellular matrix bioinks and the external stimuli to enhance cardiac tissue development in vitro. Acta Biomater. 2019;95:188-200. doi: 10.1016/j.actbio.2019.04.026
- Min S, Cho S-W. Engineered human cardiac tissues for modeling heart diseases. BMB Rep. 2023;56(1):32. doi: 10.5483/BMBRep.2022-0185
- Eng G, Lee BW, Protas L, et al. Autonomous beating rate adaptation in human stem cell-derived cardiomyocytes. Nat Commun. 2016;7(1):10312. doi: 10.1038/ncomms10312
- Ronaldson-Bouchard K, Ma SP, Yeager K, et al. Advanced maturation of human cardiac tissue grown from pluripotent stem cells. Nature. 2018;556(7700):239-243. doi: 10.1038/s41586-018-0016-3
- Lasli S, Kim HJ, Lee K, et al. A human liver‐on‐a‐chip platform for modeling nonalcoholic fatty liver disease. Adv Biosyst. 2019;3(8):1900104. doi: 10.1002/adbi.201900104
- Ozougwu JC. Physiology of the liver. Int J Res Pharm Biosci. 2017;4(8):13-24.
- Bellentani S. The epidemiology of non‐alcoholic fatty liver disease. Liver Int. 2017;37:81-84. doi: 10.1111/liv.13299
- Friedman SL. Liver fibrosis–from bench to bedside. J Hepatol. 2003;38:38-53. doi: 10.1016/S0168-8278(02)00429-4
- Border WA, Noble NA. Transforming growth factor β in tissue fibrosis. N Engl J Med. 1994;331(19):1286-1292. doi: 10.1056/NEJM199411103311907
- Yanni SB, Augustijns PF, Benjamin DK, Brouwer KLR, Thakker DR, Annaert PP. In vitro investigation of the hepatobiliary disposition mechanisms of the antifungal agent micafungin in humans and rats. Drug Metab Dispos. 2010;38(10):1848-1856. doi: 10.1124/dmd.110.033811
- LeCluyse EL, Audus KL, Hochman JH. Formation of extensive canalicular networks by rat hepatocytes cultured in collagen-sandwich configuration. Am J Physiol: Cell Physiol. 1994;266(6):C1764-C1774. doi: 10.1152/ajpcell.1994.266.6.C1764
- De Graaf IA, Olinga P, De Jager MH, et al. Preparation and incubation of precision-cut liver and intestinal slices for application in drug metabolism and toxicity studies. Nat. Protoc. 2010;5(9):1540-1551. doi: 10.1038/nprot.2010.111
- Du Y, Li N, Yang H, et al. Mimicking liver sinusoidal structures and functions using a 3D-configured microfluidic chip. Lab Chip. 2017;17(5):782-794. doi: 10.1039/C6LC01374K
- Vollmar B, Menger MD. The hepatic microcirculation: mechanistic contributions and therapeutic targets in liver injury and repair. Physiol Rev. 2009;89(4):1269-1339. doi: 10.1152/physrev.00027.2008
- Wisse E, De Zanger R, Charels K, van der Smissen P, McCuskey RS. The liver sieve: considerations concerning the structure and function of endothelial fenestrae, the sinusoidal wall and the space of Disse. Hepatology. 1985;5(4):683-692. doi: 10.1002/hep.1840050427
- Berger DR, Ware BR, Davidson MD, Allsup SR, Khetani SR. Enhancing the functional maturity of induced pluripotent stem cell–derived human hepatocytes by controlled presentation of cell–cell interactions in vitro. Hepatology. 2015;61(4):1370-1381. doi: 10.1002/hep.27621
- Petronis S, Eckert K-L, Gold J, Wintermantel E. Microstructuring ceramic scaffolds for hepatocyte cell culture. J Mater Sci: Mater Med. 2001;12:523-528. doi: 10.1023/A:1011219729687
- Rennert K, Steinborn S, Gröger M, et al. A microfluidically perfused three dimensional human liver model. Biomaterials. 2015;71:119-131. doi: 10.1016/j.biomaterials.2015.08.043
- Yamada M, Utoh R, Ohashi K, et al. Controlled formation of heterotypic hepatic micro-organoids in anisotropic hydrogel microfibers for long-term preservation of liver-specific functions. Biomaterials. 2012;33(33): 8304-8315. doi: 10.1016/j.biomaterials.2012.07.068
- Lee H, Chae S, Kim JY, et al. Cell-printed 3D liver-on-a-chip possessing a liver microenvironment and biliary system. Biofabrication. 2019;11(2):025001. doi: 10.1088/1758-5090/aaf9fa
- Carvalho V, Gonçalves I, Lage T, et al. 3D printing techniques and their applications to organ-on-a-chip platforms: a systematic review. Sensors. 2021; 21(9):3304. doi: 10.3390/s21093304
- Mazzocchi A, Soker S, Skardal A. 3D bioprinting for high-throughput screening: drug screening, disease modeling, and precision medicine applications. Appl Phys Rev. 2019;6(1):011302. doi: 10.1063/1.5056188
- Kang HK, Sarsenova M, Kim D-H, et al. Establishing a 3D in vitro hepatic model mimicking physiologically relevant to in vivo state. Cells. 2021;10(5):1268. doi: 10.3390/cells10051268
- Lee H, Han W, Kim H, et al. Development of liver decellularized extracellular matrix bioink for three-dimensional cell printing-based liver tissue engineering. Biomacromolecules. 2017;18(4):1229-1237. doi: 10.1021/acs.biomac.6b01908
- Lee H, Kim J, Choi Y, Cho D-W. Application of gelatin bioinks and cell-printing technology to enhance cell delivery capability for 3D liver fibrosis-on-a-chip development. ACS Biomater Sci Eng. 2020;6(4):2469-2477. doi: 10.1021/acsbiomaterials.9b01735
- Taymour R, Kilian D, Ahlfeld T, Gelinsky M, Lode A. 3D bioprinting of hepatocytes: core–shell structured co-cultures with fibroblasts for enhanced functionality. Sci Rep. 2021;11(1):5130. doi: 10.1038/s41598-021-84384-6
- Wang T, Du Z, Zhu F, et al. Comorbidities and multi-organ injuries in the treatment of COVID-19. Lancet. 2020;395(10228):e52. doi: 10.1016/S0140-6736(20)30558-4
- Sahlman P, Nissinen M, Puukka P, et al. Genetic and lifestyle risk factors for advanced liver disease among men and women. J Gastroenterol Hepatol. 2020;35(2):291-298. doi: 10.1111/jgh.14770
- Yang H, Sun L, Pang Y, et al. Three-dimensional bioprinted hepatorganoids prolong survival of mice with liver failure. Gut. 2021;70(3):567-574. doi: 10.1136/gutjnl-2019-319960
- Jin B, Liu Y, Du S, Sang X, Yang H. Current trends and research topics regarding liver 3D bioprinting: a bibliometric analysis research. Front Cell Dev Biol. 2022;10:1047524. doi: 10.3389/fcell.2022.1047524
- Deng J, Wei W, Chen Z, et al. Engineered liver-on-a-chip platform to mimic liver functions and its biomedical applications: a review. Micromachines. 2019;10(10):676. doi: 10.3390/mi10100676
- Shinozawa T, Kimura M, Cai Y, et al. High-fidelity drug-induced liver injury screen using human pluripotent stem cell– derived organoids. Gastroenterology. 2021;160(3):831-846.e10. doi: 10.1053/j.gastro.2020.10.002
- Man WH, de Steenhuijsen Piters WA, Bogaert D. The microbiota of the respiratory tract: gatekeeper to respiratory health. Nat Rev Microbiol. 2017;15(5):259-270. doi: 10.1038/nrmicro.2017.14
- Comroe JH. The lung. Sci Am. 1966;214(2):56-71. https://jstor.org/stable/24931268
- Mccracken Jr GH. Diagnosis and management of pneumonia in children. Pediatr Infect Dis J. 2000;19(9):924-928. doi: 10.1097/00006454-200009000-00036
- Bateman ED, Reddel HK, van Zyl-Smit RN, Agusti A. The asthma–COPD overlap syndrome: towards a revised taxonomy of chronic airways diseases? Lancet Respir Med. 2015;3(9):719-728. doi: 10.1016/S2213-2600(15)00254-4
- Flume PA, Van Devanter DR. State of progress in treating cystic fibrosis respiratory disease. BMC Med. 2012;10(1):88. doi: 10.1186/1741-7015-10-88
- Antonelli M, Conti G, Bufi M, et al. Noninvasive ventilation for treatment of acute respiratory failure in patients undergoing solid organ transplantation: a randomized trial. JAMA. 2000;283(2):235-241. doi: 10.1001/jama.283.2.235
- Cidem A, Bradbury P, Traini D, Ong HX. Modifying and integrating in vitro and ex vivo respiratory models for inhalation drug screening. Front Bioeng Biotechnol. 2020;8:581995. doi: 10.3389/fbioe.2020.581995
- Baldassi D, Gabold B, Merkel OM. Air−liquid Interface cultures of the healthy and diseased human respiratory tract: promises, challenges, and future directions. Adv Nanobiomed Res. 2021;1(6):2000111. doi: 10.1002/anbr.202000111
- Zheng F, Fu F, Cheng Y, Wang C, Zhao Y, Gu Z. Organ‐on‐a‐chip systems: microengineering to biomimic living systems. Small. 2016;12(17):2253-2282. doi: 10.1002/smll.201503208
- Horváth L, Umehara Y, Jud C, Blank F, Petri-Fink A, Rothen- Rutishauser B. Engineering an in vitro air-blood barrier by 3D bioprinting. Sci Rep. 2015;5(1):7974. doi: 10.1038/srep07974
- Osório LA, Silva E, Mackay RE. A review of biomaterials and scaffold fabrication for organ-on-a-chip (OOAC) systems. Bioengineering. 2021;8(8):113. doi: 10.3390/bioengineering8080113
- Humayun M, Chow C-W, Young EW. Microfluidic lung airway-on-a-chip with arrayable suspended gels for studying epithelial and smooth muscle cell interactions. Lab Chip. 2018;18(9):1298-1309. doi: 10.1039/C7LC01357D
- Benam K H, Villenave R, Lucchesi C, et al. Small airway-on-a-chip enables analysis of human lung inflammation and drug responses in vitro. Nat Methods. 2016;13(2):151-157. doi: 10.1038/nmeth.3697
- Sellgren KL, Butala EJ, Gilmour BP, Randell SH, Grego S. A biomimetic multicellular model of the airways using primary human cells. Lab Chip. 2014;14(17):3349-3358. doi: 10.1039/C4LC00552J
- Nalayanda DD, Puleo C, Fulton WB, Sharpe LM, Wang T-H, Abdullah F. An open-access microfluidic model for lung-specific functional studies at an air-liquid interface. Biomed Microdevices. 2009;11:1081-1089. doi: 10.1007/s10544-009-9325-5
- Douville NJ, Zamankhan P, Tung Y-C, et al. Combination of fluid and solid mechanical stresses contribute to cell death and detachment in a microfluidic alveolar model. Lab Chip. 2011;11(4):609-619. doi: 10.1039/C0LC00251H
- Huh D, Matthews BD, Mammoto A, Montoya-Zavala M, Hsin HY, Ingber DE. Reconstituting organ-level lung functions on a chip. Science. 2010;328(5986):1662-1668. doi: 10.1126/science.1188302
- Bennet TJ, Randhawa A, Hua J, Cheung KC. Airway-on-a-chip: designs and applications for lung repair and disease. Cells. 2021;10(7):1602. doi: 10.3390/cells10071602
- Higham A, Bostock D, Booth G, Dungwa JV, Singh D. The effect of electronic cigarette and tobacco smoke exposure on COPD bronchial epithelial cell inflammatory responses. Int J Chronic Obstruct Pulm Dis. 2018;13:989-1000. doi: 10.2147/COPD.S157728
- de Boer JD, Majoor CJ, van’t Veer C, Bel EHD, van der Poll T. Asthma and coagulation. Blood. 2012;119(14):3236-3244. doi: 10.1182/blood-2011-11-391532
- Guo R, Pittler M, Ernst E. Herbal medicines for the treatment of COPD: a systematic review. Eur Respir J. 2006;28(2):330-338. doi: 10.1183/09031936.06.00119905
- Park JY, Ryu H, Lee B, et al. Development of a functional airway-on-a-chip by 3D cell printing. Biofabrication. 2018;11(1):015002. doi: 10.1088/1758-5090/aae545
- Nam H, Choi Y-m, Cho S, et al. Modular assembly of bioprinted perfusable blood vessel and tracheal epithelium for studying inflammatory respiratory diseases. Biofabrication. 2022;15(1):014101. doi: 10.1088/1758-5090/ac93b6
- Gu Z, Fu J, Lin H, He Y. Development of 3D bioprinting: from printing methods to biomedical applications. Asian J Pharm Sci. 2020;15(5):529-557. doi: 10.1016/j.ajps.2019.11.003
- Chen EP, Toksoy Z, Davis BA, Geibel JP. 3D bioprinting of vascularized tissues for in vitro and in vivo applications. Front Bioeng Biotechnol. 2021;9:664188. doi: 10.3389/fbioe.2021.664188
- Kuperman DA, Huang X, Koth LL, et al. Direct effects of interleukin-13 on epithelial cells cause airway hyperreactivity and mucus overproduction in asthma. Nat Med. 2002;8(8):885-889. doi: 10.1038/nm734
- Gao G, Lee JH, Jang J, et al. Tissue engineered bio‐blood‐vessels constructed using a tissue‐specific bioink and 3D coaxial cell printing technique: a novel therapy for ischemic disease. Adv Funct Mater. 2017;27(33):1700798. doi: 10.1038/nm734
- Luster AD, Alon R, von Andrian UH. Immune cell migration in inflammation: present and future therapeutic targets. Nat Immunol. 2005;6(12):1182-1190. doi: 10.1038/ni1275
- Park JH, Ahn M, Park SH, et al. 3D bioprinting of a trachea-mimetic cellular construct of a clinically relevant size. Biomaterials. 2021;279:121246. doi: 10.1016/j.biomaterials.2021.121246
- Nizamoglu M, Joglekar MM, Almeida CR, et al. Innovative three-dimensional models for understanding mechanisms underlying lung diseases: powerful tools for translational research. Eur Respir Rev. 2023;32(169). doi: 10.1183/16000617.0042-2023
- Barreiro Carpio M, Dabaghi M, Ungureanu J, et al. 3D bioprinting strategies, challenges, and opportunities to model the lung tissue microenvironment and its function. Front Bioeng Biotechnol. 2021;9:773511. doi: 10.3389/fbioe.2021.773511
- Cho WW, Ahn M, Kim BS, et al. Blood‐lymphatic integrated system with heterogeneous melanoma spheroids via in‐bath three‐dimensional bioprinting for modelling of combinational targeted therapy. Adv Sci. 2022;9(29): 2202093. doi: 10.1002/advs.202202093
- Alencar AM, Arold SP, Buldyrev SV, et al. Dynamic instabilities in the inflating lung. Nature. 2002;417(6891): 809-811. doi: 10.1038/417809b
- Sengupta A, Roldan N, Kiener M, et al. A new immortalized human alveolar epithelial cell model to study lung injury and toxicity on a breathing lung-on-chip system. Front Toxicol. 2022;4:840606. doi: 10.3389/ftox.2022.840606
- Yi H-G, Kim H, Kwon J, et al. Application of 3D bioprinting in the prevention and the therapy for human diseases. Signal Transduction Targeted Ther. 2021;6(1):177. doi: 10.1038/s41392-021-00566-8
- Vanaei S, Parizi MS, Vanaei S, Salemizadehparizi F, Vanaei HR. An overview on materials and techniques in 3D bioprinting toward biomedical application. Eng Regener. 2021;2:1-18. doi: 10.1016/j.engreg.2020.12.001
- Malda J, Visser J, Melchels FP, et al. 25th anniversary article: engineering hydrogels for biofabrication. Adv Mater. 2013;25(36):5011-5028. doi: 10.1002/adma.201302042
- Khoshnood N, Zamanian A. A comprehensive review on scaffold-free bioinks for bioprinting. Bioprinting. 2020;19:e00088. doi: 10.1016/j.bprint.2020.e00088
- Ali M, Pr AK, Yoo JJ, Zahran F, Atala A, Lee SJ. A photo‐crosslinkable kidney ECM‐derived bioink accelerates renal tissue formation. Adv Healthc Mater. 2019;8(7):1800992. doi: 10.1002/adhm.201800992
- Kim H, Kang B, Cui X, et al. Light‐activated decellularized extracellular matrix‐based bioinks for volumetric tissue analogs at the centimeter scale. Adv Funct Mater. 2021;31(32):2011252. doi: 10.1002/adfm.202011252
- Brassard JA, Nikolaev M, Hübscher T, Hofer M, Lutolf MP. Recapitulating macro-scale tissue self-organization through organoid bioprinting. Nat Mater. 2021;20(1):22-29. doi: 10.1038/s41563-020-00803-5
- Lee VK, Dai G. Three-dimensional bioprinting and tissue fabrication: prospects for drug discovery and regenerative medicine. Adv Healthc Technol. 2015;2015:23-35. doi: 10.2147/AHCT.S69191
- Topuz B, Günal G, Guler S, Aydin HM. Use of supercritical CO2 in soft tissue decellularization. Methods Cell Biol. 2020;157:49-79. doi: 10.1016/bs.mcb.2019.10.012
- Mostow EN, Haraway GD, Dalsing M, Hodde JP, King D. Effectiveness of an extracellular matrix graft (OASIS Wound Matrix) in the treatment of chronic leg ulcers: a randomized clinical trial. J Vasc Surg. 2005;41(5):837-843. doi: 10.1016/j.jvs.2005.01.042
- Wainwright D. Use of an acellular allograft dermal matrix (AlloDerm) in the management of full-thickness burns. Burns. 1995;21(4):243-248. doi: 10.1016/0305-4179(95)93866-I
- Kirillova A, Bushev S, Abubakirov A, Sukikh G. Bioethical and legal issues in 3D bioprinting. Int J Bioprint. 2020;6(3). doi: 10.18063/ijb.v6i3.272
- Jessop ZM, Al-Sabah A, Gardiner MD, Combellack E, Hawkins K, Whitaker IS. 3D bioprinting for reconstructive surgery: principles, applications and challenges. J Plast Reconstr. Aesthetic Surg. 2017;70(9):1155-1170. doi: 10.1016/j.bjps.2017.06.001
- Salmikangas P, Schuessler-Lenz M, Ruiz S, et al. Marketing regulatory oversight of advanced therapy medicinal products (ATMPs) in Europe: the EMA/CAT perspective. Regul Aspects Gene Ther Cell Ther Prod. 2015:103-130. doi: 10.1007/978-3-319-18618-4_6
- Shafiq MA, Gemeinhart RA, Yue BY, Djalilian AR. Decellularized human cornea for reconstructing the corneal epithelium and anterior stroma. Tissue Eng Part C. 2012;18(5):340-348. doi: 10.1089/ten.tec.2011.0072
- Polisetti N, Schmid A, Schlötzer-Schrehardt U, et al. A decellularized human corneal scaffold for anterior corneal surface reconstruction. Sci Rep. 2021;11(1):1-15. doi: 10.1038/s41598-021-82678-3
- Gonzalez-Andrades M, de la Cruz Cardona J, Ionescu AM, Campos A, del Mar Perez M, Alaminos M. Generation of bioengineered corneas with decellularized xenografts and human keratocytes. Invest Ophthalmol Visual Sci. 2011;52(1):215-222. doi: 10.1167/iovs.09-4773
- Ventura RD, Padalhin AR, Park CM, Lee BT. Enhanced decellularization technique of porcine dermal ECM for tissue engineering applications. Mater Sci Eng C. 2019;104:109841. doi: 10.1016/j.msec.2019.109841
- Funamoto S, Nam K, Kimura T, et al. The use of high-hydrostatic pressure treatment to decellularize blood vessels. Biomaterials. 2010;31(13):3590-3595. doi: 10.1016/j.biomaterials.2010.01.073
- Jang J, Park H-J, Kim S-W, et al. 3D printed complex tissue construct using stem cell-laden decellularized extracellular matrix bioinks for cardiac repair. Biomaterials. 2017;112: 264-274. doi: 10.1016/j.biomaterials.2016.10.026
- Quint C, Kondo Y, Manson RJ, Lawson JH, Dardik A, Niklason LE. Decellularized tissue-engineered blood vessel as an arterial conduit. Proc Natl Acad Sci. 2011;108(22): 9214-9219. doi: 10.1073/pnas.1019506108
- Flynn L. The use of decellularized adipose tissue to provide an inductive microenvironment for the adipogenic differentiation of human adipose-derived stem cells. Biomaterials. 2010;31(17):4715-4724. doi: 10.1016/j.biomaterials.2010.02.046
- Banyard DA, Sarantopoulos C, Tassey J, et al. Preparation, characterization, and clinical implications of human decellularized adipose tissue extracellular matrix. In: Duscher D, Shiffman MA, eds. Regenerative Medicine and Plastic Surgery. Cham: Springer; 2019:71-89. doi: 10.1007/978-3-030-19962-3_6
- Kang H, Peng J, Lu S, et al. In vivo cartilage repair using adipose‐derived stem cell‐loaded decellularized cartilage ECM scaffolds. J Tissue Eng Regener Med. 2014;8(6): 442-453. doi: 10.1002/term.1538
- Elder BD, Kim DH, Athanasiou KA. Developing an articular cartilage decellularization process toward facet joint cartilage replacement. Neurosurgery. 2010;66(4):722-727. doi: 10.1227/01.NEU.0000367616.49291.9F
- Bae M, Ko MK, Jin Y, et al. Neural stem cell delivery using brain-derived tissue-specific bioink for recovering from traumatic brain injury. Biofabrication. 2021;13(4): 044110. doi: 10.1088/1758-5090/ac293f
- Min K, Kong JS, Kim J, et al. Three-dimensional microfilament printing of a decellularized extracellular matrix (dECM) bioink using a microgel printing bath for nerve graft fabrication and the effectiveness of dECM graft combined with a polycaprolactone conduit. ACS Appl Biomater. 2022;5(4):1591-1603.doi: 10.1021/acsabm.1c01142
- Chen C-C, Yu J, Ng H-Y, et al. The physicochemical properties of decellularized extracellular matrix-coated 3D printed poly (ε-caprolactone) nerve conduits for promoting Schwann cells proliferation and differentiation. Materials. 2018;11(9):1665. doi: 10.3390/ma11091665
- Noor N, Shapira A, Edri R, Gal I, Wertheim L, Dvir T. 3D printing of personalized thick and perfusable cardiac patches and hearts. Adv Sci. 2019; 6(11):1900344. doi: 10.1002/advs.201900344