Effect of lattice type on biomechanical and osseointegration properties of 3D-printed porous Ti6Al4V scaffolds
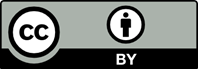
Porous structure is an efficient tool for optimizing the elastic modulus and osseointegration properties of titanium alloy materials. However, the investigations on pore shape remain scarce. In this study, we created porous Ti6Al4V scaffolds with a pore size of 600 μm but different lattices (cubic pentagon, diamond, cuboctahedron). The mechanical and biological properties of the scaffolds were investigated in static simulation analysis, in vitro mechanical compression test, computational fluid dynamics, as well as cell and animal experiments. The results demonstrated that the calculated yield strength difference between the three Ti6Al4V porous scaffolds was negligible, at approximately 140 MPa, allowing them to match the strength requirements of human bones. The diamond scaffold has the lowest calculated elastic modulus (11.6 GPa), which is conducive for preventing stress shielding. The shear stress was largely concentrated in the diamond scaffold, and the stress range of 120–140 MPa accounted for the greatest share. The mouse MC3T3-E1 cells were found to attach to all three scaffolds, with the diamond scaffold displaying a higher degree of cell adherence. There was more proliferating cells on the diamond and cubic pentagon scaffolds than on the cuboctahedron scaffolds (P < 0.05). The diamond scaffold exhibited the highest alkaline phosphatase activity and calcium salt accumulation in cell differentiation tests. Besides, the expression of osteogenic genes on the diamond scaffold was higher than that on the cuboctahedron scaffold, the cubic pentagon scaffold displaying the lowest expression. The in vivo studies revealed that all three scaffolds fused well with the surrounding bone and that there was no loosening or movement of the prosthesis. Micro-computed tomography, corroborated by the staining results of hard tissues, revealed that the level of new bone formation was the highest in the diamond scaffold, followed by the cuboctahedron scaffold (P < 0.05). Taken together, the diamond scaffold is comparatively better at optimizing the elastic modulus and osseointegration properties of titanium alloy materials, and thus is a preferred choice for porous design.
- Neto MQ, Radice S, Hall DJ, et al. Microstructure and electrochemical behavior of contemporary Ti6Al4V implant alloys. J Bio Tribocorros. 2022;8(1). doi: 10.1007/s40735-021-00623-3
- Xi D, Wong L. Titanium and implantology: A review in dentistry. J Biol Regul Homeost Agents. 2021;35(1 Suppl. 1):63- 72. doi: 10.3390%2Fma15093150
- Gkiatas I, Sharma AK, Driscoll DA, McLawhorn AS, Chalmers BP, Sculco PK. Nonconcentric and irregular dislocations of total hip arthroplasties: Radiographic analysis and review of the literature. J Emerg Med. 2021;60(4): 451-459. doi: 10.1016/j.jemermed.2020.11.023
- Liu T, Hua X, Yu W, et al. Long-term follow-up outcomes for patients undergoing primary total hip arthroplasty with uncemented versus cemented femoral components: A retrospective observational study with a 5-year minimum follow-up. J Orthop Surg Res. 2019;14(1):371. doi: 10.1186/s13018-019-1415-3
- Raja BS, Gowda A, Singh S, Ansari S, Kalia RB, Paul S. Comparison of functional outcomes and complications of cemented vs uncemented total hip arthroplasty in the elderly neck of femur fracture patients: A systematic review and meta-analysis. J Clin Orthop Trauma. 2022;29:101876. doi: 10.1016%2Fj.jcot.2022.101876
- Bittredge O, Hassanin H, El-Sayed MA, et al. Fabrication and optimisation of Ti-6Al-4V lattice-structured total shoulder implants using laser additive manufacturing. Materials (Basel). 2022;15(9). doi: 10.3390/ma15093095
- Ito K, Mori Y, Kamimura M, et al. Beta-type TiNbSn alloy plates with low Young modulus accelerates osteosynthesis in Rabbit Tibiae. Clin Orthop Relat Res. 2022;480(9):1817-1832. doi: 10.1097/corr.0000000000002240
- Lei P, Qian H, Zhang T, et al. Porous tantalum structure integrated on Ti6Al4V base by laser powder bed fusion for enhanced bony-ingrowth implants: In vitro and in vivo validation. Bioact Mater. 2022;7:3-13. doi: 10.1016/j.bioactmat.2021.05.025
- Li L, Li Y, Yang L, et al. Polydopamine coating promotes early osteogenesis in 3D printing porous Ti6Al4V scaffolds. Ann Transl Med. 2019;7(11):240. doi: 10.21037/atm.2019.04.79
- Meena VK, Kalra P, Sinha RK. Finite element study on the influence of pore size and structure on stress shielding effect of additive manufactured spinal cage. Comput Methods Biomech Biomed Engin. 2022;25(5):566-577. doi: 10.1080/10255842.2021.1970142
- Al-Tamimi AA. 3D topology optimization and mesh dependency for redesigning locking compression plates aiming to reduce stress shielding. Int J Bioprint. 2021;7(3):339. doi: 10.18063/ijb.v7i3.339
- Han X, Ma J, Tian A, et al. Surface modification techniques of titanium and titanium alloys for biomedical orthopaedics applications: A review. Colloids Surf B Biointerfaces. 2023;227:113339. doi: 10.1016/j.colsurfb.2023.113339
- Xia C, Ma X, Zhang X, et al. Enhanced physicochemical and biological properties of C/Cu dual ions implanted medical titanium. Bioact Mater. 2020;5(2):377-386. doi: 10.1016/j.bioactmat.2020.02.017
- Wang Q, Zhou P, Liu S, et al. 2020, Multi-scale surface treatments of titanium implants for rapid osseointegration: A review. Nanomaterials (Basel), 10(6). doi: 10.3390%2Fnano10061244
- Wang L, Zhou W, Yu Z, et al. An in vitro evaluation of the hierarchical micro/nanoporous structure of a Ti3Zr2Sn3Mo25Nb alloy after surface dealloying. ACS Appl Mater Interfaces. 2021;13(13):15017-15030. doi: 10.1021/acsami.1c02140
- Wang R, Ni S, Ma L, Li M. Porous construction and surface modification of titanium-based materials for osteogenesis: A review. Front Bioeng Biotechnol. 2022; 10:973297. doi: 10.3389%2Ffbioe.2022.973297
- Liverani E, Rogati G, Pagani S, Brogini S, Fortunato A, Caravaggi P. Mechanical interaction between additive-manufactured metal lattice structures and bone in compression: Implications for stress shielding of orthopaedic implants. J Mech Behav Biomed Mater. 2021;121:104608. doi: 10.1016/j.jmbbm.2021.104608
- Arabnejad S, Johnston B, Tanzer M, Pasini D. Fully porous 3D printed titanium femoral stem to reduce stress-shielding following total hip arthroplasty. J Orthop Res. 2017; 35(8):1774-1783. doi: 10.1002/jor.23445
- Yao YT, Yang Y, Ye Q, et al. Effects of pore size and porosity on cytocompatibility and osteogenic differentiation of porous titanium. J Mater Sci Mater Med. 2021;32(6):72. doi: 10.1007/s10856-021-06548-0
- Cuan-Urquizo E, Silva RG. Fused filament fabrication of cellular, lattice and porous mechanical metamaterials: A review. Virtual Phys Prototyp. 2023;1(18):e2224300. doi: 10.1080/17452759.2023.2224300
- Yan L, Wu J, Zhang L, Liu X, Zhou K, Su B. Pore structures and mechanical properties of porous titanium scaffolds by bidirectional freeze casting. Mater Sci Eng C Mater Biol Appl. 2017;75:335-340. doi: 10.1016/j.msec.2016.12.044
- Dziaduszewska M, Zielinski A. Structural and material determinants influencing the behavior of porous ti and its alloys made by additive manufacturing techniques for biomedical applications. Materials (Basel). 2021;14(4). doi: 10.3390/ma14040712
- Xu Z, Zhang Y, Wu Y, et al. In vitro and in vivo analysis of the effects of 3D-printed porous titanium alloy scaffold structure on osteogenic activity. Biomed Res Int. 2022;2022:8494431. doi: 10.1155/2022/8494431
- Wang H, Su K, Su L, Liang P, Ji P, Wang C. Comparison of 3D-printed porous tantalum and titanium scaffolds on osteointegration and osteogenesis. Mater Sci Eng C Mater Biol Appl. 2019;104:109908. doi: 10.1016/j.msec.2019.109908
- Yang J, Li Y, Shi X, et al. Design and analysis of three-dimensional printing of a porous titanium scaffold. BMC Musculoskelet Disord. 2021;22(1):654. doi: 10.1186/s12891-021-04520-1
- Afrouzian A, Groden CJ, Field DP, Bose S, Bandyopadhyay A. Additive manufacturing of Ti-Ni bimetallic structures. Mater Des. 2022;215. doi: 10.1016%2Fj.matdes.2022.110461
- Huang G, Pan ST, Qiu JX. The osteogenic effects of porous Tantalum and Titanium alloy scaffolds with different unit cell structure. Colloids Surf B Biointerfaces. 2022;210:112229. doi: 10.1016/j.colsurfb.2021.112229
- Ciliveri S, Bandyopadhyay A. Influence of strut-size and cell-size variations on porous Ti6Al4V structures for load-bearing implants. J Mech Behav Biomed Mater. 2022;126:105023. doi: 10.1016/j.jmbbm.2021.105023
- Deng F, Liu L, Li Z, Liu J. 3D printed Ti6Al4V bone scaffolds with different pore structure effects on bone ingrowth. J Biol Eng. 2021;15(1):4. doi: 10.1186/s13036-021-00255-8
- Lei H, Yi T, Fan H, et al. Customized additive manufacturing of porous Ti6Al4V scaffold with micro-topological structures to regulate cell behavior in bone tissue engineering. Mater Sci Eng C Mater Biol Appl. 2021;120:111789. doi: 10.1016/j.msec.2020.111789
- Suresh S, Sun CN, Tekumalla S, Rosa V, Ling Nai SM, Wen Wong RC. Mechanical properties and in vitro cytocompatibility of dense and porous Ti-6Al-4V ELI manufactured by selective laser melting technology for biomedical applications. J Mech Behav Biomed Mater. 2021;123:104712. doi: 10.1016/j.jmbbm.2021.104712
- Ran Q, Yang W, Hu Y, et al. Osteogenesis of 3D printed porous Ti6Al4V implants with different pore sizes. J Mech Behav Biomed Mater. 2018;84:1-11. doi: 10.1016/j.jmbbm.2018.04.010
- Zaharin HA, Abdul RA, Azam FI, et al. Effect of unit cell type and pore size on porosity and mechanical behavior of additively manufactured Ti6Al4V scaffolds. Materials (Basel). 2018;11(12). doi: 10.3390%2Fma11122402
- Taniguchi N, Fujibayashi S, Takemoto M, et al. Effect of pore size on bone ingrowth into porous titanium implants fabricated by additive manufacturing: An in vivo experiment. Mater Sci Eng C Mater Biol Appl. 2016;59:690-701. doi: 10.1016/j.msec.2015.10.069
- Lu Y, Cheng L, Yang Z, Li J, Zhu H. Relationship between the morphological, mechanical and permeability properties of porous bone scaffolds and the underlying microstructure. PLoS One. 2020;15(9):e238471. doi: 10.1371%2Fjournal.pone.0238471
- Chen H, Han Q, Wang C, Liu Y, Chen B, Wang J. Porous scaffold design for additive manufacturing in orthopedics: A review. Front Bioeng Biotechnol. 2020;8:609. doi: 10.3389/fbioe.2020.00609
- Li X, Wang Y, Zhang B, et al. The design and evaluation of bionic porous bone scaffolds in fluid flow characteristics and mechanical properties. Comput Methods Programs Biomed. 2022;225:107059. doi: 10.1016/j.cmpb.2022.107059
- Omar AM, Hassan MH, Daskalakis E, et al. Geometry-based computational fluid dynamic model for predicting the biological behavior of bone tissue engineering scaffolds. J Funct Biomater. 2022;13(3). doi: 10.3390/jfb13030104
- Chao L, Jiao C, Liang H, Xie D, Shen L, Liu Z. Analysis of mechanical properties and permeability of trabecular-like porous scaffold by additive manufacturing. Front Bioeng Biotechnol. 2021;9:779854. doi: 10.3389%2Ffbioe.2021.779854
- Zhang C, Zhu H, Ren X, et al. Mechanics-driven nuclear localization of YAP can be reversed by N-cadherin ligation in mesenchymal stem cells. Nat Commun. 2021;12(1):6229. doi: 10.1038/s41467-021-26454-x
- Zhang Z, Sha B, Zhao L, et al. Programmable integrin and N-cadherin adhesive interactions modulate mechanosensing of mesenchymal stem cells by cofilin phosphorylation. Nat Commun. 2022;13(1):6854. doi: 10.1038/s41467-022-34424-0
- De Belly H, Paluch EK, Chalut KJ. Interplay between mechanics and signalling in regulating cell fate. Nat Rev Mol Cell Biol. 2022;23(7):465-480. doi: 10.1038/s41580-022-00472-z
- Truscello S, Kerckhofs G, Van Bael S, Pyka G, Schrooten J, Van Oosterwyck H. Prediction of permeability of regular scaffolds for skeletal tissue engineering: A combined computational and experimental study. Acta Biomater. 2012;8(4):1648-1658. doi: 10.1016/j.actbio.2011.12.021
- Winther NS, Jensen CL, Jensen CM, et al. Comparison of a novel porous titanium construct (Regenerex(R)) to a well proven porous coated tibial surface in cementless total knee arthroplasty - A prospective randomized RSA study with two-year follow-up. Knee. 2016;23(6): 1002-1011. doi: 10.1016/j.knee.2016.09.010
- Arts M, Torensma B, Wolfs J. Porous titanium cervical interbody fusion device in the treatment of degenerative cervical radiculopathy; 1-year results of a prospective controlled trial. Spine J. 2020;20(7):1065-1072. doi: 10.1016/j.spinee.2020.03.008
- Ibhadode O, Zhang Z, Sixt J, et al. Topology optimization for metal additive manufacturing: current trends, challenges, and future outlook. Virtual Phys Prototyp. 2023;1(18):e2181192. doi: 10.1080/17452759.2023.2181192
- Ahmadi SM, Yavari SA, Wauthle R, et al. Additively manufactured open-cell porous biomaterials made from six different space-filling unit cells: The mechanical and morphological properties. Materials (Basel). 2015;8(4):1871-1896. doi: 10.3390%2Fma8041871
- Hedayati R, Sadighi M, Mohammadi-Aghdam M, et al. Mechanics of additively manufactured porous biomaterials based on the rhombicuboctahedron unit cell. J Mech Behav Biomed Mater. 2016;53:272-294. doi: 10.1016/j.jmbbm.2015.07.013
- Matena J, Petersen S, Gieseke M, et al. SLM produced porous titanium implant improvements for enhanced vascularization and osteoblast seeding. Int J Mol Sci; 2015;16(4):7478-7492. doi: 10.3390%2Fijms16047478
- Gogolewski D, Kozior T, Zmarzly P, Gogolewski D. Morphology of models manufactured by SLM technology and the Ti6Al4V titanium alloy designed for medical applications. Materials (Basel). 2021;14(21). doi: 10.3390/ma14216249
- Ataee A, Li Y, Brandt M, Wen C. Ultrahigh-strength titanium gyroid scaffolds manufactured by selective laser melting (SLM) for bone implant applications. Acta Materialia. 2018;158:15. doi: 10.3390%2Fjfb14030125
- Li JL, Guo D, L J, et al. Irregular pore size of degradable bioceramic Voronoi scaffolds prepared by stereolithography: Osteogenesis and computational fluid dynamics analysis. Mater Des. 2022;224:111414. doi: 10.1016/j.matdes.2022.111414
- Li J, Chen D, Luan H, Zhang Y, Fan Y. Numerical evaluation and prediction of porous implant design and flow performance. Biomed Res Int. 2018;2018:1215021. doi: 10.1155%2F2018%2F1215021
- Li J, Chen D, Fan Y, Evaluation and prediction of mass transport properties for porous implant with different unit cells: A numerical study. Biomed Res Int. 2019;2019:3610785. doi: 10.1155/2019/3610785
- Porter B, Zauel R, Stockman H, Guldberg R, Fyhrie D. 3-D computational modeling of media flow through scaffolds in a perfusion bioreactor. J Biomech. 2005;38(3):543-549. doi: 10.1016/j.jbiomech.2004.04.011
- Cartmell SH, Porter BD, Garcia AJ, Guldberg RE. Effects of medium perfusion rate on cell-seeded three-dimensional bone constructs in vitro. Tissue Eng. 2003;9(6):1197-1203. doi: 10.1089/10763270360728107
- Raimondi MT, Boschetti F, Falcone L, et al. Mechanobiology of engineered cartilage cultured under aquantified fluid-dynamic environment. Biomechan Model Mechanobiol. 2002;1(1):14. doi: 10.1007/s10237-002-0007-y
- Van Bael S, Chai YC, Truscello S, et al. The effect of pore geometry on the in vitro biological behavior of human periosteum-derived cells seeded on selective laser-melted Ti6Al4V bone scaffolds. Acta Biomater. 2012;8(7):2824-2834. doi: 10.1016/j.actbio.2012.04.001
- 59. Rudrich U, Lasgorceix M, Champion E, et al. Pre-osteoblast cell colonization of porous silicon substituted hydroxyapatite bioceramics: Influence of microporosity and macropore design. Mater Sci Eng C Mater Biol Appl. 2019;97:510-528. doi: 10.1016/j.msec.2018.12.046