3D bioprinting of adhesive, anti-bacterial alginate/polyacrylamide-based customized boluses using digital light processing for radiotherapy applications
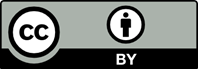
Boluses are a type of materials used to enhance skin dose during the treatment of superficial lesions. However, the current commercially available boluses cannot fully conform to irregular skin surfaces due to their uniform thickness, thereby compromising the efficacy of radiotherapy. Three-dimensional (3D) bioprinting boasts a huge potential in the creation of customized boluses, but the use of this technique is limited by shortcomings of the prevailing materials, such as their indirect printability and substance rigidity. As a potential substitute, hydrogels possessing a tensile modulus comparable to that of skin tissue are optimal candidates for customizing boluses. In this study, we developed a photocurable bioink for multifunctional boluses using digital light processing (DLP). Alginate, acrylamide, polyethylene glycol diacrylate, lithium phenyl-2,4,6-trimethylbenzoylphosphinate, and protocatechuic acid were synergistically combined to fabricate the bioink. The bolus printed using this bioink was endowed with enhanced toughness, superior adhesion, tissue equivalence, anti-dehydration and anti-bacterial properties, as well as excellent biocompatibility and radiation performance. In conclusion, the DLP-based 3D bioprinting of the proposed bioink can provide an avenue for obtaining personalized boluses in radiotherapy treatment of superficial tumors.
- Pugh R, Lloyd K, Collins M, et al. The use of 3D printing within radiation therapy to improve bolus conformity: A literature review. J Radiother Pract. 2017;16(3):319–325. doi: 10.1017/s1460396917000115
- Wang X, Wang X, Xiang Z, et al. The clinical application of 3D-printed boluses in superficial tumor radiotherapy. Front Oncol. 2021;11:698773. doi: 10.3389/fonc.2021.698773
- Lu Y, Song J, Yao X, et al. 3D printing polymer-based bolus used for radiotherapy. Int J Bioprint. 2021;7(4):414. doi: 10.18063/ijb.v7i4.414
- Aras S, Tanzer I O, Ikizceli T. Dosimetric comparison of superflab and specially prepared bolus materials used in radiotherapy practice. Eur J Breast Health. 2020;16(3): 167–170. doi: 10.5152/ejbh.2020.5041
- Robar JL, Moran K, Allan J, et al. Intrapatient study comparing 3D printed bolus versus standard vinyl gel sheet bolus for postmastectomy chest wall radiation therapy. Pract Radiat Oncol. 2018;8(4):221–229. doi: 10.1016/j.prro.2017.12.008
- Khan Y, Villarreal-Barajas JE, Udowicz M, et al. Clinical and dosimetric implications of air gaps between bolus and skin surface during radiation therapy. J Cancer Ther. 2013;04(07):1251–1255. doi: 10.4236/jct.2013.47147
- Zheng Q, Zhao L, Wang J, et al. High-strength and high-toughness sodium alginate/polyacrylamide double physically crosslinked network hydrogel with superior self-healing and self-recovery properties prepared by a one-pot method. Colloids Surf A: Physicochem Eng Asp. 2020;589:124402. doi: 10.1016/j.colsurfa.2019.124402
- Al-Sudani TA, Biasi G, Wilkinson D, et al. eXaSkin: A novel high-density bolus for 6MV X-rays radiotherapy. Phys Med. 2020;80:42–46. doi: 10.1016/j.ejmp.2020.09.002
- Obeid JP, Gutkin PM, Lewis J, et al. Volumetric modulated arc therapy and 3-dimensional printed bolus in the treatment of refractory primary cutaneous gamma delta lymphoma of the bilateral legs. Pract Radiat Oncol. 2019;9(4):220–225. doi: 10.1016/j.prro.2019.02.016
- Baltz GC, Chi PM, Wong PF, et al. Development and validation of a 3D‐printed bolus cap for total scalp irradiation. J Appl Clin Med Phys. 2019;20(3):89–96. doi: 10.1002/acm2.12552
- Zhao Y, Moran K, Yewondwossen M, et al. Clinical applications of 3-dimensional printing in radiation therapy. Med Dosim. 2017;42(2):150–155. doi: 10.1016/j.meddos.2017.03.001
- Park SY, Choi CH, Park JM, et al. A patient-specific polylactic acid bolus made by a 3D printer for breast cancer radiation therapy. PLoS One. 2016;11(12):e0168063. doi: 10.1371/journal.pone.0168063
- Park JW, Yea JW. Three-dimensional customized bolus for intensity-modulated radiotherapy in a patient with Kimura’s disease involving the auricle. Cancer Radiother. 2016;20(3):205–209. doi: 10.1016/j.canrad.2015.11.003
- Ricotti R, Ciardo D, Pansini F, et al. Dosimetric characterization of 3D printed bolus at different infill percentage for external photon beam radiotherapy. Phys Med. 2017;39:25–32. doi: 10.1016/j.ejmp.2017.06.004
- Chiu T, Tan J, Brenner M, et al. Three-dimensional printer-aided casting of soft, custom silicone boluses (SCSBs) for head and neck radiation therapy. Pract Radiat Oncol. 2018;8(3):e167–e174. doi: 10.1016/j.prro.2017.11.001
- Park JM, Son J, An HJ, et al. Bio-compatible patient-specific elastic bolus for clinical implementation. Phys Med Biol. 2019;64(10):105006. doi: 10.1088/1361-6560/ab1c93
- Kong Y, Yan T, Sun Y, et al. A dosimetric study on the use of 3D‐printed customized boluses in photon therapy: A hydrogel and silica gel study. J Appl Clin Med Phys. 2019;20(1):348–355. doi: 10.1002/acm2.12489
- Zasadzinski K, Spalek MJ, Rutkowski P. Modern dressings in prevention and therapy of acute and chronic radiation dermatitis-A literature review. Pharmaceutics. 2022;14(6). doi: 10.3390/pharmaceutics14061204
- Zhao M, Wang C, Xie J, et al. Eco-friendly and scalable synthesis of fullerenols with high free radical scavenging ability for skin radioprotection. Small. 2021;17(37):e2102035. doi: 10.1002/smll.202102035
- Fu Z, Naghieh S, Xu C, et al. Printability in extrusion bioprinting. Biofabrication. 2021;13(3). doi: 10.1088/1758-5090/abe7ab
- Ng WL, Huang X, Shkolnikov V, et al. Polyvinylpyrrolidone-based bioink: influence of bioink properties on printing performance and cell proliferation during inkjet-based bioprinting. Bio-Des Manuf. 2023;6:676–690. doi: 10.1007/s42242-023-00245-3
- Xu X, Awad A, Robles-Martinez P, et al. Vat photopolymerization 3D printing for advanced drug delivery and medical device applications. J Control Release. 2021;329:743–757. doi: 10.1016/j.jconrel.2020.10.008
- Braghiroli FL, Amaral-Labat G, Boss AFN, et al. Tannin gels and their carbon derivatives: A review. Biomolecules. 2019;9(10):587. doi: 10.3390/biom9100587
- Mortezaee K, Najafi M, Farhood B, et al. Resveratrol as an adjuvant for normal tissues protection and tumor sensitization. Curr Cancer Drug Targets. 2020;20(2):130–145. doi: 10.2174/1568009619666191019143539
- Zhou LY, Fu J, He Y. A review of 3D printing technologies for soft polymer materials. Adv Funct Mater. 2020;2000187. doi: 10.1002/adfm.202000187
- Mau R, Nazir J, John S, et al. Preliminary study on 3D printing of PEGDA hydrogels for frontal sinus implants using digital light processing (DLP). Curr Dir Biomed Eng. 2019;5(1):249–252. doi: 10.1515/cdbme-2019-0063
- Zhang B, Li S, Hingorani H, et al. Highly stretchable hydrogels for UV curing based high-resolution multimaterial 3D printing. J Mater Chem B. 2018;6(20):3246–3253. doi: 10.1039/c8tb00673c
- Sun JY, Zhao X, Illeperuma WR, et al. Highly stretchable and tough hydrogels. Nature. 2012;489(7414):133–136. doi: 10.1038/nature11409
- Huang B, Hu R, Xue Z, et al. Continuous liquid interface production of alginate/polyacrylamide hydrogels with supramolecular shape memory properties. Carbohydr Polym. 2020;231:115736. doi: 10.1016/j.carbpol.2019.115736
- Dávila JL, d’Ávila MA. Rheological evaluation of laponite/ alginate inks for 3D extrusion-based printing. Int J Adv Manuf Technol. 2018;101(1–4):675–686. doi: 10.1007/s00170-018-2876-y
- Xiang Z, Li N, Rong Y, et al. 3D-printed high-toughness double network hydrogels via digital light processing. Colloids Surf A: Physicochem Eng Asp. 2022;639:128329. doi: 10.1016/j.colsurfa.2022.128329
- Li N, Xiang Z, Rong Y, et al. 3D printing tannic acid-based gels via digital light processing. Macromol Biosci. 2022;22(4):e2100455. doi: 10.1002/mabi.202100455
- Zhu L, Rong Y, Wang Y, et al. DLP printing of tough organogels for customized wearable sensors. Eur Polym J. 2023;187:111886. doi: 10.1016/j.eurpolymj.2023.111886
- Zhu W, Ma X, Gou M, et al. 3D printing of functional biomaterials for tissue engineering. Curr Opin Biotechnol. 2016;40:103–112. doi: 10.1016/j.copbio.2016.03.014
- Axpe E, Oyen M. Applications of alginate-based bioinks in 3D bioprinting. Int J Mol Sci. 2016;17(12):1976. doi: 10.3390/ijms17121976
- Caprioli M, Roppolo I. 3D-printed self-healing hydrogels via Digital Light Processing. Nat Commun. 2021;12(1):2462. doi: 10.1038/s41467-021-22802-z
- Guo J, Suma T, Richardson JJ, et al. Modular assembly of biomaterials using polyphenols as building blocks. ACS Biomater Sci Eng. 2019;5(11):5578–5596. doi: 10.1021/ acsbiomaterials.8b01507
- Lee J, Yeo M, Kim W, et al. Development of a tannic acid cross-linking process for obtaining 3D porous cell-laden collagen structure. Int J Biol Macromol. 2018;110:497–503. doi: 10.1016/j.ijbiomac.2017.10.105
- Fischer N, Seo EJ, Efferth T. Prevention from radiation damage by natural products. Phytomedicine. 2018;47: 192–200. doi: 10.1016/j.phymed.2017.11.005
- Wang J, Liu X, Wang Y, et al. Casein micelles embedded composite organohydrogel as potential wound dressing. Int J Biol Macromol. 2022;211:678–688. doi: 10.1016/j.ijbiomac.2022.05.081
- 41. Kalra A, Lowe A, Al-Jumaily AM. Mechanical behaviour of skin: A review. J Material Sci Eng. 2016;5: 254. doi: 10.4172/2169-0022.1000254