Building a degradable scaffold with 3D printing using Masquelet technique to promote osteoblast differentiation and angiogenesis in chronic tibial osteomyelitis with bone defects
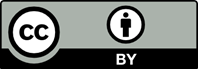
The aim of this study was to investigate the use of three-dimensional (3D) printing technology to create a biodegradable scaffold loaded with WNT5A protein and assess its impact on chronic tibial osteomyelitis with bone defects (CTO&BD), focusing on osteoblast differentiation and angiogenesis. We extracted RNA from peripheral blood of healthy individuals and CTO&BD patients for sequencing, followed by differential expression and functional enrichment analysis. Network analysis was performed to identify core genes associated with CTO&BD and construct a protein–protein interaction network. Using Masquelet technique, we fabricated a 3D-printed biodegradable scaffold (G40T60@WNT5A) and conducted various experiments, including rheological testing, printability evaluation, Fourier-transform infrared spectroscopy, X-ray diffraction, scanning electron microscopy analysis, as well as mechanical and degradation performance assessments. In in vivo experiments, we observed the formation of induced membranes in a CTO&BD rat model implanted with the scaffold. In vitro experiments involved the assessment of scaffold toxicity on rat bone marrow mesenchymal stem cells and umbilical vein endothelial cells, as well as the influence on osteoblast differentiation and angiogenesis. Molecular biology techniques were used to analyze gene and protein expression levels. We discovered for the first time that WNT5A may play a crucial role in CTO&BD. The biodegradable scaffold prepared by 3D printing (G40T60@WNT5A) exhibited excellent biocompatibility in vitro. This scaffold significantly promoted the formation of induced membranes in CTO&BD rats and further enhanced osteoblast differentiation and angiogenesis. In conclusion, this study utilized innovative 3D printing technology to fabricate the G40T60@WNT5A scaffold, confirming its potential application in the treatment of CTO&BD, particularly in promoting osteoblast differentiation and angiogenesis. This research provides new methods and theoretical support for the treatment of bone defects.
- Montanaro L, Speziale P, Campoccia D, et al. Scenery of Staphylococcus implant infections in orthopedics. Future Microbiol. 2011;6(11):1329-1349. doi: 10.2217/fmb.11.117
- Claro T, Widaa A, O’Seaghdha M, et al. Staphylococcus aureus protein A binds to osteoblasts and triggers signals that weaken bone in osteomyelitis. PLoS One. 2011;6(4):e18748. doi: 10.1371/journal.pone.0018748
- McNally MA, Ferguson JY, Lau AC, et al. Single-stage treatment of chronic osteomyelitis with a new absorbable, gentamicin-loaded, calcium sulphate/hydroxyapatite biocomposite: A prospective series of 100 cases. Bone Joint J. 2016;98-B(9):1289-1296. doi: 10.1302/0301-620X.98B9.38057
- Bury DC, Rogers TS, Dickman MM. Osteomyelitis: Diagnosis and treatment. Am Fam Physician. 2021;104(4):395-402.
- Hofmann SR, Kapplusch F, Girschick HJ, et al. Chronic recurrent multifocal osteomyelitis (CRMO): Presentation, pathogenesis, and treatment. Curr Osteoporos Rep. 2017;15(6):542-554. doi: 10.1007/s11914-017-0405-9
- Conterno LO, Turchi MD. Antibiotics for treating chronic osteomyelitis in adults. Cochrane Database Syst Rev. 2013;(9):CD004439.doi: 10.1002/14651858.CD004439.pub3
- Leerling AT, Clunie G, Koutrouba E, Dekkers OM, Appelman-Dijkstra NM, Winter EM. Diagnostic and therapeutic practices in adult chronic nonbacterial osteomyelitis (CNO). Orphanet J Rare Dis. 2023;18(1):206. doi: 10.1186/s13023-023-02831-1
- Guo B, Zhao W, Wu H, et al. Ilizarov metatarsal bone lengthening in treatment of diabetic foot ulcer complicated with chronic osteomyelitis of metatarsal head. Zhongguo Xiu Fu Chong Jian Wai Ke Za Zhi. 2020;34(8):1000-1004. doi: 10.7507/1002-1892.201911070
- Chou PH, Lin HH, Su YP, Chiang CC, Chang MC, Chen CM. Staged protocol for the treatment of chronic femoral shaft osteomyelitis with Ilizarov’s technique followed by the use of intramedullary locked nail. J Chin Med Assoc. 2017;80(6):376-382. doi: 10.1016/j.jcma.2017.01.001
- Kliushin NM, Burnashov SI, Mekki WA, Leonchuk DS, Sudnitsyn AS. Treatment of postoperative tibial chronic osteomyelitis using bone transport techniques; an observational study. J Clin Orthop Trauma. 2021;24:101652. doi: 10.1016/j.jcot.2021.101652
- Xiang F, Fan W, Liang J, Yang Y. Ilizarov technique in treating chronic osteomyelitis of the tibia with severe malformation and skin canceration: A case report. Asian J Surg. 2023;46(5):2231-2232. doi: 10.1016/j.asjsur.2022.11.117
- Sergi CM, Miller E, Demellawy DE, Shen F, Zhang M. Chronic recurrent multifocal osteomyelitis. A narrative and pictorial review. Front Immunol. 2022;13:959575. doi: 10.3389/fimmu.2022.959575
- Masquelet A, Kanakaris NK, Obert L, Stafford P, Giannoudis PV. Bone repair using the Masquelet technique. J Bone Joint Surg Am. 2019;101(11):1024-1036. doi: 10.2106/JBJS.18.00842
- Careri S, Vitiello R, Oliva MS, Ziranu A, Maccauro G, Perisano C. Masquelet technique and osteomyelitis: Innovations and literature review. Eur Rev Med Pharmacol Sci. 2019;23(2 Suppl):210-216. doi: 10.26355/eurrev_201904_17495
- Klein C, Monet M, Barbier V, et al. The Masquelet technique: Current concepts, animal models, and perspectives. J Tissue Eng Regen Med. 2020;14(9):1349-1359. doi: 10.1002/term.3097
- Khaled A, El-Gebaly O, El-Rosasy M. Masquelet-Ilizarov technique for the management of bone loss post debridement of infected tibial nonunion. Int Orthop. 2022;46(9):1937-1944. doi: 10.1007/s00264-022-05494-y
- Zhang L, Lu C, Lv Y, Wang X, Guo S, Zhang H. Three-dimensional printing-assisted Masquelet technique in the treatment of calcaneal defects. Orthop Surg. 2021;13(3):876- 883. doi: 10.1111/os.12873
- Wu Y, Shi X, Zi S, et al. The clinical application of customized 3D-printed porous tantalum scaffolds combined with Masquelet’s induced membrane technique to reconstruct infective segmental femoral defect. J Orthop Surg Res. 2022;17(1):479. doi: 10.1186/s13018-022-03371-3
- Liu X, Liu Y, Lu W, et al. Combined application of modified three-dimensional printed anatomic templates and customized cutting blocks in pelvic reconstruction after pelvic tumor resection. J Arthroplasty. 2019;34(2):338-345.e1. doi: 10.1016/j.arth.2018.10.001
- Vasiliadis AV, Koukoulias N, Katakalos K. From three-dimensional (3D)- to 6D-printing technology in orthopedics: Science fiction or scientific reality?. J Funct Biomater. 2022;13(3):101. Published 2022 Jul 21. doi: 10.3390/jfb13030101
- Li B, Zhang M, Lu Q, et al. Application and development of modern 3D printing technology in the field of orthopedics. Biomed Res Int. 2022;2022:8759060. doi: 10.1155/2022/8759060
- Xu L, Qin H, Tan J, et al. Clinical study of 3D printed personalized prosthesis in the treatment of bone defect after pelvic tumor resection. J Orthop Translat. 2021;29:163-169. doi: 10.1016/j.jot.2021.05.007
- Ganguly P, Jones E, Panagiotopoulou V, et al. Electrospun and 3D printed polymeric materials for one-stage critical-size long bone defect regeneration inspired by the Masquelet technique: Recent advances. Injury. 2022;53(Suppl 2): S2-S12. doi: 10.1016/j.injury.2022.02.036
- George A, Shah PA, Shrivastav PS. Natural biodegradable polymers based nano-formulations for drug delivery: A review. Int J Pharm. 2019;561:244-264. doi: 10.1016/j.ijpharm.2019.03.011
- Ulery BD, Nair LS, Laurencin CT. Biomedical applications of biodegradable polymers. J Polym Sci B Polym Phys. 2011;49(12):832-864. doi: 10.1002/polb.22259
- Feng Y, Zhu S, Mei D, et al. Application of 3D printing technology in bone tissue engineering: A review. Curr Drug Deliv. 2021;18(7):847-861. doi: 10.2174/1567201817999201113100322
- Gumienna M, Górna B. Antimicrobial food packaging with biodegradable polymers and bacteriocins. Molecules. 2021;26(12):3735. doi: 10.3390/molecules26123735
- Stroganov V, Al-Hussein M, Sommer JU, Janke A, Zakharchenko S, Ionov L. Reversible thermosensitive biodegradable polymeric actuators based on confined crystallization. Nano Lett. 2015;15(3):1786-1790. doi: 10.1021/nl5045023
- Liu X, Holzwarth JM, Ma PX. Functionalized synthetic biodegradable polymer scaffolds for tissue engineering. Macromol Biosci. 2012;12(7):911-919. doi: 10.1002/mabi.201100466
- Asghari F, Samiei M, Adibkia K, Akbarzadeh A, Davaran S. Biodegradable and biocompatible polymers for tissue engineering application: A review. Artif Cells Nanomed Biotechnol. 2017;45(2):185-192. doi: 10.3109/21691401.2016.1146731
- He M, Hou Y, Zhu C, et al. 3D-printing biodegradable PU/ PAAM/Gel hydrogel scaffold with high flexibility and self-adaptibility to irregular defects for nonload-bearing bone regeneration. Bioconjug Chem. 2021;32(8):1915-1925. doi: 10.1021/acs.bioconjchem.1c00322
- Chen P, Zheng L, Wang Y, et al. Desktop-stereolithography 3D printing of a radially oriented extracellular matrix/ mesenchymal stem cell exosome bioink for osteochondral defect regeneration. Theranostics. 2019;9(9):2439-2459. doi: 10.7150/thno.31017
- Duan DY, Tang J, Tian HT, Shi YY, Jia J. Adipocyte-secreted microvesicle-derived miR-148a regulates adipogenic and osteogenic differentiation by targeting Wnt5a/Ror2 pathway. Life Sci. 2021;278:119548. doi: 10.1016/j.lfs.2021.119548
- Park HW, Kim YC, Yu B, et al. Alternative Wnt signaling activates YAP/TAZ. Cell. 2015;162(4):780-794. doi: 10.1016/j.cell.2015.07.013
- Zhang X, Chang M, Wang B, Liu X, Zhang Z, Han G. YAP/ WNT5A/FZD4 axis regulates osteogenic differentiation of human periodontal ligament cells under cyclic stretch. J Periodontal Res. 2023;58(5):907-918. doi: 10.1111/jre.13143
- Chen Y, Chen Q, Zhong M, Xu C, Wu Y, Chen R. miR-637 inhibits osteogenic differentiation of human intervertebral disc cartilage endplate stem cells by targeting WNT5A. J Invest Surg. 2022;35(6):1313-1321. doi: 10.1080/08941939.2022.2050857
- Gu Q, Tian H, Zhang K, et al. Wnt5a/FZD4 mediates the mechanical stretch-induced osteogenic differentiation of bone mesenchymal stem cells. Cell Physiol Biochem. 2018;48(1):215-226. doi: 10.1159/000491721
- Chen Y, Zhang Y, Deng Q, et al. Inhibition of Wnt inhibitory factor 1 under hypoxic condition in human umbilical vein endothelial cells promoted angiogenesis in vitro. Reprod Sci. 2016;23(10):1348-1358. doi: 10.1177/1933719116638174
- Yan J, Dennin RH. High homologous nucleotide to GBV-C was amplified from DNA of MT2 and HeLa cells and PBMC of human and chimpanzee. Acta Pharmacol Sin. 2001;22(4):320-326.
- Grievink HW, Luisman T, Kluft C, Moerland M, Malone KE. Comparison of three isolation techniques for human peripheral blood mononuclear cells: Cell recovery and viability, population composition, and cell functionality. Biopreserv Biobank. 2016;14(5):410-415. doi: 10.1089/bio.2015.0104
- Hashimoto S, Noguchi E, Bando H, et al. Neoantigen prediction in human breast cancer using RNA sequencing data. Cancer Sci. 2021;112(1):465-475. doi: 10.1111/cas.14720
- Li XN, Wang ZJ, Ye CX, Zhao BC, Li ZL, Yang Y. RNA sequencing reveals the expression profiles of circRNA and indicates that circDDX17 acts as a tumor suppressor in colorectal cancer. J Exp Clin Cancer Res. 2018;37(1):325. doi: 10.1186/s13046-018-1006-x
- Zhang Z, Jia H, Gu T, et al. RNA sequencing and bioinformatics analysis of the long noncoding RNA-mRNA network in colorectal cancer. J Cell Biochem. 2018;119(12):9957-9966. doi: 10.1002/jcb.27319
- Wan J, Liu B. Construction of lncRNA-related ceRNA regulatory network in diabetic subdermal endothelial cells. Bioengineered. 2021;12(1):2592-2602. doi: 10.1080/21655979.2021.1936892
- Zhou Y, Shi W, Zhao D, Xiao S, Wang K, Wang J. Identification of immune-associated genes in diagnosing aortic valve calcification with metabolic syndrome by integrated bioinformatics analysis and machine learning. Front Immunol. 2022;13:937886.
doi: 10.3389/fimmu.2022.937886
- Yang C, Delcher C, Shenkman E, Ranka S. Machine learning approaches for predicting high cost high need patient expenditures in health care. Biomed Eng Online. 2018;17(Suppl 1):131. doi: 10.1186/s12938-018-0568-3
- Ellis K, Kerr J, Godbole S, Lanckriet G, Wing D, Marshall S. A random forest classifier for the prediction of energy expenditure and type of physical activity from wrist and hip accelerometers. Physiol Meas. 2014;35(11):2191-2203. doi: 10.1088/0967-3334/35/11/2191
- Zhang M, Zhu K, Pu H, et al. An immune-related signature predicts survival in patients with lung adenocarcinoma. Front Oncol. 2019;9:1314. doi: 10.3389/fonc.2019.01314
- Alderden J, Pepper GA, Wilson A, et al. Predicting pressure injury in critical care patients: A machine-learning model. Am J Crit Care. 2018;27(6):461-468. doi: 10.4037/ajcc2018525
- Li J, Liu C, Chen Y, et al. Tumor characterization in breast cancer identifies immune-relevant gene signatures associated with prognosis. Front Genet. 2019;10:1119. doi: 10.3389/fgene.2019.01119
- Zhu M, Ye M, Wang J, Ye L, Jin M. Construction of potential miRNA-mRNA regulatory network in COPD plasma by bioinformatics analysis. Int J Chron Obstruct Pulmon Dis. 2020;15:2135-2145. doi: 10.2147/COPD.S255262
- Jeong JE, Park SY, Shin JY, et al. 3D printing of bone-mimetic scaffold composed of gelatin/β-tri-calcium phosphate for bone tissue engineering. Macromol Biosci. 2020;20(12):e2000256. doi: 10.1002/mabi.202000256
- Jin J, Ou Q, Wang Z, et al. BMSC-derived extracellular vesicles intervened the pathogenic changes of scleroderma in mice through miRNAs. Stem Cell Res Ther. 2021;12(1):327. doi: 10.1186/s13287-021-02400-y
- Lou Z, Peng Z, Wang B, Li X, Li X, Zhang X. miR-142-5p promotes the osteoclast differentiation of bone marrow-derived macrophages via PTEN/PI3K/AKT/FoxO1 pathway. J Bone Miner Metab. 2019;37(5):815-824. doi: 10.1007/s00774-019-00997-y
- Li R, Zhou C, Chen J, et al. Synergistic osteogenic and angiogenic effects of KP and QK peptides incorporated with an injectable and self-healing hydrogel for efficient bone regeneration. Bioact Mater. 2022;18:267-283. doi: 10.1016/j.bioactmat.2022.02.011
- Messner K, Wei Y, Andersson B, Gillquist J, Räsänen T. Rat model of Achilles tendon disorder. A pilot study. Cells Tissues Organs. 1999;165(1):30-39. doi: 10.1159/000016671
- Wu J, Pan X, Fu H, et al. Effect of curcumin on glycerol-induced acute kidney injury in rats. Sci Rep. 2017;7(1):10114. doi: 10.1038/s41598-017-10693-4
- Wang C, Liang C, Ma J, et al. Co-exposure to fluoride and sulfur dioxide on histological alteration and DNA damage in rat brain. J Biochem Mol Toxicol. 2018;32(2). doi: 10.1002/jbt.22023
- Xu Y, Zhou J, Liu C, et al. Understanding the role of tissue-specific decellularized spinal cord matrix hydrogel for neural stem/progenitor cell microenvironment reconstruction and spinal cord injury. Biomaterials. 2021;268:120596. doi: 10.1016/j.biomaterials.2020.120596
- Zhou W, Zhou Y, Chen X, et al. Pancreatic cancer-targeting exosomes for enhancing immunotherapy and reprogramming tumor microenvironment. Biomaterials. 2021;268:120546. doi: 10.1016/j.biomaterials.2020.120546
- Wu Q, Yi X. Down-regulation of long noncoding RNA MALAT1 protects hippocampal neurons against excessive autophagy and apoptosis via the PI3K/Akt signaling pathway in rats with epilepsy. J Mol Neurosci. 2018;65(2):234-245. doi: 10.1007/s12031-018-1093-3
- Shen J, Sun Y, Liu X, et al. EGFL6 regulates angiogenesis and osteogenesis in distraction osteogenesis via Wnt/β-catenin signaling. Stem Cell Res Ther. 2021;12(1):415. doi: 10.1186/s13287-021-02487-3
- He X, Liu W, Liu Y, et al. Nano artificial periosteum PLGA/ MgO/Quercetin accelerates repair of bone defects through promoting osteogenic - angiogenic coupling effect via Wnt/ β-catenin pathway. Mater Today Bio. 2022;16:100348. doi: 10.1016/j.mtbio.2022.100348
- Wu M, Chen F, Liu H, et al. Bioinspired sandwich-like hybrid surface functionalized scaffold capable of regulating osteogenesis, angiogenesis, and osteoclastogenesis for robust bone regeneration. Mater Today Bio. 2022;17:100458. doi: 10.1016/j.mtbio.2022.100458
- Baschant U, Rauner M, Balaian E, et al. Wnt5a is a key target for the pro-osteogenic effects of iron chelation on osteoblast progenitors. Haematologica. 2016;101(12):1499-1507. doi: 10.3324/haematol.2016.144808
- Wan X, Guan S, Hou Y, et al. FOSL2 promotes VEGF-independent angiogenesis by transcriptionnally activating Wnt5a in breast cancer-associated fibroblasts. Theranostics. 2021;11(10):4975-4991. doi: 10.7150/thno.55074
- Clarke B. Normal bone anatomy and physiology. Clin J Am Soc Nephrol. 2008;3(Suppl 3):S131-S139. doi: 10.2215/CJN.04151206
- Yang T, Zhang J, Cao Y, et al. Wnt5a/Ror2 mediates temporomandibular joint subchondral bone remodeling. J Dent Res. 2015;94(6):803-812. doi: 10.1177/0022034515576051
- Brun J, Fromigué O, Dieudonné FX, et al. The LIM-only protein FHL2 controls mesenchymal cell osteogenic differentiation and bone formation through Wnt5a and Wnt10b. Bone. 2013;53(1):6-12. doi: 10.1016/j.bone.2012.11.020
- Uehara S, Udagawa N, Mukai H, et al. Protein kinase N3 promotes bone resorption by osteoclasts in response to Wnt5a-Ror2 signaling. Sci Signal. 2017;10(494):eaan0023. doi: 10.1126/scisignal.aan0023
- Uehara S, Udagawa N, Kobayashi Y. Regulation of osteoclast function via Rho-Pkn3-c-Src pathways. J Oral Biosci. 2019;61(3):135-140. doi: 10.1016/j.job.2019.07.002
- Wang Z, Wang Y, Yan J, et al. Pharmaceutical electrospinning and 3D printing scaffold design for bone regeneration. Adv Drug Deliv Rev. 2021;174:504-534. doi: 10.1016/j.addr.2021.05.007
- Wang S, Zhao S, Yu J, Gu Z, Zhang Y. Advances in translational 3D printing for cartilage, bone, and osteochondral tissue engineering. Small. 2022;18(36):e2201869. doi: 10.1002/smll.202201869
- Zhang L, Yang G, Johnson BN, Jia X. Three-dimensional (3D) printed scaffold and material selection for bone repair. Acta Biomater. 2019;84:16-33. doi: 10.1016/j.actbio.2018.11.039
- Xu J, Ji J, Jiao J, et al. 3D Printing for bone-cartilage interface regeneration. Front Bioeng Biotechnol. 2022;10: 828921. doi: 10.3389/fbioe.2022.828921
- Chang PC, Lin ZJ, Luo HT, et al. Degradable RGD-functionalized 3D-printed scaffold promotes osteogenesis. J Dent Res. 2021;100(10):1109-1117. doi: 10.1177/00220345211024634
- Wu N, Liu J, Ma W, et al. Degradable calcium deficient hydroxyapatite/poly(lactic-glycolic acid copolymer) bilayer scaffold through integral molding 3D printing for bone defect repair. Biofabrication. 2021;13(2). doi: 10.1088/1758-5090/abcb48
- Yang Y, Chu L, Yang S, et al. Dual-functional 3D-printed composite scaffold for inhibiting bacterial infection and promoting bone regeneration in infected bone defect models. Acta Biomater. 2018;79:265-275. doi: 10.1016/j.actbio.2018.08.015
- Wang H, Fan HX, Cheng HZ, Li R, Geng HX. Shanghai Kou Qiang Yi Xue. 2021;30(1):28-32.
- 80. Deng Y, Jiang C, Li C, et al. 3D printed scaffolds of calcium silicate-doped β-TCP synergize with co-cultured endothelial and stromal cells to promote vascularization and bone formation. Sci Rep. 2017;7(1):5588. doi: 10.1038/s41598-017-05196-1