Microfluidic fiber spinning for 3D bioprinting: Harnessing microchannels to build macrotissues
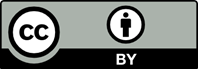
Microfluidics is rapidly revolutionizing the scientific panorama, providing unmatched high-throughput platforms that find application in numerous areas of physics, chemistry, biology, and materials science. Recently, microfluidic chips have been proposed, in combination with bioactive materials, as promising tools for spinning cell-laden fibers with on-demand characteristics. However, cells encapsulated in filaments produced via microfluidic spinning technology are confined in a quasi-three-dimensional (3D) environment that fails to replicate the intricate 3D architecture of biological tissues. Thanks to the recent synergistic combination of microfluidic devices with 3D bioprinting technologies that enable the production of sophisticated microfibers serving as the backbone of 3D structures, a new age of tissue engineering is emerging. This review looks at how combining microfluidics with 3D printing is contributing to the biofabrication of relevant human substitutes and implants. This paper also describes the whole manufacturing process from the production of the microfluidic tool to the printing of tissue models, focusing on cutting-edge fabrication technologies and emphasizing the most noticeable achievements for microfluidic spinning technology. A theoretical insight for thixotropic hydrogels is also proposed to predict the fiber size and shear stress developing within microfluidic channels. The potential of using microfluidic chips as bio-printheads for multi-material and multi-cellular bioprinting is discussed, highlighting the challenges that microfluidic bioprinting still faces in advancing the field of biofabrication for tissue engineering and regenerative medicine purposes.
- Whitesides GM. The origins and the future of microfluidics. Nature. 2006;442(7101):368-373. doi: 10.1038/nature05058
- Convery N, Gadegaard N. 30 Years of microfluidics. Micro Nano Eng. 2019;2(November 2018):76-91. doi: 10.1016/j.mne.2019.01.003
- Guimarães CF, Gasperini L, Marques AP, Reis RL. 3D flow-focusing microfluidic biofabrication: One-chip-fits-all hydrogel fiber architectures. Appl Mater Today. 2021;23:101013. doi: 10.1016/j.apmt.2021.101013
- Abrishamkar A, Nilghaz A, Saadatmand M, Naeimirad M, deMello AJ. Microfluidic-assisted fiber production: Potentials, limitations, and prospects. Biomicrofluidics. 2022;16(6):1-32. doi: 10.1063/5.0129108
- Pati F, Gantelius J, Svahn HA. 3D bioprinting of tissue/organ models. Angew Chemie Int Ed. 2016;55(15):4650-4665. doi: 10.1002/anie.201505062
- van der Heide D, Cidonio G, Stoddart MJ, D'Este M. 3D printing of inorganic-biopolymer composites for bone regeneration. Biofabrication. 2022;14(4):042003. doi: 10.1088/1758-5090/ac8cb2
- Scognamiglio C, Soloperto A, Ruocco G, Cidonio G. Bioprinting stem cells: Building physiological tissues one cell at a time. Am J Physiol - Cell Physiol. 2020;319(3):C465-C480. doi: 10.1152/ajpcell.00124.2020
- Cidonio G, Glinka M, Kim Y-H, Dawson JI, Oreffo ROC. Nanocomposite clay-based bioinks for skeletal tissue engineering. Methods Mol Biol. 2021;2147:63-72. doi: 10.1007/978-1-0716-0611-7_6
- Memic A, Navaei A, Mirani B, et al. Bioprinting technologies for disease modeling. Biotechnol Lett. 2017;39(9):1279-1290. doi: 10.1007/s10529-017-2360-z
- Iafrate L, Benedetti MC, Donsante S, et al. Modelling skeletal pain harnessing tissue engineering. Vitr Model. 2022;1(4-5):289-307. doi: 10.1007/s44164-022-00028-7
- Marcotulli M, Tirelli MC, Volpi M, Jaroszewicz J. Microfluidic 3D printing of emulsion ink for engineering porous functionally graded materials. Adv Mater Technol. 2022;2201244:1-12. doi: 10.1002/admt.202201244
- Davoodi E, Sarikhani E, Montazerian H, et al. Extrusion and microfluidic-based bioprinting to fabricate biomimetic tissues and organs. Adv Mater Technol. 2020;5(8): 1901044. doi: 10.1002/admt.201901044
- Sackmann EK, Fulton AL, Beebe DJ. The present and future role of microfluidics in biomedical research. Nature. 2014;507(7491):181-189. doi: 10.1038/nature13118
- Chen L, Yang C, Xiao Y, et al. Millifluidics, microfluidics, and nanofluidics: manipulating fluids at varying length scales. Mater Today Nano. 2021;16:100136. doi: 10.1016/j.mtnano.2021.100136
- Bezrukov AN, Galyametdinov YG. Evaluation of diffusion coefficients of small ions in a microfluidic channel. 2021;85(8):889-893. doi: 10.3103/S1062873821080049
- Böke JS, Kraus D, Henkel T. Microfluidic network simulations enable on-demand prediction of control parameters for operating lab-on-a-chip-devices. Processes. 2021;9(8):1320. doi: 10.3390/pr9081320
- Qin D, Xia Y, Rogers JA, Jackman RJ, Zhao X-M, Whitesides GM. Microfabrication, microstructures and microsystems. In: Manz A, Becker H, eds. Microsystem Technology in Chemistry and Life Sciences. 1998;Vol 194 Springer-Verlag. 1-20. doi: 10.1007/3-540-69544-3_1
- Xia Y, Whitesides GM. Soft lithography. Angew Chemie - Int Ed. 1998;37(5):550-575. doi: 10.1002/(sici)1521-3773(19980316)37:5<550::aid-anie550>3.3.co;2-7
- O’Neill PF, Ben Azouz A, Vázquez M, et al. Advances in three-dimensional rapid prototyping of microfluidic devices for biological applications. Biomicrofluidics. 2014;8(5):052112. doi: 10.1063/1.4898632
- Qin D, Xia Y, Whitesides GM. Soft lithography for micro- and nanoscale patterning. Nat Protoc. 2010;5(3): 491-502. doi: 10.1038/nprot.2009.234
- Bhattacharjee N, Urrios A, Kang S, Folch A. The upcoming 3D-printing revolution in microfluidics. Lab Chip. 2016;16(10):1720-1742. doi: 10.1039/c6lc00163g
- Thaweskulchai T, Schulte A. A low-cost 3-in-1 3d printer as a tool for the fabrication of flow-through channels of microfluidic systems. Micromachines. 2021;12(8):947. doi: 10.3390/mi12080947
- Zhu F, Friedrich T, Nugegoda D, Kaslin J, Wlodkowic D. Assessment of the biocompatibility of three-dimensional-printed polymers using multispecies toxicity tests. Biomicrofluidics. 2015;9(6):61103. doi: 10.1063/1.4939031
- MacDonald NP, Zhu F, Hall CJ, et al. Assessment of biocompatibility of 3D printed photopolymers using zebrafish embryo toxicity assays. Lab Chip. 2016;16(2): 291-297. doi: 10.1039/c5lc01374g
- Joseph Rey JRH, Chen Q, Maalihan RD, et al. 3D printing of biomedically relevant polymer materials and biocompatibility. MRS Commun. 2021;11(2):197-212. doi: 10.1557/s43579-021-00038-8
- de Almeida Monteiro Melo Ferraz M, Nagashima JB, Venzac B, Le Gac S, Songsasen N. 3D printed mold leachates in PDMS microfluidic devices. Sci Rep. 2020;10(1):994. doi: 10.1038/s41598-020-57816-y
- Zhou Z, Chen D, Wang X, Jiang J. Milling positive master for polydimethylsiloxane microfluidic devices: The microfabrication and roughness issues. Micromachines. 2017;8(10). doi: 10.3390/mi8100287
- Guckenberger DJ, De Groot TE, Wan AMD, Beebea DJ, Young EWK. Micromilling: A method for ultra-rapid prototyping of plastic microfluidic devices. Lab Chip. 2015;15(11): 2364-2378. doi: 10.1039/c5lc00234f
- Ku X, Zhang Z, Liu X, Chen L. Low-cost rapid prototyping of glass microfluidic devices using a micromilling technique. Microfluid Nanofluidics. 2018;22(8):1-8. doi: 10.1007/s10404-018-2104-y
- Hossain MM, Rahman T. Low cost micro milling machine for prototyping plastic microfluidic devices. Proceedings. 2018;2(13):707. doi: 10.3390/proceedings2130707
- Virumbrales-Muñoz M, Livingston MK, Farooqui M, Skala MC, Beebe DJ, Ayuso JM. Development of a microfluidic array to study drug response in breast cancer. Molecules. 2019;24(23):1-12. doi: 10.3390/molecules24234385
- Costantini M, Idaszek J, Szöke K, et al. 3D bioprinting of BM-MSCs-loaded ECM biomimetic hydrogels for in vitro neocartilage formation. Biofabrication. 2016;8(3):035002. doi: 10.1088/1758-5090/8/3/035002
- Costantini M, Testa S, Mozetic P, et al. Microfluidic-enhanced 3D bioprinting of aligned myoblast-laden hydrogels leads to functionally organized myofibers in vitro and in vivo. Biomaterials. 2017;131:98-110. doi: 10.1016/j.biomaterials.2017.03.026
- Idaszek J, Costantini M, Karlsen TA, et al. 3D bioprinting of hydrogel constructs with cell and material gradients for the regeneration of full-thickness chondral defect using a microfluidic printing head. Biofabrication. 2019;11(4):044101. doi: 10.1088/1758-5090/ab2622
- Behroodi E, Latifi H, Bagheri Z, Ermis E, Roshani S, Moghaddam MS. A combined 3D printing/CNC micro-milling method to fabricate a large-scale microfluidic device with the small size 3D architectures: An application for tumor spheroid production. Sci Rep. 2020;10(1):1-14. doi: 10.1038/s41598-020-79015-5
- Kyle S, Jessop ZM, Al-Sabah A, Whitaker IS. Printability of candidate biomaterials for extrusion based 3D printing: State-of-the-art. Adv Healthc Mater. 2017;6(16):1-16. doi: 10.1002/adhm.201700264
- Waheed S, Cabot JM, Macdonald NP, et al. 3D printed microfluidic devices: Enablers and barriers. Lab Chip. 2016;16(11):1993-2013. doi: 10.1039/c6lc00284f
- Macdonald NP, Cabot JM, Smejkal P, Guijt RM, Paull B, Breadmore MC. Comparing microfluidic performance of three-dimensional (3D) printing platforms. Anal Chem. 2017;89(7):3858-3866. doi: 10.1021/acs.analchem.7b00136
- Ho CMB, Ng SH, Li KHH, Yoon Y-J. 3D printed microfluidics for biological applications. Lab Chip. 2015;15(18): 3627-3637. doi: 10.1039/c5lc00685f
- Zeraatkar M, de Tullio MD, Pricci A, Pignatelli F. Exploiting limitations of fused deposition modeling to enhance mixing in 3D printed microfluidic devices. Rapid Prototyp J. 2021;27(10):1850-1859. doi: 10.1108/RPJ-03-2021-0051
- Quero RF, Domingos Da Silveira G, Fracassi Da Silva JA, de Jesus DP. Understanding and improving FDM 3D printing to fabricate high-resolution and optically transparent microfluidic devices. Lab Chip. 2021;21(19):3715-3729. doi: 10.1039/d1lc00518a
- Ballacchino G, Weaver E, Mathew E, et al. Manufacturing of 3d-printed microfluidic devices for the synthesis of drug-loaded liposomal formulations. Int J Mol Sci. 2021;22(15):8064. doi: 10.3390/ijms22158064
- Mehta V, Vilikkathala Sudhakaran S, Rath SN. Facile route for 3D printing of transparent PETg-based hybrid biomicrofluidic devices promoting cell adhesion. ACS Biomater Sci Eng. 2021;7(8):3947-3963. doi: 10.1021/acsbiomaterials.1c00633
- Mader M, Rein C, Konrat E, et al. 2021, Fused deposition modeling of microfluidic chips in transparent polystyrene. Micromachines, 12(11):1348. doi: 10.3390/mi12111348
- Kara A, Vassiliadou A, Ongoren B, et al. Engineering 3d printed microfluidic chips for the fabrication of nanomedicines. Pharmaceutics. 2021;13(12):1-17. doi: 10.3390/pharmaceutics13122134
- Ching T, Li Y, Karyappa R, Ohno A. Fabrication of integrated microfluidic devices by direct ink writing (DIW) 3D printing. Sensors Actuators, B Chem. 2019;297(December 2018):126609. doi: 10.1016/j.snb.2019.05.086
- Karyappa R, Ching T, Hashimoto M. Embedded ink writing (EIW) of polysiloxane inks. ACS Appl Mater Interfaces. 2020;12(20):23565-23575. doi: 10.1021/acsami.0c03011
- Childs EH, Latchman AV, Lamont AC, Hubbard J. Additive assembly for polyjet-based multi-material 3D printed microfluidics. J Microelectromech Syst. 2020;29(5): 1094-1096. doi: 10.1109/JMEMS.2020.3003858
- Walczak R, Adamski K. Inkjet 3D printing of microfluidic structures - On the selection of the printer towards printing your own microfluidic chips. J. Micromech Microeng. 2015;25(8):085013. doi: 10.1088/0960-1317/25/8/085013
- Hwang Y, Paydar OH, Candler RN. 3D printed molds for non-planar PDMS microfluidic channels. Sensors Actuators, A Phys. 2015;226:137-142. doi: 10.1016/j.sna.2015.02.028
- King PH, Jones G, Morgan H, de Planquea MRR, Zauner K-P. Interdroplet bilayer arrays in millifluidic droplet traps from 3D-printed moulds. Lab Chip. 2014;14(14):722-729. doi: 10.1039/c3lc51072g
- Glick CC, Srimongkol MT, Schwartz AJ, et al. Rapid assembly of multilayer microfluidic structures via 3D-printed transfer molding and bonding. Microsyst Nanoeng. 2016;2(1):16063. doi: 10.1038/micronano.2016.63
- Vijayan S, Parthiban P, Hashimoto M. Evaluation of lateral and vertical dimensions of micromolds fabricated by a polyjetTM printer. Micromachines. 2021;12(3):1-13. doi: 10.3390/mi12030302
- Anderson KB, Lockwood SY, Martin RS, et al. A 3D printed fluidic device that enables integrated features. Anal Chem. 2013;85(12):5622-5626. doi: 10.1021/ac4009594
- Chen S, He Z, Choi S, Novosselov IV. Characterization of inkjet-printed digital microfluidics devices. Sensors. 2021;21(9):3064. doi: 10.3390/s21093064
- Sochol RD, Sweet E, Glick CC, et al. 3D printed microfluidic circuitry via multijet-based additive manufacturing. Lab Chip. 2016;16(4):668-678. doi: 10.1039/c5lc01389e
- Layani M, Wang X, Magdassi S. Novel materials for 3D printing by photopolymerization. Adv Mater. 2018;30(41):1706344. doi: 10.1002/adma.201706344
- Lu Y, Mapili G, Suhali G, Chen S, Roy K. A digital micro-mirror device-based system for the microfabrication of complex, spatially patterned tissue. J Biomed Mater Res. 2006;77(2):396-405. doi: 10.1002/jbm.a.30601
- Männel MJ, Selzer L, Bernhardt R, et al. Optimizing process parameters in commercial micro- stereolithography for forming emulsions and polymer microparticles in nonplanar microfluidic devices. Adv Mater Technol. 2019;1800408: 1-10. doi: 10.1002/admt.201800408
- Qin D, Xia Y, Whitesides GM. Rapid prototyping of complex structures with feature sizes larger than 20 μm. Adv Mater. 1996;8(11):917-919. doi: 10.1002/adma.19960081110
- Bertsch A, Heimgartner S, Cousseau P, Renauda P. Static micromixers based on large-scale industrial mixer geometry. Lab Chip. 2001;1(1):56-60. doi: 10.1039/b103848f
- Morimoto Y, Kiyosawa M, Takeuchi S. Three-dimensional printed microfluidic modules for design changeable coaxial microfluidic devices. Sens Actuators B Chem. 2018;274(July):491-500. doi: 10.1016/j.snb.2018.07.151
- Costantini M, Testa S, Fornetti E, et al. Biofabricating murine and human myo-substitutes for rapid volumetric muscle loss restoration. EMBO Mol Med. 2021;13(3):1-17. doi: 10.15252/emmm.202012778
- Li W, Yao K, Tian L, Xue C, Zhang X, Gao X. 3D printing of heterogeneous microfibers with multi-hollow structure via microfluidic spinning. J Tissue Eng Regen Med. 2022;16(10):913-922. doi: 10.1002/term.3339
- Maruo S, Nakamura O, Kawata S. Three-dimensional microfabrication with two-photon-absorbed photopolymerization. Opt Lett. 1997;22(2):132-134. doi: 10.1364/OL.22.000132
- Faraji Rad Z, Prewett PD, Davies GJ. High-resolution two-photon polymerization: the most versatile technique for the fabrication of microneedle arrays. Microsyst Nanoeng. 2021;7(1):71. doi: 10.1038/s41378-021-00298-3
- Fornell A, Söderbäck P, Liu Z, De Albuquerque Moreira M, Tenje M. Fabrication of silicon microfluidic chips for acoustic particle focusing using direct laser writing. Micromachines. 2020;11(2):113. doi: 10.3390/mi11020113
- Oliveira B, Veigas B, Fernandes AR, et al. Fast prototyping microfluidics: Integrating droplet digital lamp for absolute quantification of cancer biomarkers. Sensors. 2020;20(6):1624. doi: 10.3390/s20061624
- Yong J, Zhan Z, Singh SC, Chen F, Guo C. Microfluidic channels fabrication based on underwater superpolymphobic microgrooves produced by femtosecond laser direct writing. ACS Appl Polym Mater. 2019;1(11):2819-2825. doi: 10.1021/acsapm.9b00269
- Zyla G, Kovalev A, Esen C, Ostendorf A, Gorb S. Two-photon polymerization as a potential manufacturing tool for biomimetic engineering of complex structures found in nature. J Opt Microsyst. 2022;2(03):1-12. doi: 10.1117/1.JOM.2.3.031203
- Lölsberg J, Linkhorst J, Cinar A, Jans A, Kuehne AJC, Wessling M. 3D nanofabrication inside rapid prototyped microfluidic channels showcased by wet-spinning of single micrometre fibres. Lab Chip. 2018;18(9):1341-1348. doi: 10.1039/c7lc01366c
- Wang Y, Kankala RK, Zhu K, Wang S-B, Zhang YS, Chen A-Zg. Coaxial extrusion of tubular tissue constructs using a gelatin/GelMA blend bioink. ACS Biomater Sci Eng. 2019;5(10):5514-5524. doi: 10.1021/acsbiomaterials.9b00926
- Shao L, Gao Q, Zhao H, et al. Fiber-based mini tissue with morphology-controllable GelMA microfibers. Small. 2018;14(44):1-8. doi: 10.1002/smll.201802187
- Yu Y, Wei W, Wang Y, Xu C, Guo Y, Qin J. Simple spinning of heterogeneous hollow microfibers on chip. Adv Mater. 2016;28:6649-6655. doi: 10.1002/adma.201601504
- Hu M, Kurisawa M, Deng R, et al. Cell immobilization in gelatin – hydroxyphenylpropionic acid hydrogel fibers. Biomaterials. 2009;30(21):3523-3531. doi: 10.1016/j.biomaterials.2009.03.004
- Yang Y, Sun J, Liu X, et al. Wet-spinning fabrication of shear-patterned alginate hydrogel microfibers and the guidance of cell alignment. Regen Biomater. 2017;4(5):299-307. doi: 10.1093/rb/rbx017
- Zhang X, Weng L, Liu Q, Li D, Deng B. Facile fabrication and characterization on alginate microfibres with grooved structure via microfluidic spinning. R Soc Open Sci. 2019;6(5):181928. doi: 10.1098/rsos.181928
- Rinoldi C, Costantini M, Kijeńska-Gawrońska E, et al. Tendon tissue engineering:Effects of mechanical and biochemical stimulation on stem cell alignment on cell-laden hydrogel yarns. Adv Healthc Mater. 2019; 8(7):1-10. doi: 10.1002/adhm.201801218
- Xu Z, Wu M, Ye Q, Chen D, Liu K, Bai H. Spinning from nature: Engineered preparation and application of high-performance bio-based fibers. Engineering. 2022;14:100-112. doi: 10.1016/j.eng.2021.06.030
- Colosi C, Costantini M, Barbetta A, et al. Microfluidic bioprinting of heterogeneous 3d tissue constructs. Methods Mol Biol. 2017;1612:369-380. doi: 10.1007/978-1-4939-7021-6_26
- Wang G, Jia L, Han F, et al. Microfluidics-based fabrication of cell-laden hydrogel microfibers for potential applications in tissue engineering. Molecules. 2019;24(8). doi: 10.3390/molecules24081633
- Wu F, Ju X jie, He X heng, et al. A novel synthetic microfiber with controllable size for cell encapsulation and culture. J Mater Chem B. 2016;4:2455-2465. doi: 10.1039/c6tb00209a
- Onoe H, Okitsu T, Itou A, et al. Metre-long cell-laden microfibres exhibit tissue morphologies and functions. Nat Mater. 2013;12(6):584-590. doi: 10.1038/nmat3606
- Hu M, Deng R, Schumacher KM, et al. Hydrodynamic spinning of hydrogel fibers. Biomaterials. 2010;31(5):863–869. doi: 10.1016/j.biomaterials.2009.10.002
- Bonhomme O, Leng J, Colin A. Microfluidic wet-spinning of alginate microfibers: A theoretical analysis of fiber formation. Soft Matter. 2012;8(41):10641-10649. doi: 10.1039/c2sm25552a
- Kurdzinski ME, Gol Berrak, Hee AC, et al. Dynamics of high viscosity contrast confluent microfluidic flows. Sci Rep. 2017;7(1):1-11. doi: 10.1038/s41598-017-06260-6
- Zaeri A, Zgeib R, Cao K, Zhang F, Chang RC. Numerical analysis on the effects of microfluidic-based bioprinting parameters on the microfiber geometrical outcomes. Sci Rep. 2022;12(1):1-16. doi: 10.1038/s41598-022-07392-0
- Zhao M, Liu H, Zhang X, Wang H, Taoab T, Qin J. A flexible microfluidic strategy to generate grooved microfibers for guiding cell alignment. Biomater Sci. 2021;9(14):4880-4890. doi: 10.1039/D1BM00549A
- Cai J, Ye D, Wu Y, Fan L, Yu H. Injectable alginate fibrous hydrogel with a three-dimensional network structure fabricated by microfluidic spinning. Compos Commun. 2019;15(April):1-5. doi: 10.1016/j.coco.2019.06.004
- Ebrahimi M, Ostrovidov S, Bae H, Kim SB, Bae H, Khademhosseini A. Enhanced skeletal muscle formation on microfluidic spun gelatin methacryloyl (GelMA) fibres using surface patterning and agrin treatment. J Tissue Eng Regenrative Med. 2019;12:2151-2163. doi: 10.1002/term.2738
- Daniele MA, Radom K, Ligler FS. Microfluidic fabrication of multiaxial microvessels via hydrodynamic shaping. RSC Adv. 2014;4:23440-23446. doi: 10.1039/c4ra03667k
- Williams CG, Malik AN, Kim TK, Manson PN, Elisseeff JH. Variable cytocompatibility of six cell lines with photoinitiators used for polymerizing hydrogels and cell encapsulation. Biomaterials. 2005;26(11):1211-1218. doi: 10.1016/j.biomaterials.2004.04.024
- Lim KS, Klotz BJ, Lindberg GCJ, et al. Visible light cross-linking of gelatin hydrogels offers an enhanced cell microenvironment with improved light penetration depth. Macromol Biosci. 2019;19(6):1-14. doi: 10.1002/mabi.201900098
- He X, Wang W, Deng K, et al. Microfluidic fabrication of chitosan microfibers with controllable internals from tubular to peapodlike structures. RSC Adv. 2015;5: 928-936. doi: 10.1039/c4ra10696b
- Cui T, Yu J, Li Q, et al. Large-scale fabrication of robust artificial skins from a biodegradable sealant-loaded nanofiber scaffold to skin tissue via microfluidic blow-spinning. Adv Mater. 2020;2000982(32):1-11. doi: 10.1002/adma.202000982
- Jia J, Richards DJ, Pollard S, et al. Engineering alginate as bioink for bioprinting. Acta Biomater. 2014;10(10): 4323-4331. doi: 10.1016/j.actbio.2014.06.034
- Hernández-González AC, Téllez-Jurado L, Rodríguez- Lorenzo LM. Alginate hydrogels for bone tissue engineering, from injectables to bioprinting: A review. Carbohydr Polym. 2020;229(October 2019):115514. doi: 10.1016/j.carbpol.2019.115514
- Costantini M, Colosi C, Świe¸szkowski W, Barbetta A. Co-axial wet-spinning in 3D bioprinting: State of the art and future perspective of microfluidic integration. Biofabrication. 2019;11(1):012001. doi: 10.1088/1758-5090/aae605
- Du XY, Li Q, Wu G, Chen S. Multifunctionalmicro/ nanoscale fibers based on microfluidic spinning technology. Adv Mater. 2019;31(52):1-38. doi: 10.1002/adma.201903733
- Cidonio G, Costantini M, Pierini F, Scognamiglio C, Agarwald T, Barbetta A. 3D printing of biphasic inks: beyond single-scale architectural control. J Mater Chem C. 2021;9(37):12489-12508. doi: 10.1039/D1TC02117F
- Sivashanmugam A, Arun Kumar R, Vishnu Priya M, Nair SV, Jayakumar R. An overview of injectable polymeric hydrogels for tissue engineering. Eur Polym J. 2015;72: 543-565. doi: 10.1016/j.eurpolymj.2015.05.014
- Chopin-Doroteo M, Mandujano-Tinoco EA, Krötzsch E. Tailoring of the rheological properties of bioinks to improve bioprinting and bioassembly for tissue replacement. Biochim Biophys Acta - Gen Subj. 2021;1865(2):129782. doi: 10.1016/j.bbagen.2020.129782
- Cooke ME, Rosenzweig DH. The rheology of direct and suspended extrusion bioprinting. APL Bioeng. 2021;5(1):011502. doi: 10.1063/5.0031475
- Townsend JM, Beck EC, Gehrke SH, Berkland CJ, Detamore MS. Flow behavior prior to crosslinking: The need for precursor rheology for placement of hydrogels in medical applications and for 3D bioprinting. Prog Polym Sci. 2019;91:126-140. doi: 10.1016/j.progpolymsci.2019.01.003
- Rudolph N, Osswald TA. Polymer Rheology: Fundamentals and Applications, Carl Hanser Verlag GmbH & Company KG; 2014. https://books.google.pl/books?id=11ctBQAAQBAJ
- Bird RB, Armstrong RC, Hassager O. Dynamics of Polymeric Liquids, Volume 1: Fluid Mechanics, Wiley; 1987.
- Doi M, Edwards SF. The Theory of Polymer Dynamics, Clarendon Press; 1986. https://books.google.pl/books?id=sAFQzQEACAAJ
- Maxwell JC. On the dynamical theory of gases. Philos Trans R Soc London. 1867;157:49-88. http://www.jstor.org/stable/108968
- Bird RB, Armstrong RC, Hassager O. Dynamics of Polymeric Liquids, Volume 2: Kinetic Theory, Wiley; 1987.
- Pourmasoumi P, Moghaddam A, Mahand SN, et al. A review on the recent progress, opportunities, and challenges of 4D printing and bioprinting in regenerative medicine. J Biomater Sci Polym Ed. 2023;34(1):108-146. doi: 10.1080/09205063.2022.2110480
- Paxton N, Smolan W, Böck T, Melchels F, Groll J, Jungst T. Proposal to assess printability of bioinks for extrusion-based bioprinting and evaluation of rheological properties governing bioprintability. Biofabrication. 2017;9(4):044107. doi: 10.1088/1758-5090/aa8dd8
- Gregory T, Benhal P, Scutte A, et al. Rheological characterization of cell-laden alginate-gelatin hydrogels for 3D biofabrication. J Mech Behav Biomed Mater. 2022;136(September):105474. doi: 10.1016/j.jmbbm.2022.105474
- Cooke ME, Rosenzweig DH. The rheology of direct and suspended extrusion bioprinting. APL Bioeng. 2021;5(1):011502. doi: 10.1063/5.0031475
- Filippi M, Buchner T, Yasa O, Weirich S, Katzschmann RK. Microfluidic tissue engineering and bio-actuation. Adv Mater. 2022;34(23):2108427. doi: 10.1002/adma.202108427
- Cheng Y, Yu Y, Fu F, et al. Controlled fabrication of bioactive microfibers for creating tissue constructs using microfluidic techniques. ACS Appl Mater Interfaces. 2016;8(2): 1080-1086. doi: 10.1021/acsami.5b11445
- Boyd DA, Shields AR, Howell PB, Ligler FS. Design and fabrication of uniquely shaped thiol–ene microfibers using a two-stage hydrodynamic focusing design. Lab Chip. 2013;13:3105-3110. doi: 10.1039/c3lc50413a
- Kobayashi A, Yamakoshi K, Yajima Y, Utoh R, Yamada M, Seki M. Preparation of stripe-patterned heterogeneous hydrogel sheets using micro fluidic devices for high-density coculture of hepatocytes and fibroblasts. J Biosci Bioeng. 2013;116(6):761-767. doi: 10.1016/j.jbiosc.2013.05.034
- Gursoy A, Iranshahi K, Wei K, et al. Facile fabrication of microfluidic chips for 3D hydrodynamic focusing and wet spinning of polymeric fibers. Polymers (Basel). 2020;12(3): 1-13. doi: 10.3390/polym12030633
- Attalla R, Ling C, Selvaganapathy P. Fabrication and characterization of gels with integrated channels using 3D printing with microfluidic nozzle for tissue engineering applications. Biomed Microdevices. 2016;18(1):17. doi: 10.1007/s10544-016-0042-6
- Wei D, Sun J, Bolderson J, et al. Continuous fabrication and assembly of spatial cell-laden fibers for a tissue-like construct via a photolithographic-based microfluidic chip. ACS Appl Mater Interfaces. 2017;9:14606-14617. doi: 10.1021/acsami.7b00078
- Pi Q, Maharjan S, Yan X, et al. Digitally tunable microfluidic bioprinting of multilayered cannular tissues. Adv Mater. 2018;30(43):1-10. doi: 10.1002/adma.201706913
- Xiao Y, Yang C, Zhai X, et al. Bioinspired tough and strong fibers with hierarchical core–shell structure. Adv Mater Interfaces. 2023;10(2):2201962. doi: 10.1002/admi.202201962
- Colosi C, Shin SR, Manoharan V, et al. Microfluidic bioprinting of heterogeneous 3D tissue constructs using low-viscosity bioink. Adv Mater, 2016;28(4):677-684. doi: 10.1002/adma.201503310
- Feng F, He J, Li J, Mao M, Li D. Multicomponent bioprinting of heterogeneous hydrogel constructs based on microfluidic printheads. Int JBioprint. 2019;5(2):39-48. doi: 10.18063/ijb.v5i2.202
- Miri AK, Nieto D, Iglesias L, et al. Microfluidics-enabled multimaterial maskless stereolithographic bioprinting. Adv Mater. 2018;30(27):1-9. doi: 10.1002/adma.201800242
- Hassan I, Selvaganapathy PR. Microfluidic printheads for highly switchable multimaterial 3D printing of soft materials. Adv Mater Technol. 2022;2101709:1-10. doi: 10.1002/admt.202101709
- Hardin JO, Ober TJ, Valentine AD, Lewis JA. Microfluidic printheads for multimaterial 3D printing of viscoelastic inks. Adv Mater, 2015;27(21):3279-3284. doi: 10.1002/adma.201500222
- Zhang L, Fu L, Zhang X, Chen L, Cai Q, Yang X. Hierarchical and heterogeneous hydrogel system as a promising strategy for diversified interfacial tissue regeneration. Biomater Sci. 2021;9(5):1547-1573. doi: 10.1039/D0BM01595D
- Chai N, Zhang J, Zhang Q, et al. Construction of 3D printed constructs based on microfluidic microgel for bone regeneration. Compos Part B Eng. 2021;223(June):109100. doi: 10.1016/j.compositesb.2021.109100
- Kamperman T, Henke S, van den Berg A, et al. Single cell microgel based modular bioinks for uncoupled cellular micro- and macroenvironments. Adv Healthc Mater. 2017;6(3):1600913. doi: 10.1002/adhm.201600913
- Kim B, Kim I, Choi W, Kim SW, Kim J, Lim G. Fabrication of cell-encapsulated alginate microfiber scaffold using microfluidic channel. J Manuf Sci Eng. 2008;130(2):0210161-0210166. doi: 10.1115/1.2898576
- Yao K, Li W, Li K, et al. Simple fabrication of multicomponent heterogeneous fibers for cell co-culture via microfluidic spinning. Macromol Biosci. 2020;20(3):1900395. doi: 10.1002/mabi.201900395
- Oh J, Kim K, Won SW, et al. Microfluidic fabrication of cell adhesive chitosan microtubes. Biomed Microdevices. 2013;15(3):465-472. doi: 10.1007/s10544-013-9746-z
- Novosel EC, Kleinhans C, Kluger PJ. Vascularization is the key challenge in tissue engineering. Adv Drug Deliv Rev. 2011;63(4-5):300-311. doi: 10.1016/j.addr.2011.03.004
- Cheng Y, Zheng F, Lu J, et al. Bioinspired multicompartmental microfibers from microfluidics. Adv Mater, 2014;26(30):5184-5190. doi: 10.1002/adma.201400798
- Cheng J, Jun Y, Qin J, Lee S-H. Electrospinning versus microfluidic spinning of functional fibers for biomedical applications. Biomaterials. 2017;114:121-143. doi: 10.1016/j.biomaterials.2016.10.040
- Jun Y, Kang E, Chae S, Lee S-H. Microfluidic spinning of micro- and nano-scale fibers for tissue engineering. Lab Chip. 2014;14(13):2145-2160. doi: 10.1039/c3lc51414e
- Kang E, Choi YY, Chae SK, Moon J-H, Chang J-Y, Lee S-H. Microfluidic spinning of flat alginate fibers with grooves for cell-aligning scaffolds. Adv Mater. 2012;24(31):4271-4277. doi: 10.1002/adma.201201232
- Celikkin N, Presutti D, Maiullari F, et al. Combining rotary wet-spinning biofabrication and electro-mechanical stimulation for the in vitro production of functional myo-substitutes. Biofabrication. 2023;15(4):045012. doi: 10.1088/1758-5090/ace934
- Cidonio G, Glinka M, Dawson JI, Oreffo ROC. The cell in the ink: Improving biofabrication by printing stem cells for skeletal regenerative medicine. Biomaterials. 2019;209(March):10-24. doi: 10.1016/j.biomaterials.2019.04.009
- Jia L, Han F, Yang H, et al. Microfluidic fabrication of biomimetic helical hydrogel microfibers for blood-vessel-on-a-chip applications. Adv Healthc Mater. 2019;8(13):1-10. doi: 10.1002/adhm.201900435
- van Genderen AM, Valverde MG, Capendale PE, et al. Co-axial printing of convoluted proximal tubule for kidney disease modeling. Biofabrication. 2022;14(4):044102. doi: 10.1088/1758-5090/ac7895
- Xu H, Casillas J, Krishnamoorthy S, Xu C. Effects of Irgacure 2959 and lithium physical properties, and microstructure in 3D bioprinting of vascular-like constructs. Biomed Mater. 2020;15(5):055021. doi: 10.1088/1748-605X/ab954e
- Wang M, Li W, Mille LS, et al. Digital light processing based bioprinting with composable gradients. Adv Mater. 2022;34(1):2107038. doi: 10.1002/adma.202107038
- Hogan J, Sun Y, Yu K, et al. Modeling the printability of photocuring and strength adjustable hydrogel bioink during projection-based 3D bioprinting. J Manuf Process. 2021;69:583-592. doi: 10.1088/1758-5090/aba413
- Xie X, Wu S, Mou S, Guo N, Wang Z, Sun J. Microtissue-based bioink as a chondrocyte microshelter for DLP bioprinting. Adv Healthc Mater. 2022;11(22):2201877. doi: 10.1002/adhm.202201877
- Guifang G, Tomo Y, Karen H, Dai G, Cui X. Inkjet-bioprinted acrylated peptides and PEG hydrogel with human mesenchymal stem cells promote robust bone and cartilage formation with minimal printhead clogging. Biotechnol J. 2015;10(10):1568-1577. doi: 10.1002/biot.201400635
- Park JA, Yoon S, Kwon J, et al. Freeform micropatterning of living cells into cell culture medium using direct inkjet printing. Sci Rep. 2017;7(1):14610. doi: 10.1038/s41598-017-14726-w
- Solis LH, Ayala Y, Portillo S, Varela-Ramirez A, Aguilera R, Boland T. Thermal inkjet bioprinting triggers the activation of the VEGF pathway in human microvascular endothelial cells in vitro. Biofabrication. 2019;11(4):045005. doi: 10.1088/1758-5090/ab25f9
- Saadi MASR, Maguire A, Pottackal NT, et al. Direct ink writing: A 3D printing technology for diverse materials. Adv Mater. 2022;34(28):1-57. doi: 10.1002/adma.202108855
- Jung Y, Shafranek RT, Tsui JH, Walcott J, Nelson A, Kim D-H. 3D bioprinting of mechanically tuned bioinks derived from cardiac decellularized extracellular matrix. Acta Biomater. 2021;119: 75-88. doi: 10.1016/j.actbio.2020.11.006
- Connell CDO, Konate S, Onofrillo C, et al. Bioprinting free-form co-axial bioprinting of a gelatin methacryloyl bio-ink by direct in situ photo-crosslinking during extrusion. Bioprinting. 2020;19(April):e00087. doi: 10.1016/j.bprint.2020.e00087
- Bertlein S, Brown G, Lim KS, et al. Thiol–ene clickable gelatin: A platform bioink for multiple 3D biofabrication technologies. Adv Mater. 2017;29(44):1-6. doi: 10.1002/adma.201703404
- Ooi HW, Mota C, ten Cate AT, Calore A, Moroni L, Baker MB. Thiol–ene alginate hydrogels as versatile bioinks for bioprinting. Biomacromolecules. 2018;19(8):3390-3400. doi: 10.1021/acs.biomac.8b00696
- Bhattacharyya A, Janarthanan G, Kim T, et al. Modulation of bioactive calcium phosphate micro/nanoparticle size and shape during in situ synthesis of photo-crosslinkable gelatin methacryloyl based nanocomposite hydrogels for 3D bioprinting and tissue engineering. Biomater Res. 2022;26(1):54. doi: 10.1186/s40824-022-00301-6
- Rastin H, Ormsby RT, Atkins GJ, Losic D. 3D bioprinting of methylcellulose/gelatin-methacryloyl (MC/GelMA) bioink with high shape integrity. ACS Appl Bio Mater. 2020;3(3):1815-1826. doi: 10.1021/acsabm.0c00169
- Bertassoni LE, Cardoso JC, Manoharan V, et al. Direct-write bioprinting of cell-laden methacrylated gelatin hydrogels. Biofabrication. 2014;6(2):024105. doi: 10.1088/1758-5082/6/2/024105
- Romanazzo S, Molley TG, Nemec S, et al. Synthetic bone-like structures through omnidirectional ceramic bioprinting in cell suspensions. Adv Funct Mater. 2021;2008216:1-12. doi: 10.1002/adfm.202008216
- Spencer AR, Sani ES, Soucy JR, et al. Bioprinting of a cell-laden conductive hydrogel composite. ACS Appl Mater Interfaces. 2019;11:30518-30533. doi: 10.1021/acsami.9b07353
- Dikyol C, Altunbek M, Koc B. Embedded multimaterial bioprinting platform for biofabrication of biomimetic vascular structures. J Mater Res. 2021;36(19):3851-3864. doi: 10.1557/s43578-021-00254-x
- Chalard A, Mauduit M, Souleille S, Joseph P, Malaquin L, Fitremann J. 3D printing of a biocompatible low molecular weight supramolecular hydrogel by dimethylsulfoxide water solvent exchange. Addit Manuf. 2020;33(February):101162. doi: 10.1016/j.addma.2020.101162
- Colosi C, Costantini M, Latini R, et al. Rapid prototyping of chitosan-coated alginate scaffolds through the use of a 3D fiber deposition technique. J Mater Chem B. 2014;2(39): 6779-6791. doi: 10.1039/c4tb00732h
- Duchi S, Onofrillo C, O’Connell CD, et al. Handheld co-axial bioprinting: Application to in situ surgical cartilage repair. Sci Rep. 2017;7(1):5837. doi: 10.1038/s41598-017-05699-x
- Hakimi N, Cheng R, Leng L, et al. Handheld skin printer: In situ formation of planar biomaterials and tissues. Lab Chip. 2018;18(10):1440-1451. doi: 10.1039/c7lc01236e
- Ying G, Manríquez J, Wu D, et al. An open-source handheld extruder loaded with pore-forming bioink for in situ wound dressing. Mater Today Bio. 2020;8(July):100074. doi: 10.1016/j.mtbio.2020.100074
- Pagan E, Stefanek E, Seyfoori A, et al. A handheld bioprinter for multi-material printing of complex constructs. Biofabrication. 2023;15(3):035012. doi: 10.1088/1758-5090/acc42c
- Salaris F, Colosi C, Brighi C, et al. 3D bioprinted human cortical neural constructs derived from induced pluripotent stem cells. J Clin Med. 2019;8(1595):1-13. doi: 10.3390%2Fjcm8101595
- Yu Y, Shang L, Guo J, Wang J, Zhao Y. Design of capillary microfluidics for spinning cell-laden microfibers. Nat Protoc. 2018;13(11):2557-2579. doi: 10.1038/s41596-018-0051-4
- Gao Q, He Y, Fu J, Liu A, Ma L. Coaxial nozzle-assisted 3D bioprinting with built-in microchannels for nutrients delivery. Biomaterials. 2015;61:203-215. doi: 10.1016/j.biomaterials.2015.05.031
- Gao G, Park JY, Kim BS, Jang J, Cho D-W. Coaxial cell printing of freestanding, perfusable, and functional in vitro vascular models for recapitulation of native vascular endothelium pathophysiology. Adv Healthc Mater. 2018;7(23):1801102. doi: 10.1002/adhm.201801102
- Wu Z, Cai H, Ao Z, Xu J, Heaps S, Guo F. Microfluidic printing of tunable hollow microfibers for vascular tissue engineering. Adv Mater Technol. 2021;6(8):1-9. doi: 10.1002/admt.202000683
- Gao Q, Liu Z, Lin Z, et al. 3D bioprinting of vessel-like structures with multi-level fluidic channels 3D bioprinting of vessel-like structures with multi-level fluidic channels. ACS Biomater Sci Eng. 2017;3(3):399-408. doi: 10.1021/acsbiomaterials.6b00643
- Wang D, Maharjan S, Kuang X, et al. Microfluidic bioprinting of tough hydrogel-based vascular conduits for functional blood vessels. Sci Adv. 2022;8(43):1-18. doi: 10.1126/sciadv.abq6900
- Silva CA, Cortés-Rodriguez CJ, Hazur J, Reakasame S, Boccaccini AR. Rationaldesign of a triple-layered coaxial extruder system: In silico and in vitro evaluations directed towards optimizing cell viability. Int JBioprint. 2020;6(4): 96-105. doi: 10.18063/ijb.v6i4.282
- Zuo Y, He X, Yang Y, et al. Microfluidic-based generation of functional microfibers for biomimetic complex tissue construction. Acta Biomater. 2016;38:153-162. doi: 10.1016/j.actbio.2016.04.036
- Li S, Liu Y, Li Y, Sun Y, Hu Q. A novel method for fabricating engineered structures with branched micro-channel using hollow hydrogel fibers. Biomicrofluidics. 2016;10(6):064104. doi: 10.1063/1.4967456
- Puertas-Bartolomé M, Włodarczyk-Biegun MK, del Campo A, Vázquez-Lasa B, Román JS. 3D printing of a reactive hydrogel bio-ink using a static mixing tool. Polymers. 2020;12(9):1986. doi: 10.3390/polym12091986
- Fernando C, Johana E, Quevedo-moreno DA, et al. High-throughput and continuous chaotic bioprinting of spatially controlled bacterial microcosms. ACS Biomater Sci Eng. 2021;7:2192-2197. doi: 10.1021/acsbiomaterials.0c01646
- Chávez-Madero C, de León-Derby MD, Samandari M, et al. Using chaotic advection for facile high-throughput fabrication of ordered multilayer micro- and nanostructures: Continuous chaotic printing. Biofabrication. 2020;12(3):35023. doi: 10.1088/1758-5090/ab84cc
- Samandari M, Alipanah F, Majidzadeh-A K, Alvarez MM, Santiago GT-de, Tamayol A. Controlling cellular organization in bioprinting through designed 3D microcompartmentalization. Appl Phys Rev. 2021;8(2):1-14. doi: 10.1063/5.0040732
- Guimarães CF, Gasperini L, Ribeiro RS, Carvalho AF, Marquesab AP, Reis RL. High-throughput fabrication of cell-laden 3D biomaterial gradients. Mater Horizons. 2020;7(9):2414-2421. doi: 10.1039/D0MH00818D
- Lavrentieva A, Fleischhammer T, Enders A, Pirmahboub H, Bahnemann J, Pepelanova I. Fabrication of stiffness gradients of GelMA hydrogels using a 3D printed micromixer. Macromol Biosci. 2020;20(7):e2000107. doi: 10.1002/mabi.202000107
- Kuzucu M, Vera G, Beaumont M, et al. Extrusion-based 3D bioprinting of gradients of stiff ness, cell density, and immobilized peptide using thermogelling hydrogels. ACS Biomater Sci Eng. 2021;7:2192-2197. doi: 10.1021/acsbiomaterials.1c00183
- Attalla R, Puersten E, Jain N, Selvaganapathy PR. 3D bioprinting of heterogeneous bi- and tri-layered hollow channels within gel scaffolds using scalable multi-axial microfluidic extrusion nozzle. Biofabrication. 2019;11(1):015012. doi: 10.1088/1758-5090/aaf7c7
- Beyer ST, Bsoul A, Ahmadi A. 3D alginate constructs for tissue engineering printed using a coaxial flow focusing microfluidic device. In: 2013 Transducers & Eurosensors XXVII: The 17th International Conference on Solid-State Sensors, Actuators and Microsystems (TRANSDUCERS & EUROSENSORS XXVII), IEEE. 2013;1206-1209. doi: 10.1109/TRANSDUCERS.2013.6626990
- Abelseth E, Abelseth L, De La Vega L, Beyer ST, Wadsworth SJ, Willerth SM. 3D printing of neural tissues derived from human induced pluripotent stem cells using a fibrin-based bioink. ACS Biomater Sci Eng. 2019;5(1):234-243. doi: 10.1021/acsbiomaterials.8b01235
- Mirani B, Stefanek E, Godau B, Hossein Dabiri SM, Akbari M. Microfluidic 3D printing of a photo-cross-linkable bioink using insights from computational modeling. ACS Biomater Sci Eng. 2021;7(7):3269-3280. doi: 10.1021/acsbiomaterials.1c00084
- Akbari M, Khademhosseini A. Tissue bioprinting for biology and medicine. Cell. 2022;185(15):2644-2648. doi: 10.1016/j.cell.2022.06.015
- Beyer ST, Mohamed T, Walus K. A microfluidics based 3D bioprinter with on-the-fly multimaterial switching capability. 17th Int Conf Miniaturized Syst Chem Life Sci MicroTAS. 2013;1(October):176-178.
- Perez MR, Sharma R, Masri NZ. 3D bioprinting mesenchymal stem cell-derived neural tissues using a fibrin-based bioink. Biomolecules. 2021;11(1250):1-15. doi: 10.3390/biom11081250
- Lee C, Abelseth E, de la Vega L, Willerth SM. Bioprinting a novel glioblastoma tumor model using a fibrin-based bioink for drug screening. Mater Today Chem. 2019;12:78-84. doi: 10.1016/j.mtchem.2018.12.005
- Smits IPM, Blaschuk OW, Willerth SM. Novel N-cadherin antagonist causes glioblastoma cell death in a 3D bioprinted co-culture model. Biochem Biophys Res Commun. 2020;529(2):162-168. doi: 10.1016/j.bbrc.2020.06.001
- Sharma R, Smits IPM, De La Vega L, Lee C, Willerth SM. 3D bioprinting pluripotent stem cell derived neural tissues using a novel fibrin bioink containing drug releasing microspheres. Front Bioeng Biotechnol. 2020;8(February):1-12. doi: 10.3389/fbioe.2020.00057
- Sharma R, Kirsch R, Valente KP, Perez MR, Willerth SM. Physical and mechanical characterization of fibrin-based bioprinted constructs containing drug-releasing microspheres for neural tissue engineering applications. Processes. 2021;9(7):1205. doi: 10.3390/pr9071205
- Dickman CTD, Russo V, Thain K, et al. Functional characterization of 3D contractile smooth muscle tissues generated using a unique microfluidic 3D bioprinting technology. FASEB J, 2020;34(1):1652-1664. doi: 10.1096/fj.201901063RR
- Addario G, Djudjaj S, Farè S, Boor P, Moroni L, Mota C. Microfluidic bioprinting towards a renal in vitro model. Bioprinting. 2020;20(July):e00108. doi: 10.1016/j.bprint.2020.e00108
- Serex L, Bertsch A, Renaud P. Microfluidics: A new layer of control for extrusion-based 3D printing. Micromachines. 2018;9(2):86. doi: 10.3390/mi9020086
- Serex L, Sharma K, Rizov V, Bertsch A, McKinney JD, Renaud P. Microfluidic-assisted bioprinting of tissues and organoids at high cell concentrations. Biofabrication. 2021;13(12). doi: 10.1088/1758-5090/abca80
- Lee KG, Park KJ, Seok S, et al. 3D printed modules for integrated microfluidic devices. RSC Adv. 2014;4(62): 32876-32880. doi: 10.1039/c4ra05072j
- Bhargava KC, Thompson B, Malmstadt N. Discrete elements for 3D microfluidics. Proc Natl Acad Sci USA. 2014;111(42):15013-15018. doi: 10.1073/pnas.1414764111
- Vittayarukskul K, Lee AP. A truly Lego®-like modular microfluidics platform. J MicromechMicroeng. 2017; 27(3):35004. doi: 10.1088/1361-6439/aa53ed
- Yuen PK. A reconfigurable stick-n-play modular microfluidic system using magnetic interconnects. Lab Chip. 2016;16(19):3700-3707. doi: 10.1039/c6lc00741d
- Chae S, Kang E, Khademhosseini A, Lee SH. Micro/ nanometer-scale fiber with highly ordered structures by mimicking the spinning process of silkworm. Adv Mater. 2013;25(22):3071-3078. doi: 10.1002/adma.201300837
- Ahn SY, Mun CH, Lee SH. Microfluidic spinning of fibrous alginate carrier having highly enhanced drug loading capability and delayed release profile. RSC Adv. 2015;5:15172-15181. doi: 10.1039/c4ra11438h
- Sugimoto M, Kitagawa Y, Yamada M, Yajima Y, Utoha R, Sekia Minoru. Micropassage-embedding composite hydrogel fibers enable quantitative evaluation of cancer cell invasion under 3D coculture conditions. Lab Chip. 2018;18(9):1378-1387. doi: 10.1039/C7LC01280B
- Zhou X, Nowicki M, Sun H, et al. 3D bioprinting-tunable small-diameter blood vessels with biomimetic biphasic cell layers. ACS Appl Mater Interfaces. 2020;12(41): 45904-45915. doi: 10.1021/acsami.0c14871