Exploring the potential of supramolecular hydrogels as advanced bioinks for bioprinting and biomedical applications
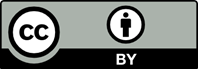
Supramolecular hydrogels have emerged as versatile bioinks in tissue engineering, providing a promising avenue for constructing intricate and functional biological structures. This paper explores the significance of employing supramolecular hydrogels as advanced bioinks for three-dimensional bioprinting and various biomedical applications. Supramolecular hydrogels possess distinct and tunable characteristics attributed to the dynamic nature of supramolecular host–guest interactions alongside interactions based on DNA and peptides, which increases their significance in tissue engineering. These interactions are essential for enhancing the mechanical properties, injectability, printability, post-printing stability, and biocompatibility of hydrogels. Gelation kinetics and rheological properties can also be manipulated to suit specific printing techniques. Furthermore, these supramolecular interactions facilitate the incorporation of bioactive molecules to regulate cellular behavior and tissue development. These diverse interactions observed in supramolecular hydrogels underscore their ability to emulate the dynamic and responsive nature of the cell’s extracellular matrix, which fosters cell growth, adherence, and differentiation. This review specifically highlights the cucurbit[n]uril and cyclodextrin-based host–guest supramolecular hydrogels, as well as peptide and DNA-based supramolecular structures as advanced bioinks and brief examples of their applications in various biomedical fields. These advanced bioinks would drive the development of intricate tissue constructs with enhanced biomimicry and therapeutic potential in regenerative medicine.
- Xiang T, Guo Q, Jia L, et al. Multifunctional hydrogels for the healing of diabetic wounds. Adv Healthc Mater. 2023:2301885. doi: 10.1002/adhm.202301885
- Gelmi A, Schutt CE. Stimuli‐responsive biomaterials: scaffolds for stem cell control. Adv Healthc Mater. 2021;10(1):2001125. doi: 10.1002/adhm.202001125
- Lim J, Lin Q, Xue K, Loh X. Recent advances in supramolecular hydrogels for biomedical applications. Mater Today Adv. 2019;3:100021. doi: 10.1016/j.mtadv.2019.100021
- Karoyo AH, Wilson LD. Physicochemical properties and the gelation process of supramolecular hydrogels: a review. Gels. 2017;3(1):1. doi: 10.3390/gels3010001
- Skopinska-Wisniewska J, De la Flor S, Kozlowska J. From supramolecular hydrogels to multifunctional carriers for biologically active substances. Int J Mol Sci. 2021;22(14):7402. doi: 10.3390/ijms22147402
- Saunders L, Ma PX. Self‐healing supramolecular hydrogels for tissue engineering applications. Macromol Biosci. 2019;19(1):1800313. doi: 10.1002/mabi.201800313
- Dong R, Zhou Y, Huang X, Zhu X, Lu Y, Shen J. Functional supramolecular polymers for biomedical applications. Adv Mater. 2015;27(3):498-526. doi: 10.1002/adma.201402975
- Dong R, Pang Y, Su Y, Zhu X. Supramolecular hydrogels: synthesis, properties and their biomedical applications. Biomater Sci. 2015;3(7):937-954. doi: 10.1039/C4BM00448E
- Malík M, Velechovský J, Tlustoš P. Natural pentacyclic triterpenoid acids potentially useful as biocompatible nanocarriers. Fitoterapia. 2021;151:104845. doi: 10.1016/j.fitote.2021.104845
- Xu C, Yu B, Qi Y, Zhao N, Xu FJ. Versatile types of cyclodextrin‐based nucleic acid delivery systems. Adv Healthc Mater. 2021;10(1):2001183. doi: 10.1002/adhm.202001183
- Cai Y, Zheng C, Xiong F, et al. Recent progress in the design and application of supramolecular peptide hydrogels in cancer therapy. Adv Healthc Mater. 2021;10(1):2001239. doi: 10.1002/adhm.202001239
- Ayoubi‐Joshaghani MH, Seidi K, Azizi M, et al. Potential applications of advanced nano/hydrogels in biomedicine: static, dynamic, multi‐stage, and bioinspired. Adv Funct Mater. 2020;30(45):2004098. doi: 10.1002/adfm.202004098
- Hu J-H, Huang Y, Redshaw C, Tao Z, Xiao X. Cucurbit [n] uril-based supramolecular hydrogels: synthesis, properties and applications. Coord Chem Rev. 2023;489:215194. doi: 10.1016/j.ccr.2023.215194
- Raymond DM, Abraham BL, Fujita T, et al. Low-molecular-weight supramolecular hydrogels for sustained and localized in vivo drug delivery. ACS Appl Bio Mater. 2019;2(5): 2116-2124. doi: 10.1021/acsabm.9b00125
- Hao Z, Li H, Wang Y, et al. Supramolecular peptide nanofiber hydrogels for bone tissue engineering: from multihierarchical fabrications to comprehensive applications. Adv Sci. 2022;9(11):2103820. doi: 10.1002/advs.202103820
- Dong RJ, Pang Y, Su Y, Zhu XY. Supramolecular hydrogels: synthesis, properties and their biomedical applications. Biomater Sci. 2015;3(7):937-954. doi: 10.1039/c4bm00448e
- Wang X, Wang J, Yang YY, Yang F, Wu DC. Fabrication of multi-stimuli responsive supramolecular hydrogels based on host-guest inclusion complexation of a tadpole-shaped cyclodextrin derivative with the azobenzene dimer. Polym Chem-Uk. 2017;8(26):3901-3909. doi: 10.1039/c7py00698e
- Chen Y, Pang XH, Dong CM. Dual stimuli-responsive supramolecular polypeptide-based hydrogel and reverse micellar hydrogel mediated by host-guest chemistry. Adv Funct Mater. 2010;20(4):579-586. doi: 10.1002/adfm.200901400
- Ghosh G, Barman R, Sarkar J, Ghosh S. pH-responsive biocompatible supramolecular peptide hydrogel. J Phys Chem B. 2019;123(27):5909-5915. doi: 10.1021/acs.jpcb.9b02999
- Wang Q, Zhang YY, Dai XY, Shi XH, Liu WG. A high strength pH responsive supramolecular copolymer hydrogel. Sci China Technol Sc. 2017;60(1):78-83. doi: 10.1007/s11431-016-0698-0
- Zhu CN, Zheng SY, Qiu HN, et al. Plastic-like supramolecular hydrogels with polyelectrolyte/surfactant complexes as physical crosslinks. Macromolecules. 2021;54(17): 8052-8066. doi: 10.1021/acs.macromol.1c00835
- Bernhard S, Tibbitt MW. Supramolecular engineering of hydrogels for drug delivery. Adv Drug Deliv Rev. 2021;171:240-256. doi: 10.1016/j.addr.2021.02.002
- Raina N, Pahwa R, Bhattacharya J, et al. Drug delivery strategies and biomedical significance of hydrogels: translational considerations. Pharmaceutics. 2022;14(3):574. doi: 10.1002/btm2.10147
- O’Connor JP, Kanjilal D, Teitelbaum M, Lin SS, Cottrell JA. Zinc as a therapeutic agent in bone regeneration. Materials. 2020;13(10). doi: 10.3390/ma13102211
- Chandramohan Y, Jeganathan K, Sivanesan S, et al. Assessment of human ovarian follicular fluid derived mesenchymal stem cells in chitosan/PCL/Zn scaffold for bone tissue regeneration. Life Sci. 2021;264. doi: 10.1016/j.lfs.2020.118502
- Wahid F, Zhou Y-N, Wang H-S, Wan T, Zhong C, Chu L-Q. Injectable self-healing carboxymethyl chitosan-zinc supramolecular hydrogels and their antibacterial activity. Int J Biol Macromol. 2018;114:1233-1239. doi: 10.1002/adhm.201900847
- Xu J, Feng Q, Lin S, et al. Injectable stem cell-laden supramolecular hydrogels enhance in situ osteochondral regeneration via the sustained co-delivery of hydrophilic and hydrophobic chondrogenic molecules. Biomaterials. 2019;210:51-61. doi: 10.1016/j.biomaterials.2019.04.031
- Grosskopf AK, Roth GA, Smith AA, Gale EC, Hernandez HL, Appel EA. Injectable supramolecular polymer– nanoparticle hydrogels enhance human mesenchymal stem cell delivery. Bioeng Transl Med. 2020;5(1):e10147. doi: 10.1186/s40824-018-0122-1
- Aguado BA, Mulyasasmita W, Su J, Lampe KJ, Heilshorn SC. Improving viability of stem cells during syringe needle flow through the design of hydrogel cell carriers. Tissue Eng Part A. 2012;18(7-8):806-815. doi: 10.1021/acs.chemrev.0c00015
- Lopez Hernandez H, Grosskopf AK, Stapleton LM, Agmon G, Appel EA. Non‐Newtonian polymer–nanoparticle hydrogels enhance cell viability during injection. Macromol Biosci. 2019;19(1):1800275. doi: 10.1016/j.polymer.2018.08.029
- Mol EA, Lei Z, Roefs MT, et al. Injectable supramolecular ureidopyrimidinone hydrogels provide sustained release of extracellular vesicle therapeutics. Adv Healthc Mater. 2019;8(20):1900847. doi: 10.1002/anie.201804400
- Meis CM, Grosskopf AK, Correa S, Appel EA. Injectable supramolecular polymer-nanoparticle hydrogels for cell and drug delivery applications. J Vis Exp: JoVE. 2021;(168). doi: 10.1002/anie.201804400
- Zhao Y, Song S, Ren X, Zhang J, Lin Q, Zhao Y. Supramolecular adhesive hydrogels for tissue engineering applications. Chem Rev. 2022;122(6):5604-5640. doi: 10.1021/acs.chemrev.1c00815
- Morwood AJ, El-Karim IA, Clarke SA, Lundy FT. The role of extracellular matrix (ECM) adhesion motifs in functionalised hydrogels. Molecules. 2023;28(12):4616. doi: 10.3390/molecules28124616
- Zhang ZP, Hu J, Ma PX. Nanofiber-based delivery of bioactive agents and stem cells to bone sites. Adv Drug Deliv Rev. 2012;64(12):1129-1141. doi: 10.1016/j.addr.2012.04.008
- Luo ZL, Zhang SG. Designer nanomaterials using chiral self-assembling peptide systems and their emerging benefit for society. Chem Soc Rev. 2012;41(13):4736-4754. doi: 10.1039/c2cs15360b
- Yang YL, Khoe U, Wang XM, Horii A, Yokoi H, Zhang SG. Designer self-assembling peptide nanomaterials. Nano Today. 2009;4(2):193-210. doi: 10.1016/j.nantod.2009.02.009
- Shi L, Ding P, Wang Y, Zhang Y, Ossipov D, Hilborn J. Self‐healing polymeric hydrogel formed by metal–ligand coordination assembly: design, fabrication, and biomedical applications. Macromol Rapid Commun. 2019;40(7):1800837. doi: 10.1002/marc.201800837
- Gopinathan J, Noh I. Recent trends in bioinks for 3D printing. Biomater Res. 2018;22(1):11. doi: 10.1186/s40824-018-0122-1
- Lee SC, Gillispie G, Prim P, Lee SJ. Physical and chemical factors influencing the printability of hydrogel-based extrusion bioinks. Chem Rev. 2020;120(19):10834-10886. doi: 10.1021/acs.chemrev.0c00015
- Li H, Wang H, Zhang D, Xu Z, Liu W. A highly tough and stiff supramolecular polymer double network hydrogel. Polymer. 2018;153:193-200. doi: 10.1016/j.polymer.2018.08.029
- Wang Z, Ren Y, Zhu Y, et al. A rapidly self‐healing host– guest supramolecular hydrogel with high mechanical strength and excellent biocompatibility. Angew Chem Int Ed. 2018;57(29):9008-9012. doi: 10.1002/anie.201804400
- Wu Q, Wei J, Xu B, et al. A robust, highly stretchable supramolecular polymer conductive hydrogel with self-healability and thermo-processability. Sci Rep. 2017;7(1):1-11. doi: 10.1002/anie.201804400
- Zhu S, Wang J, Yan H, et al. An injectable supramolecular self-healing bio-hydrogel with high stretchability, extensibility and ductility, and a high swelling ratio. J Mater Chem B. 2017;5(34):7021-7034. doi: 10.1039/C7TB01183K
- Lorson T, Jaksch S, Lübtow MM, et al. A thermogelling supramolecular hydrogel with sponge-like morphology as a cytocompatible bioink. Biomacromolecules. 2017;18(7): 2161-2171. doi: 10.1021/acs.biomac.7b00481
- Li L, Tian X, Yu X, Dong S. Effects of acute and chronic heavy metal (Cu, Cd, and Zn) exposure on sea cucumbers (Apostichopus japonicus). Biomed Res Int. 2016;2016:4532697. doi: 10.1155/2016/4532697
- Hu T, Cui X, Zhu M, et al. 3D-printable supramolecular hydrogels with shear-thinning property: fabricating strength tunable bioink via dual crosslinking. Bioact Mater. 2020;5(4):808-818. doi: 10.1016/j.bioactmat.2020.06.001
- Gao F, Xu Z, Liang Q, et al. Osteochondral regeneration with 3D‐printed biodegradable high‐strength supramolecular polymer reinforced‐gelatin hydrogel scaffolds. Adv Sci. 2019;6(15):1900867. doi: 10.1002/advs.201900867
- Godoy-Gallardo M, Merino-Gómez M, Mateos-Timoneda MA, Eckhard U, Gil FJ, Perez RA. Advanced binary guanosine and guanosine 5’-monophosphate cell-laden hydrogels for soft tissue reconstruction by 3D bioprinting. ACS Appl Mater Interfaces. 2023;15(25):29729-29742. doi: 10.1021/acsami.2c23277
- Yang J, Fatima K, Zhou X, He C. Meticulously engineered three-dimensional-printed scaffold with microarchitecture and controlled peptide release for enhanced bone regeneration. Biomater Trans. 2024;5(1):69. doi: 10.12336/biomatertransl.2024.01.007
- Li X, Jian H, Han Q, et al. Three-dimensional (3D) bioprinting of medium toughened dipeptide hydrogel scaffolds with Hofmeister effect. J Colloid Interface Sci. 2023;639:1-6. doi: 10.1016/j.jcis.2023.02.033
- Liu X, Song S, Chen Z, et al. Release of O-GlcNAc transferase inhibitor promotes neuronal differentiation of neural stem cells in 3D bioprinted supramolecular hydrogel scaffold for spinal cord injury repair. Acta Biomater. 2022;151:148-162. doi: 10.1016/j.actbio.2022.08.031
- Shim J-H, Jang K-M, Hahn SK, et al. Three-dimensional bioprinting of multilayered constructs containing human mesenchymal stromal cells for osteochondral tissue regeneration in the rabbit knee joint. Biofabrication. 2016;8(1):014102. doi: 10.1088/1758-5090/8/1/014102
- Chen H, Hou S, Ma H, Li X, Tan Y. Controlled gelation kinetics of cucurbit[7]uril-adamantane crosslinked supramolecular hydrogels with competing guest molecules. Sci Rep. 2016;6(1):20722. doi: 10.1038/srep20722
- Gao W, Chao H, Zheng Y-C, et al. Ionic carbazole-based water-soluble two-photon photoinitiator and the fabrication of biocompatible 3D hydrogel scaffold. ACS Appl Mater Interf. 2021;13(24):27796-27805. doi: 10.1021/acsami.1c02227
- Madl AC, Madl CM, Myung D. Injectable cucurbit [8] uril-based supramolecular gelatin hydrogels for cell encapsulation. ACS Macro Lett. 2020;9(4):619-626. doi: 10.1021/acsmacrolett.0c00184
- Zou H, Liu J, Li Y, Li X, Wang X. Cucurbit [8] uril‐based polymers and polymer materials. Small. 2018;14(46):1802234. doi: 10.1002/smll.201802234
- Liu YH, Zhang YM, Yu HJ, Liu Y. Cucurbituril‐based biomacromolecular assemblies. Angew Chem. 2021;133(8):3914-3924. doi: 10.1002/anie.202009797
- Wang Z, Shui M, Wyman IW, Zhang Q-W, Wang R. Cucurbit [8] uril-based supramolecular hydrogels for biomedical applications. RSC Med Chem. 2021;12(5):722-729. doi: 10.3390/molecules28083566
- Meng Z-J, Liu J, Yu Z, et al. Viscoelastic hydrogel microfibers exploiting cucurbit [8] uril host–guest chemistry and microfluidics. ACS Appl Mater Interfaces. 2020;12(15): 17929-17935. doi: 10.1021/acsami.9b21240
- Zou L, Braegelman AS, Webber MJ. Dynamic supramolecular hydrogels spanning an unprecedented range of host–guest affinity. ACS Appl Mater Interfaces. 2019;11(6):5695-5700. doi: 10.1021/acsami.8b22151
- Madl AC, Myung D. Supramolecular host–guest hydrogels for corneal regeneration. Gels. 2021;7(4):163. doi: 10.3390/gels7040163
- Wang Y, Zhang X, Wan K, Zhou N, Wei G, Su Z. Supramolecular peptide nano-assemblies for cancer diagnosis and therapy: from molecular design to material synthesis and function-specific applications. J Nanobiotechnol. 2021;19(1):1-31. doi: 10.1186/s12951-021-00999-x
- Wang H, Zhu H, Fu W, et al. A high strength self‐healable antibacterial and anti‐inflammatory supramolecular polymer hydrogel. Macromol Rapid Commun. 2017;38(9):1600695. doi: 10.1002/marc.201600695
- Park KM, Roh JH, Sung G, Murray J, Kim K. Self‐healable supramolecular hydrogel formed by nor‐seco‐cucurbit [10] uril as a supramolecular crosslinker. Chem Asian J. 2017;12(13):1461-1464. doi: 10.1002/asia.201700386
- Xiao T, Xu L, Zhou L, Sun X-Q, Lin C, Wang L. Dynamic hydrogels mediated by macrocyclic host–guest interactions. J Mater Chem B. 2019;7(10):1526-1540. doi: 10.1039/C8TB02339E
- Zhou Y, Zhang Y, Dai Z, Jiang F, Tian J, Zhang W. A super-stretchable, self-healing and injectable supramolecular hydrogel constructed by a host–guest crosslinker. Biomater Sci. 2020;8(12):3359-3369. doi: 10.1039/D0BM00290A
- Miller B, Hansrisuk A, Highley CB, Caliari SR. Guest–host supramolecular assembly of injectable hydrogel nanofibers for cell encapsulation. ACS Biomater Sci Eng. 2021;7(9): 4164-4174. doi: 10.1021/acsbiomaterials.1c00275
- Dai W, Zhang L, Yu Y, et al. 3D bioprinting of heterogeneous constructs providing tissue-specific microenvironment based on host–guest modulated dynamic hydrogel bioink for osteochondral regeneration. Adv Funct Mater. 2022;32(23):2200710. doi: 10.1002/adfm.202200710
- Wei W, Liu W, Kang H, et al. A one-stone-two-birds strategy for osteochondral regeneration based on a 3D printable biomimetic scaffold with kartogenin biochemical stimuli gradient. Adv Healthc Mater. 2023;12(15):2300108. doi: 10.1002/adhm.202300108
- Jain M, Nowak BP, Ravoo BJ. Supramolecular hydrogels based on cyclodextrins: progress and perspectives. Chem Nano Mat. 2022;8(5):e202200077. doi: 10.1002/cnma.202200077
- Rey-Rico A, Babicz H, Madry H, Concheiro A, Alvarez-Lorenzo C, Cucchiarini M. Supramolecular polypseudorotaxane gels for controlled delivery of rAAV vectors in human mesenchymal stem cells for regenerative medicine. Int J Pharm. 2017;531(2):492-503. doi: 10.1016/j.ijpharm.2017.05.050
- Alvarez-Lorenzo C, Garcia-Gonzalez CA, Concheiro A. Cyclodextrins as versatile building blocks for regenerative medicine. JCR. 2017;268:269-281. doi: 10.1016/j.jconrel.2017.10.038
- Xia D, Wang P, Ji X, Khashab NM, Sessler JL, Huang F. Functional supramolecular polymeric networks: the marriage of covalent polymers and macrocycle-based host– guest interactions. Chem Rev. 2020;120(13):6070-6123. doi: 10.1021/acs.chemrev.9b00839
- Harada A, Okada M, Li J, Kamachi M. Preparation and characterization of inclusion complexes of poly (propylene glycol) with cyclodextrins. Macromolecules. 1995;28(24):8406-8411. doi: 10.1021/ma00128a060
- Simões S, Veiga F, Torres-Labandeira J, et al. Syringeable pluronic–α-cyclodextrin supramolecular gels for sustained delivery of vancomycin. Eur J Pharm Biopharm. 2012;80(1):103-112. doi: 10.1016/j.ejpb.2011.09.017
- Li J, Li X, Ni X, Wang X, Li H, Leong KW. Self-assembled supramolecular hydrogels formed by biodegradable PEO–PHB–PEO triblock copolymers and α-cyclodextrin for controlled drug delivery. Biomaterials. 2006;27(22): 4132-4140. doi: 10.1016/j.biomaterials.2006.03.025
- Khodaverdi E, Heidari Z, Tabassi SAS, et al. Injectable supramolecular hydrogel from insulin-loaded triblock PCL-PEG-PCL copolymer and γ-cyclodextrin with sustained-release property. AAPS Pharm Sci Tech. 2015;16:140-149. doi: 10.1208/s12249-014-0198-4
- Rey-Rico A, Cucchiarini M. Supramolecular cyclodextrin-based hydrogels for controlled gene delivery. Polymers. 2019;11(3):514. doi: 10.3390/polym11030514
- Kauscher U, Stuart MCA, Drücker P, Galla H-J, Ravoo BJ.Incorporation of amphiphilic cyclodextrins into liposomes as artificial receptor units. Langmuir. 2013;29(24): 7377-7383. doi: 10.1021/la3045434
- Redondo-Gómez C, Abdouni Y, Becer CR, Mata A. Self-assembling hydrogels based on a complementary host–guest peptide amphiphile pair. Biomacromolecules. 2019;20(6):2276-2285. doi: 10.1021/acsbiomaterials.0c00549
- Redondo-Gómez C, Padilla-Lopategui S, Azevedo HS, Mata A. Host–guest-mediated epitope presentation on self-assembled peptide amphiphile hydrogels. ACS Biomater Sci Eng. 2020;6(9):4870-4880. doi: 10.1021/acsbiomaterials.0c00549
- Nowak BP, Ravoo BJ. Magneto-and photo-responsive hydrogels from the co-assembly of peptides, cyclodextrins, and superparamagnetic nanoparticles. Farad Disc. 2019;219:220-228. doi: 10.1039/C9FD00012G
- Wang J, Williamson GS, Yang H. Branched polyrotaxane hydrogels consisting of alpha-cyclodextrin and low-molecular-weight four-arm polyethylene glycol and the utility of their thixotropic property for controlled drug release. Colloids Surf B Biointerfaces. 2018;165:144-149. doi: 10.1016/j.colsurfb.2018.02.032
- Dai L, Liu K, Wang L, et al. Injectable and thermosensitive supramolecular hydrogels by inclusion complexation between binary-drug loaded micelles and α-cyclodextrin. Mater Sci Eng C. 2017;76:966-974. doi: 10.1002/adfm.202200710
- Zohreband Z, Adeli M, Zebardasti A. Self-healable and flexible supramolecular gelatin/MoS2 hydrogels with molecular recognition properties. Int J Biol Macromol. 2021;182:2048-2055. doi: 10.1016/j.ijbiomac.2021.05.106
- Singh A, Zhan J, Ye Z, Elisseeff JH. Modular multifunctional poly (ethylene glycol) hydrogels for stem cell differentiation. Adv Funct Mater. 2013;23(5):575-582. doi: 10.1002/adfm.201201902
- Aramoto H, Osaki M, Konishi S, et al. Redox-responsive supramolecular polymeric networks having double-threaded inclusion complexes. Chem Sci. 2020;11(17): 4322-4331. doi: 10.1039/C9SC05589D
- Arisaka Y, Tonegawa A, Tamura A, Yui N. Terminally cross‐linking polyrotaxane hydrogels applicable for cellular microenvironments. J Appl Polym Sci. 2021;138(3):49706. doi: 10.1002/app.49706
- Arisaka Y, Yui N. Polyrotaxane-based biointerfaces with dynamic biomaterial functions. J Mater Chem B. 2019;7(13):2123-2129. doi: 10.1039/C9TB00256A
- Cho IS, Ooya T. Cell‐encapsulating hydrogel puzzle: polyrotaxane‐based self‐healing hydrogels. Chem A Eur J. 2020;26(4):913-920. doi: 10.1002/chem.201904446
- Uekama K, Hirayama F, Irie T. Cyclodextrin drug carrier systems. Chem Rev. 1998;98(5):2045-2076. doi: 10.1021/cr970025p
- Li Z, Yin H, Zhang Z, Liu KL, Li J. Supramolecular anchoring of DNA polyplexes in cyclodextrin-based polypseudorotaxane hydrogels for sustained gene delivery. Biomacromolecules. 2012;13(10):3162-3172. doi: 10.1021/bm300936x
- Segredo-Morales E, Martin-Pastor M, Salas A, et al. Mobility of water and polymer species and rheological properties of supramolecular polypseudorotaxane gels suitable for bone regeneration. Bioconjug Chem. 2018;29(2):503-516. doi: 10.1021/acs.bioconjchem.7b00823
- Ohshita N, Motoyama K, Iohara D, et al. Polypseudorotaxane-based supramolecular hydrogels consisting of cyclodextrins and Pluronics as stabilizing agents for antibody drugs. Carbohydr Polym. 2021;256:117419. doi: 10.1016/j.carbpol.2020.117419
- Jian H, Wang M, Dong Q, et al. Dipeptide self-assembled hydrogels with tunable mechanical properties and degradability for 3D bioprinting. ACS Appl Materi Inter. 2019;11(50):46419-46426. doi: 10.1021/acsami.9b13905
- Farsheed AC, Thomas AJ, Pogostin BH, Hartgerink JD. 3D printing of self‐assembling nanofibrous multidomain peptide hydrogels. Adv Materi. 2023;35(11):2210378. doi: 10.1002/adma.202210378
- Chu B, He J-m, Wang Z, et al. Proangiogenic peptide nanofiber hydrogel/3D printed scaffold for dermal regeneration. Chem Eng J. 2021;424:128146. doi: 10.1016/j.cej.2020.128146
- Li Y, Wang F, Cui H. Peptide‐based supramolecular hydrogels for delivery of biologics. Bioeng Transl Med. 2016;1(3): 306-322. doi: 10.1002/btm2.10041
- Jagrosse ML, Agredo P, Abraham BL, Toriki ES, Nilsson BL. Supramolecular phenylalanine-derived hydrogels for the sustained release of functional proteins. ACS Biomater Sci Eng. 2023;9(2):784-796. doi: 10.1021/acsbiomaterials.2c01299
- Rajbhandary A, Raymond DM, Nilsson BL. Self-assembly, hydrogelation, and nanotube formation by cation-modified phenylalanine derivatives. Langmuir. 2017;33(23): 5803-5813. doi: 10.1021/acs.langmuir.7b00686
- Misra R, Tang Y, Chen Y, et al. Exploiting minimalistic backbone engineered γ‐phenylalanine for the formation of supramolecular co‐polymer. Macromol Rapid Commun. 2022;43(19):2200223. doi: 10.1002/marc.202200223
- Li W, Hu X, Chen J, Wei Z, Song C, Huang R. N-(9- Fluorenylmethoxycarbonyl)-L-phenylalanine/nano-hydroxyapatite hybrid supramolecular hydrogels as drug delivery vehicles with antibacterial property and cytocompatibility. J Mater Sci: Mater Med. 2020;31:1-9. doi: 10.1007/s10856-020-06410-9
- Dang-i AY, Kousar A, Liu J, et al. Mechanically stable C2-phenylalanine hybrid hydrogels for manipulating cell adhesion. ACS Appl Mater Interfaces. 2019;11(32): 28657-28664. doi: 10.1021/acsami.9b08655
- Chakraborty P, Ghosh M, Schnaider L, et al. Composite of peptide‐supramolecular polymer and covalent polymer comprises a new multifunctional, bio‐inspired soft material. Macromol Rapid Commun. 2019;40(18):1900175. doi: 10.1002/marc.201900175
- Misra R, Sharma A, Shiras A, Gopi HN. Backbone engineered γ-peptide amphitropic gels for immobilization of semiconductor quantum dots and 2D cell culture. Langmuir. 2017;33(31):7762-7768. doi: 10.1021/acs.langmuir.7b01283
- Wu C, Li R, Yin Y, Wang J, Zhang L, Zhong W. Redox-responsive supramolecular hydrogel based on 10-hydroxy camptothecin-peptide covalent conjugates with high loading capacity for drug delivery. Mater Sci Eng C. 2017;76: 196-202. doi: 10.1016/j.msec.2017.03.103
- Ren C, Gao Y, Liu J, et al. Anticancer supramolecular hydrogel of D/L-peptide with enhanced stability and bioactivity. J Biomed Nanotechnol. 2018;14(6):1125-1134. doi: 10.1166/jbn.2018.2564
- Wei K, Chen X, Zhao P, et al. Stretchable and bioadhesive supramolecular hydrogels activated by a one-stone–two-bird postgelation functionalization method. ACS Appl Mater Interfaces. 2019;11(18):16328-16335. doi: 10.1021/acsami.9b03029
- Zhang Y, Zhang H, Zou Q, Xing R, Jiao T, Yan X. An injectable dipeptide–fullerene supramolecular hydrogel for photodynamic antibacterial therapy. J Mater Chem B. 2018;6(44):7335-7342. doi: 10.1039/C8TB01487F
- Clarke DE, Parmenter CD, Scherman OA. Tunable pentapeptide self‐assembled β‐sheet hydrogels. Angew Chem Int Ed. 2018;57(26):7709-7713. doi: 10.1002/anie.201801001
- Diaferia C, Netti F, Ghosh M, et al. Bi-functional peptide-based 3D hydrogel-scaffolds. Soft Matter. 2020;16(30): 7006-7017. doi: 10.1039/D0SM00825G
- Dorishetty P, Dutta NK, Choudhury NR. Bioprintable tough hydrogels for tissue engineering applications. Adv Colloid Interface Sci. 2020;281:102163. doi: 10.1016/j.cis.2020.102163
- Chakraborty P, Oved H, Bychenko D, et al. Nanoengineered peptide‐based antimicrobial conductive supramolecular biomaterial for cardiac tissue engineering. Adv Mater. 2021;33(26):2008715. doi: 10.1002/adma.202008715
- Falcone N, Shao T, Andoy NMO, et al. Multi-component peptide hydrogels–a systematic study incorporating biomolecules for the exploration of diverse, tuneable biomaterials. Biomater Sci. 2020;8(20):5601-5614. doi: 10.1039/D0BM01104E
- Fichman G, Schneider JP. Utilizing Frémy’s salt to increase the mechanical rigidity of supramolecular peptide-based gel networks. Fbioe. 2021;8:594258. doi: 10.3389/fbioe.2020.594258
- Chowdhuri S, Saha A, Pramanik B, et al. Smart thixotropic hydrogels by disulfide-linked short peptides for effective three-dimensional cell proliferation. Langmuir. 2020;36(50):15450-15462. doi: 10.1021/acs.langmuir.0c03324
- Mañas-Torres MC, Gila-Vilchez C, Vazquez-Perez FJ, et al. Injectable magnetic-responsive short-peptide supramolecular hydrogels: ex vivo and in vivo evaluation. ACS Appl Mater Interfaces. 2021;13(42):49692-49704. doi: 10.1021/acsami.1c13972
- Vrehen AF, Rutten MGTA, Dankers PYW. Development of a fully synthetic corneal stromal construct via supramolecular hydrogel engineering. Adv Healthc Mater. 2023;12(32):2301392. doi: 10.1002/adhm.202301392
- Zhou K, Ding R, Tao X, et al. Peptide-dendrimer-reinforced bioinks for 3D bioprinting of heterogeneous and biomimetic in vitro models. Acta Biomater. 2023;169:243-255. doi: 10.1016/j.actbio.2023.08.008
- Chiesa I, Ligorio C, Bonatti AF, et al. Modeling the three-dimensional bioprinting process of β-sheet self-assembling peptide hydrogel scaffolds. Fmedt. 2020;2:571626. doi: 10.3389/fmedt.2020.571626
- Aronsson C, Jury M, Naeimipour S, et al. Dynamic peptide-folding mediated biofunctionalization and modulation of hydrogels for 4D bioprinting. Biofabrication. 2020;12(3):035031. doi: 10.1088/1758-5090/ab9490
- Zhao Y, Xing Y, Wang M, et al. Supramolecular hydrogel based on an osteogenic growth peptide promotes bone defect repair. ACS Omega. 2022;7(13):11395-11404. doi: 10.1021/acsomega.2c00501
- Yan M, Lewis P, Shah R. Tailoring nanostructure and bioactivity of 3D-printable hydrogels with self-assemble peptides amphiphile (PA) for promoting bile duct formation. Biofabrication. 2018;10(3):035010. doi: 10.1088/1758-5090/aac902
- Sather NA, Sai H, Sasselli IR, et al. 3D printing of supramolecular polymer hydrogels with hierarchical structure. Small. 2021;17(5):2005743. doi: 10.1002/smll.202005743
- Das AK, Gavel PK. Low molecular weight self-assembling peptide-based materials for cell culture, antimicrobial, anti-inflammatory, wound healing, anticancer, drug delivery, bioimaging and 3D bioprinting applications. Soft Matter. 2020;16(44):10065-10095. doi: 10.1039/D0SM01136C
- Cai L, Liu S, Guo J, Jia Y-G. Polypeptide-based self-healing hydrogels: design and biomedical applications. Acta Biomater. 2020;113:84-100. doi: 10.1016/j.actbio.2020.07.001
- Li C, Faulkner‐Jones A, Dun AR, et al. Rapid formation of a supramolecular polypeptide–DNA hydrogel for in situ three‐dimensional multilayer bioprinting. Angew Chem Int Ed. 2015;54(13):3957-3961. doi: 10.1002/anie.201411383
- Zhang J, Lu N, Peng H, et al. Multi-triggered and enzyme-mimicking graphene oxide/polyvinyl alcohol/G-quartet supramolecular hydrogels. Nanoscale. 2020;12(8): 5186-5195. doi: 10.1039/C9NR10779G
- Yang B, Zhao Z, Pan Y, et al. Shear-thinning and designable responsive supramolecular DNA hydrogels based on chemically branched DNA. ACS Appl Mater Interfaces. 2021;13(41):48414-48422. doi: 10.1021/acsami.1c15494
- Wu J, Liyarita BR, Zhu H, Liu M, Hu X, Shao F. Self-assembly of dendritic DNA into a hydrogel: application in three-dimensional cell culture. ACS Appl Mater Interfaces. 2021;13(42):49705-49712. doi: 10.1021/acsami.1c14445
- Sousa V, Amaral AJ, Castanheira EJ, et al. Self-supporting hyaluronic acid-functionalized G-quadruplex-based perfusable multicomponent hydrogels embedded in photo-crosslinkable matrices for bioapplications. Biomacromolecules. 2023;24(7):3380-3396. doi: 10.1021/acs.biomac.3c00433
- Tang Q, Plank TN, Zhu T, et al. Self-assembly of metallo-nucleoside hydrogels for injectable materials that promote wound closure. ACS Appl Mater Interfaces. 2019;11(22):19743-19750. doi: 10.1021/acsami.9b02265
- Shen CY, Wang J, Li GF, et al. Boosting cartilage repair with silk fibroin-DNA hydrogel-based cartilage organoid precursor. Bioact Mater. May 2024;35:429-444. doi: 10.1016/j.bioactmat.2024.02.016
- Shi J, Shi Z, Dong Y, Wu F, Liu D. Responsive DNA-based supramolecular hydrogels. ACS Appl Bio Mater. 2020;3(5):2827-2837. doi: 10.1021/acsabm.0c00081
- Shao Y, Jia H, Cao T, Liu D. Supramolecular hydrogels based on DNA self-assembly. Acc Chem Res. 2017;50(4):659-668. doi: 10.1021/acs.accounts.6b00524
- Wei Y, Wang K, Luo S, et al. Programmable DNA hydrogels as artificial extracellular matrix. Small. 2022;18(36):2107640. doi: 10.1002/smll.202107640
- Budharaju H, Zennifer A, Sethuraman S, Paul A, Sundaramurthi D. Designer DNA biomolecules as a defined biomaterial for 3D bioprinting applications. Mater Horiz. 2022;9(4):1141-1166. doi: 10.1039/D1MH01632F
- Wang D, Duan J, Liu J, et al. Stimuli-responsive self-degradable DNA hydrogels: design, synthesis, and applications. Adv Healthc Mater. 2023;12(16):2203031. doi: 10.1002/adhm.202203031
- Tang M, Zhong Z, Ke C. Advanced supramolecular design for direct ink writing of soft materials. Chem Soc Rev. 2023;52(5):1614-1649. doi: 10.1039/D2CS01011A
- Miao Y, Chen Y, Luo J, et al. Black phosphorus nanosheets-enabled DNA hydrogel integrating 3D-printed scaffold for promoting vascularized bone regeneration. Bioact Mater. 2023;21:97-109. doi: 10.1016/j.bioactmat.2022.08.005
- Li J, Lai Y, Li M, et al. Repair of infected bone defect with clindamycin-tetrahedral DNA nanostructure complex-loaded 3D bioprinted hybrid scaffold. Chem Eng J. 2022;435:134855. doi: 10.1016/j.cej.2022.134855
- Wu W, Zhang Z, Xiong T, et al. Calcium ion coordinated dexamethasone supramolecular hydrogel as therapeutic alternative for control of non-infectious uveitis. Acta Biomater. 2017;61:157-168. doi: 10.1016/j.actbio.2017.05.024
- Chen Z, Xing L, Fan Q, et al. Drug-bearing supramolecular filament hydrogels as anti-inflammatory agents. Theranostics. 2017;7(7):2003. doi: 10.7150/thno.19404
- Liu X, Chen X, Chua MX, Li Z, Loh XJ, Wu YL. Injectable supramolecular hydrogels as delivery agents of Bcl‐2 conversion gene for the effective shrinkage of therapeutic resistance tumors. Adv Healthc Mater. 2017;6(11):1700159. doi: 10.1002/adhm.201700159
- Liu Z, Xu G, Wang C, Li C, Yao P. Shear-responsive injectable supramolecular hydrogel releasing doxorubicin loaded micelles with pH-sensitivity for local tumor chemotherapy. Int J Pharm. 2017;530(1-2):53-62. doi: 10.1016/j.ijpharm.2017.07.063
- Bakker MH, van Rooij E, Dankers PY. Controlled release of RNAi molecules by tunable supramolecular hydrogel carriers. Chem Asian J. 2018;13(22):3501-3508. doi: 10.1002/asia.201800582
- Liu X, Chen X, Chua MX, Li Z, Loh XJ, Wu Y-L. Injectable supramolecular hydrogels as delivery agents of Bcl-2 conversion gene for the effective shrinkage of therapeutic resistance tumors. Adv Healthc Mater. 2017;6(11):1700159. doi: 10.1002/adhm.201700159
- d’Aquino AI, Maikawa CL, Nguyen LT, et al. Use of a biomimetic hydrogel depot technology for sustained delivery of GLP-1 receptor agonists reduces burden of diabetes management. Cell Rep Med. 2023;4(11): 101292. doi: 10.1016/j.xcrm.2023.101292
- Davis ME, Brewster ME. Cyclodextrin-based pharmaceutics: past, present and future. Nat Rev Drug Discov. 2004;3(12):1023-1035. doi: 10.1038/nrd1576
- Ma D, Zhang L-M. Novel biosensing platform based on self-assembled supramolecular hydrogel. Mater Sci Eng C. 2013;33(5):2632-2638. doi: 10.1016/j.msec.2013.02.023
- Li J, Kuang Y, Gao Y, Du X, Shi J, Xu B. D-amino acids boost the selectivity and confer supramolecular hydrogels of a nonsteroidal anti-inflammatory drug (NSAID). J Am Chem Soc. 2013;135(2):542-545. doi: 10.1021/ja310019x
- Li X, Li J, Gao Y, Kuang Y, Shi J, Xu B. Molecular nanofibers of olsalazine form supramolecular hydrogels for reductive release of an anti-inflammatory agent. J Am Chem Soc. 2010;132(50):17707-17709. doi: 10.1021/ja109269v
- Xuan X, Zhou Y, Chen A, et al. Silver crosslinked injectable bFGF-eluting supramolecular hydrogels speed up infected wound healing. J Mater Chem B. 2020;8(7): 1359-1370. doi: 10.1039/C9TB02331C
- Zhao W, Li Y, Zhang X, et al. Photo-responsive supramolecular hyaluronic acid hydrogels for accelerated wound healing. JCR. 2020;323:24-35. doi: 10.1016/j.jconrel.2020.04.014
- Niu Y, Guo T, Yuan X, Zhao Y, Ren L. An injectable supramolecular hydrogel hybridized with silver nanoparticles for antibacterial application. Soft Matter. 2018;14(7):1227-1234. doi: 10.1039/C7SM02251D
- Zheng Y, Yuan W, Liu H, Huang S, Bian L, Guo R. Injectable supramolecular gelatin hydrogel loading of resveratrol and histatin-1 for burn wound therapy. Biomater Sci. 2020;8(17):4810-4820. doi: 10.1039/D0BM00391C
- Zhai Z, Xu K, Mei L, et al. Co-assembled supramolecular hydrogels of cell adhesive peptide and alginate for rapid hemostasis and efficacious wound healing. Soft Matter. 2019;15(42):8603-8610. doi: 10.1039/C9SM01296F
- Preman NK, ES SP, Prabhu A, et al. Bioresponsive supramolecular hydrogels for hemostasis, infection control and accelerated dermal wound healing. J Mater Chem B. 2020;8(37):8585-8598. doi: 10.1039/D0TB01468K
- Wang W, Zeng Z, Xiang L, et al. Injectable self-healing hydrogel via biological environment-adaptive supramolecular assembly for gastric perforation healing. ACS Nano. 2021;15(6):9913-9923. doi: 10.1021/acsnano.1c01199
- Li Y, Zhu C, Dong Y, Liu D. Supramolecular hydrogels: mechanical strengthening with dynamics. Polymer. 2020;210:122993. doi: 10.1016/j.polymer.2020.122993
- Kim J-H, Park K, Nam HY, Lee S, Kim K, Kwon IC. Polymers for bioimaging. Prog Polym Sci. 2007;32(8-9): 1031-1053. doi: 10.1016/j.progpolymsci.2007.05.016
- Zhu Q, Qiu F, Zhu B, Zhu X. Hyperbranched polymers for bioimaging. Rsc Adv. 2013;3(7):2071-2083. doi: 10.1039/C2RA22210H
- Mehwish N, Dou X, Zhao Y, Feng C-L. Supramolecular fluorescent hydrogelators as bio-imaging probes. Mater Horiz. 2019;6(1):14-44. doi: 10.1039/C8MH01130C
- Gao F, Yang X, Song W. Bioinspired supramolecular hydrogel from design to applications. Small Methods. 2023:2300753. doi: 10.1002/smtd.202300753
- Völlmecke K, Afroz R, Bierbach S, et al. Hydrogel-based biosensors. Gels. 2022;8(12):768. doi: 10.3390/gels8120768
- Li F, Lyu D, Liu S, Guo W. DNA hydrogels and microgels for biosensing and biomedical applications. Adv Mater. 2020;32(3):1806538. doi: 10.1002/adma.201806538
- Lin X, Zhao X, Xu C, Wang L, Xia Y. Progress in the mechanical enhancement of hydrogels: fabrication strategies and underlying mechanisms. J Polym Sci. 2022;60(17): 2525-2542. doi: 10.1002/pol.20220154
- Omar J, Ponsford D, Dreiss CA, Lee TC, Loh XJ. Supramolecular hydrogels: design strategies and contemporary biomedical applications. Chem Asian J. 2022;17(9):e202200081. doi: 10.1002/asia.202200081
- Zou L, Su B, Addonizio CJ, Pramudya I, Webber MJ. Temperature-responsive supramolecular hydrogels by ternary complex formation with subsequent photo-crosslinking to alter network dynamics. Biomacromolecules. 2019;20(12):4512-4521.doi: 10.1021/acs.biomac.9b01267
- Yang B, Liang C, Chen D, et al. A conductive supramolecular hydrogel creates ideal endogenous niches to promote spinal cord injury repair. Bioact Mater. 2022;15:103-119. doi: 10.1016/j.bioactmat.2021.11.032
- Zhai X, Ma Y, Hou C, et al. 3D-printed high strength bioactive supramolecular polymer/clay nanocomposite hydrogel scaffold for bone regeneration. ACS Biomater Sci Eng. 2017;3(6):1109-1118. doi: 10.1021/acsbiomaterials.7b00224
- Jeong SH, Kim M, Kim TY, Kim H, Ju JH, Hahn SK. Supramolecular injectable hyaluronate hydrogels for cartilage tissue regeneration. ACS Appl Bio Mater. 2020;3(8):5040-5047. doi: 10.1021/acsabm.0c00537
- Andriamiseza F, Peters S, Roux C, Dietrich N, Coudret C, Fitremann J. Wet spinning and 3D printing of supramolecular hydrogels in acid-base and dynamic conditions. Colloids Surf A Physicochem Eng Asp. 2023;673:131765. doi: 10.1016/j.colsurfa.2023.131765
- Zhang X, Fan J, Lee C-S, Kim S, Chen C, Lee M. Supramolecular hydrogels based on nanoclay and guanidine-rich chitosan: injectable and moldable osteoinductive carriers. ACS Appl Bio Mater Interf. 2020;12(14):16088-16096. doi: 10.1021/acsami.0c01241
- Qin Z, Yu X, Wu H, Li J, Lv H, Yang X. Nonswellable and tough supramolecular hydrogel based on strong micelle crosslinkings. Biomacromolecules. 2019;20(9): 3399-3407. doi: 10.1021/acs.biomac.9b00666
- Li N, Liu C, Chen W. Facile access to guar gum based supramolecular hydrogels with rapid self-healing ability and multistimuli responsive gel–sol transitions. J Agric Food Chem. 2018;67(2):746-752. doi: 10.1021/acs.jafc.8b05130