The importance of phylogenetic studies in the evolution of beta-lactamases: Tracing antimicrobial resistance in priority pathogens
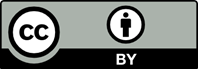
Beta-lactams have long been the standard therapeutic strategy for combating bacterial infections. However, the increasing resistance to these antibiotics has become a growing concern. One of the primary contributors to bacterial resistance is the production of beta-lactamases (BLs), enzymes capable of hydrolyzing the beta-lactam ring of these antibiotics, rendering them ineffective. In response, several alternative therapeutic strategies have been tested, but their success rate has been limited, highlighting the need for further research in this domain. Thus, it is crucial to study the evolution of BLs and their variants, as well as their transmission dynamics. In addition, cryptic genetic variations (CGVs), which act as hidden reservoirs of potential future phenotypes of BLs, have received insufficient attention. Identifying CGVs could help pinpoint selective pressures to avoid to prevent the evolution of BLs. Unlike conventional reviews that focus on classes of BLs or their resistance mechanisms, this article emphasizes the evolutionary perspectives of BLs. We explore the role of phylogenetic analysis in understanding the rise of different BL variants and their evolution in response to different selective pressures and gene transfer events. Most importantly, this review underscores the evolution of BLs, particularly in the context of the priority pathogens listed by the World Health Organization.

- Hall BG, Barlow M. Evolution of the serine beta-lactamases: Past, present and future. Drug Resist Updat. 2004;7(2):111-123. doi: 10.1016/j.drup.2004.02.003
- Carcione D, Siracusa C, Sulejmani A, Leoni V, Intra J. Old and new beta-lactamase inhibitors: Molecular structure, mechanism of action, and clinical use. Antibiotics (Basel). 2021;10(8):995. doi: 10.3390/antibiotics10080995
- Arer V, Anurag Anand A, Samanta SK, Kar D. Deeper insights into the action of class C β-lactamases against cephalosporins through molecular docking and MD simulation studies. Biologia (Bratisl). 2024;79(12):3695-3709. doi: 10.1007/s11756-024-01802-6
- Mondal RK, Sen D, Arya A, Samanta SK. Developing anti-microbial peptide database version 1 to provide comprehensive and exhaustive resource of manually curated AMPs. Sci Rep. 2023;13(1):17843. doi: 10.1038/s41598-023-45016-3
- Naghavi M, Vollset SE, Ikuta KS, et al. Global burden of bacterial antimicrobial resistance 1990-2021: A systematic analysis with forecasts to 2050. Lancet. 2024;404(10459):1199-1226. doi: 10.1016/s0140-6736(24)01867-1
- Bush K, Bradford PA. Β-lactams and β-lactamase inhibitors: An overview. Cold Spring Harb Perspect Med. 2016;6(8):a025247. doi: 10.1101/cshperspect.a025247
- Perez F, Bonomo RA. Can we really use ß-lactam/ß-lactam inhibitor combinations for the treatment of infections caused by extended-spectrum ß-lactamase-producing bacteria? Clin Infect Dis. 2012;54(2):175-177. doi: 10.1093/cid/cir793
- Qin XL, Chen Y, Wu XZ, et al. Emerging epidemic of the Africa-type plasmid in penicillinase-producing Neisseria gonorrhoeae in Guangdong, China, 2013-2022. Emerg Microbes Infect. 2025;14(1):2440489. doi: 10.1080/22221751.2024.2440489
- Vázquez-Ucha JC, Arca-Suárez J, Bou G, Beceiro A. New carbapenemase inhibitors: Clearing the way for the β-lactams. Int J Mol Sci. 2020;21(23):9308. doi: 10.3390/ijms21239308
- Meletis G. Carbapenem resistance: Overview of the problem and future perspectives. Ther Adv Infect Dis. 2016;3(1):15-21. doi: 10.1177/2049936115621709
- Mojica MF, Rossi MA, Vila AJ, Bonomo RA. The urgent need for metallo-β-lactamase inhibitors: An unattended global threat. Lancet Infect Dis. 2022;22(1):e28-e34. doi: 10.1016/s1473-3099(20)30868-9
- Papp-Wallace KM, Endimiani A, Taracila MA, Bonomo RA. Carbapenems: Past, present, and future. Antimicrob Agents Chemother. 2011;55(11):4943-4960. doi: 10.1128/aac.00296-11
- Dadgostar P. Antimicrobial resistance: Implications and costs. Infect Drug Resist. 2019;12:3903-3910. doi: 10.2147/idr.s234610
- World Health Organization. WHO Publishes List of Bacteria for which New Antibiotics Are Urgently Needed. Available from: https://www.who.int/news/item/27-02-2017-who-publishes-list-of-bacteria-for-which-new-antibiotics-are-urgently-needed [Last accessed on 2025 Mar 03].
- World Health Organization. WHO Bacterial Priority Pathogens List, 2024: Bacterial Pathogens of Public Health Importance to Guide Research, Development and Strategies to Prevent and Control Antimicrobial Resistance. World Health Organization; 2024. Available from: https://www. who.int/publications/i/item/9789240093461 [Last accessed on 2025 Mar 03].
- Arer V, Kar D. Biochemical exploration of β-lactamase inhibitors. Front Genet. 2023;13:1060736. doi: 10.3389/fgene.2022.1060736
- Bush K, Jacoby GA. Updated functional classification of β-lactamases. Antimicrob Agents Chemother. 2010; 54(3):969-976. doi: 10.1128/aac.01009-09
- Tooke CL, Hinchliffe P, Bragginton EC, et al. Β-lactamases and β-lactamase inhibitors in the 21st century. J Mol Biol. 2019;431(18):3472-3500. doi: 10.1016/j.jmb.2019.04.002
- Heil EL, McCreary EK. REVISITing treatment of metallo- β-lactamases. Lancet Infect Dis. 2025;25(2):144-146. doi: 10.1016/s1473-3099(24)00561-9
- Anurag Anand A, Sahoo AK, Samanta SK. Exploring the potential of designed peptides containing lysine and arginine repeats against VIM-2 metallo-beta-lactamases. Int J Pept Res Ther. 2024;30:41. doi: 10.1007/s10989-024-10619-5
- Oelschlaeger P. Β-lactamases: Sequence, structure, function, and inhibition. Biomolecules. 2021;11(7):986. doi: 10.3390/biom11070986
- Lu WJ, Hsu PH, Lin HTV. A novel cooperative metallo- β-lactamase fold metallohydrolase from pathogen Vibrio vulnificus exhibits β-lactam antibiotic-degrading activities. Antimicrob Agents Chemother. 2021;65(9):e0032621. doi: 10.1128/aac.00326-21
- Palzkill T. Metallo‐β‐lactamase structure and function. Ann N Y Acad Sci. 2013;1277(1):91-104. doi: 10.1111/j.1749-6632.2012.06796.x
- Feng S, Wu Z, Liang W, et al. Prediction of antibiotic resistance evolution by growth measurement of all proximal mutants of beta-lactamase. Mol Biol Evol. 2022;39(5):msac086. doi: 10.1093/molbev/msac086
- Stiffler MA, Hekstra DR, Ranganathan R. Evolvability as a function of purifying selection in TEM-1 β-lactamase. Cell. 2015;160(5):882-892. doi: 10.1016/j.cell.2015.01.035
- Schenk MF, Szendro IG, Salverda MLM, Krug J, de Visser JAGM. Patterns of epistasis between beneficial mutations in an antibiotic resistance gene. Mol Biol Evol. 2013;30(8):1779-1787. doi: 10.1093/molbev/mst096
- Das SG, Direito SOL, Waclaw B, Allen RJ, Krug J. Predictable properties of fitness landscapes induced by adaptational tradeoffs. Elife. 2020;9:e55155. doi: 10.7554/elife.55155
- Meini MR, Tomatis PE, Weinreich DM, Vila AJ. Quantitative description of a protein fitness landscape based on molecular features. Mol Biol Evol. 2015;32(7):1774-1787. doi: 10.1093/molbev/msv059
- Baier F, Hong N, Yang G, et al. Cryptic genetic variation shapes the adaptive evolutionary potential of enzymes. Elife. 2019;8:e40789. doi: 10.7554/elife.40789
- Rancati G, Moffat J, Typas A, Pavelka N. Emerging and evolving concepts in gene essentiality. Nat Rev Genet. 2018;19(1):34-49. doi: 10.1038/nrg.2017.74
- Fröhlich C, Gama JA, Harms K, et al. Cryptic β-lactamase evolution is driven by low β-lactam concentrations. mSphere. 2021;6(2):e00108-21. doi: 10.1128/msphere.00108-21
- Spratt BG, Maiden MCJ. Bacterial population genetics, evolution and epidemiology. Philos Trans R Soc Lond B Biol Sci. 1999;354(1384):701-710. doi: 10.1098/rstb.1999.0423
- San Millan A, Escudero JA, Gifford DR, Mazel D, MacLean RC. Multicopy plasmids potentiate the evolution of antibiotic resistance in bacteria. Nat Ecol Evol. 2016;1(1):10. doi: 10.1038/s41559-016-0010
- Gniadkowski M. Evolution of extended-spectrum β-lactamases by mutation. Clin Microbiol Infect. 2008;14:11-32. doi: 10.1111/j.1469-0691.2007.01854.x
- Stoesser N, Sheppard AE, Peirano G, et al. Genomic epidemiology of global Klebsiella pneumoniae carbapenemase (KPC)-producing Escherichia coli. Sci Rep. 2017;7(1):5917. doi: 10.1038/s41598-017-06256-2
- Bush K, Bradford PA. Epidemiology of β-lactamase-producing pathogens. Clin Microbiol Rev. 2020;33(2):e00047-19. doi: 10.1128/cmr.00047-19
- Liu G, Thomsen LE, Olsen JE. Antimicrobial-induced horizontal transfer of antimicrobial resistance genes in bacteria: A mini-review. J Antimicrob Chemother. 2022;77(3):556-567. doi: 10.1093/jac/dkab450
- Boyd SE, Livermore DM, Hooper DC, Hope WW. Metallo- β-lactamases: Structure, function, epidemiology, treatment options, and the development pipeline. Antimicrob Agents Chemother. 2020;64(10):e00397-20. doi: 10.1128/aac.00397-20
- Sonkar V, Venu V, Nishil B, Thatikonda S. Review on antibiotic pollution dynamics: Insights to occurrence, environmental behaviour, ecotoxicity, and management strategies. Environ Sci Pollut Res Int. 2024;31(39):51164- 51196. doi: 10.1007/s11356-024-34567-1
- Bengtsson-Palme J, Kristiansson E, Larsson DGJ. Environmental factors influencing the development and spread of antibiotic resistance. FEMS Microbiol Rev. 2018;42(1):fux053. doi: 10.1093/femsre/fux053
- Barreto Miranda I, Ignatius R, Pfüller R, et al. High carriage rate of ESBL-producing Enterobacteriaceae at presentation and follow-up among travellers with gastrointestinal complaints returning from India and Southeast Asia. J Travel Med. 2016;23(2):tav024. doi: 10.1093/jtm/tav024
- European Centre for Disease Prevention and Control (ECDC). Risk Assessment on the Spread of Carbapenemase- Producing Enterobacteriaceae (CPE). Luxembourg: Publications Office of the European Union; 2011.
- Livermore DM, Canton R, Gniadkowski M, et al. CTX-M: Changing the face of ESBLs in Europe. J Antimicrob Chemother. 2006;59(2):165-174. doi: 10.1093/jac/dkl483
- Arnold RS, Thom KA, Sharma S, Phillips M, Kristie Johnson J, Morgan DJ. Emergence of Klebsiella pneumoniae carbapenemase-producing bacteria. South Med J. 2011;104(1):40-45. doi: 10.1097/smj.0b013e3181fd7d5a
- Müller C, Reuter S, Wille J, et al. A global view on carbapenem-resistant Acinetobacter baumannii. mBio. 2023;14(6):e0226023. doi: 10.1128/mbio.02260-23
- Sewunet T, Asrat D, Woldeamanuel Y, et al. High prevalence of bla CTX-M-15 and nosocomial transmission of hypervirulent epidemic clones of Klebsiella pneumoniae at a tertiary hospital in Ethiopia. JAC Antimicrob Resist. 2021;3(1):dlab001. doi: 10.1093/jacamr/dlab001
- Keshri V, Panda A, Levasseur A, Rolain JM, Pontarotti P, Raoult D. Phylogenomic analysis of β-lactamase in Archaea and bacteria enables the identification of putative new members. Genome Biol Evol. 2018;10(4):1106-1114. doi: 10.1093/gbe/evy028
- Ebmeyer S, Kristiansson E, Larsson DGJ. A framework for identifying the recent origins of mobile antibiotic resistance genes. Commun Biol. 2021;4(1):8. doi: 10.1038/s42003-020-01545-5
- Shawa M, Furuta Y, Mulenga G, et al. Novel chromosomal insertions of ISEcp1-blaCTX-M-15 and diverse antimicrobial resistance genes in Zambian clinical isolates of Enterobacter cloacae and Escherichia coli. Antimicrob Resist Infect Control. 2021;10(1):79. doi: 10.1186/s13756-021-00941-8
- Hussain HI, Aqib AI, Seleem MN, et al. Genetic basis of molecular mechanisms in β-lactam resistant gram-negative bacteria. Microb Pathog. 2021;158:105040. doi: 10.1016/j.micpath.2021.105040
- Sabbagh P, Rajabnia M, Maali A, Ferdosi-Shahandashti E. Integron and its role in antimicrobial resistance: A literature review on some bacterial pathogens. Iran J Basic Med Sci. 2021;24(2):136-142. doi: 10.22038/ijbms.2020.48905.11208
- El Aila NA, Al Laham NA, Ayesh BM. Prevalence of extended spectrum beta lactamase and molecular detection of blaTEM, blaSHV and blaCTX-M genotypes among Gram negative bacilli isolates from pediatric patient population in Gaza strip. BMC Infect Dis. 2023;23(1):99. doi: 10.1186/s12879-023-08017-1
- Nordmann P, Naas T, Poirel L. Global spread of carbapenemase-producing Enterobacteriaceae. Emerg Infect Dis. 2011;17(10):1791-1798. doi: 10.3201/eid1710.110655
- Pitout JDD, Peirano G, Kock MM, Strydom KA, Matsumura Y. The global ascendency of OXA-48-type carbapenemases. Clin Microbiol Rev. 2019;33(1):e00102-19. doi: 10.1128/cmr.00102-19
- Nordmann P, Poirel L. The difficult-to-control spread of carbapenemase producers among Enterobacteriaceae worldwide. Clin Microbiol Infect. 2014;20(9):821-830. doi: 10.1111/1469-0691.12719
- Graham DW, Knapp CW, Christensen BT, McCluskey S, Dolfing J. Appearance of β-lactam resistance genes in agricultural soils and clinical isolates over the 20th century. Sci Rep. 2016;6(1):21550. doi: 10.1038/srep21550
- Navarro A, Sanseverino I, Cappelli F, et al. Study of antibiotic resistance in freshwater ecosystems with low anthropogenic impact. Sci Total Environ. 2023;857:159378. doi: 10.1016/j.scitotenv.2022.159378
- Husna A, Rahman MM, Badruzzaman ATM, et al. Extended-spectrum β-lactamases (ESBL): Challenges and opportunities. Biomedicines. 2023;11(11):2937. doi: 10.3390/biomedicines11112937
- Caliskan-Aydogan O, Alocilja EC. A review of carbapenem resistance in Enterobacterales and its detection techniques. Microorganisms. 2023;11(6):1491. doi: 10.3390/microorganisms11061491
- Muniesa M, García A, Miró E, et al. Bacteriophages and diffusion of β-lactamase genes. Emerg Infect Dis. 2004;10(6):1134-1137. doi: 10.3201/eid1006.030472
- Bennett PM. Plasmid encoded antibiotic resistance: Acquisition and transfer of antibiotic resistance genes in bacteria. Br J Pharmacol. 2008;153(S1):S347-S357. doi: 10.1038/sj.bjp.0707607
- Kaderabkova N, Bharathwaj M, Furniss RCD, Gonzalez D, Palmer T, Mavridou DAI. The biogenesis of β-lactamase enzymes. Microbiology (Reading). 2022;168(8):001217. doi: 10.1099/mic.0.001217
- Bradford PA. Extended-spectrum β-lactamases in the 21st century: Characterization, epidemiology, and detection of this important resistance threat. Clin Microbiol Rev. 2001;14(4):933-951. doi: 10.1128/cmr.14.4.933-951.2001
- Glen KA, Lamont IL. β-lactam Resistance in Pseudomonas aeruginosa: Current status, future prospects. Pathogens. 2021;10(12):1638. doi: 10.3390/pathogens10121638
- Singh A, Rani K, Tandon V, Sahoo AK, Samanta SK. Ag NCs as a potent antibiofilm agent against pathogenic Pseudomonas aeruginosa and Acinetobacter baumannii and drug-resistant Bacillus subtilis by affecting chemotaxis and flagellar assembly pathway genes. Biomater Sci. 2022;10(23):6778-6790. doi: 10.1039/d2bm01399a
- Feria C. Patterns and mechanisms of resistance to beta-lactams and beta-lactamase inhibitors in uropathogenic Escherichia coli isolated from dogs in Portugal. J Antimicrob Chemother. 2002;49(1):77-85. doi: 10.1093/jac/49.1.77
- Bodendoerfer E, Marchesi M, Imkamp F, Courvalin P, Böttger EC, Mancini S. Co-occurrence of aminoglycoside and β-lactam resistance mechanisms in aminoglycoside- non-susceptible Escherichia coli isolated in the Zurich area, Switzerland. Int J Antimicrob Agents. 2020;56(1):106019. doi: 10.1016/j.ijantimicag.2020.106019
- Kyriakidis I, Vasileiou E, Pana ZD, Tragiannidis A. Acinetobacter baumannii antibiotic resistance mechanisms. Pathogens. 2021;10(3):373. doi: 10.3390/pathogens10030373
- Kwon NY, Kim JD, Pai HJ. The resistance mechanisms of b-lactam antimicrobials in clinical isolates of Acinetobacter baumannii. Korean J Intern Med. 2002;17(2):94-99. doi: 10.3904/kjim.2002.17.2.94
- Scoffone VC, Trespidi G, Barbieri G, Arshad A, Israyilova A, Buroni S. The evolution of antimicrobial resistance in Acinetobacter baumannii and new strategies to fight it. Antibiotics (Basel). 2025;14(1):85. doi: 10.3390/antibiotics14010085
- Wu HJ, Xiao ZG, Lv XJ, et al. Drug-resistant Acinetobacter baumannii: From molecular mechanisms to potential therapeutics (Review). Exp Ther Med. 2023;25(5):209. doi: 10.3892/etm.2023.11908
- Medeiros AA. Cooperative evolution of mechanisms of β-lactam resistance. Clin Microbiol Infect. 2000;6:27-33. doi: 10.1111/j.1469-0691.2000.tb02037.x
- Rajer F, Allander L, Karlsson PA, Sandegren L. Evolutionary trajectories toward high-level β-lactam/β-lactamase inhibitor resistance in the presence of multiple β-lactamases. Antimicrob Agents Chemother. 2022;66(6):e0029022. doi: 10.1128/aac.00290-22
- Zhu Y, Gu J, Zhao Z, et al. Deciphering the coevolutionary dynamics of L2 β-lactamases via deep learning. J Chem Inf Model. 2024;64(9):3706-3717. doi: 10.1021/acs.jcim.4c00189
- Salahuddin P, Khan AU. Studies on structure-based sequence alignment and phylogenies of beta-lactamases. Bioinformation. 2014;10(5):308-313. doi: 10.6026/97320630010308
- Brandt K, Barrangou R. Phylogenetic analysis of the Bifidobacterium genus using glycolysis enzyme sequences. Front Microbiol. 2016;7:657.doi: 10.3389/fmicb.2016.00657
- Zhao WH, Hu ZQ. IMP-type metallo-β-lactamases in gram-negative bacilli: Distribution, phylogeny, and association with integrons. Crit Rev Microbiol. 2011;37(3):214-226. doi: 10.3109/1040841x.2011.559944
- Martínez-García L, González-Alba JM, Baquero F, Cantón R, Galán JC. Ceftazidime is the key diversification and selection driver of VIM-type carbapenemases. mBio. 2018;9(3):e02109-17. doi: 10.1128/mbio.02109-17
- Acman M, Wang R, van Dorp L, et al. Role of mobile genetic elements in the global dissemination of the carbapenem resistance gene blaNDM. Nat Commun. 2022;13(1):1131. doi: 10.1038/s41467-022-28819-2
- Abdul-Mutakabbir JC, Nguyen L, Maassen P, et al. 1297. In-vitro antibacterial activities of cefiderocol (S-649266) alone and with the addition of beta-lactamase inhibitors against multidrug-resistant Acinetobacter baumannii. Open Forum Infect Dis. 2020;7(Supplement_1):S663. doi: 10.1093/ofid/ofaa439.1480
- Dubey V, Farrington N, Harper N, et al. Acinetobacter baumannii transformants expressing oxacillinases and metallo-β-lactamases that confer resistance to meropenem: New tools for anti- Acinetobacter drug development and AMR preparedness. Antimicrob Agents Chemother. 2024;68(10):e0022224. doi: 10.1128/aac.00222-24
- Mentasti M, Prime K, Sands K, Khan S, Wootton M. Rapid detection of OXA-23-like, OXA-24-like, and OXA-58-like carbapenemases from Acinetobacter species by real-time PCR. J Hosp Infect. 2020;105(4):741-746. doi: 10.1016/j.jhin.2020.06.015
- Karakonstantis S, Kritsotakis EI, Gikas A. Treatment options for K. pneumoniae, P. aeruginosa and A. baumannii co-resistant to carbapenems, aminoglycosides, polymyxins and tigecycline: An approach based on the mechanisms of resistance to carbapenems. Infection. 2020;48(6):835-851. doi: 10.1007/s15010-020-01520-6
- Denkel LA, Gastmeier P. Gut microbiome and its role in the acquisition of extended-spectrum β-lactamase-producing enterobacterales. Clin Microbiol Infect. 2024;30(9):1102-1104. doi: 10.1016/j.cmi.2024.05.020
- Imkamp F, Kolesnik-Goldmann N, Bodendoerfer E, Zbinden R, Mancini S. Detection of extended-spectrum β-lactamases (ESBLs) and AmpC in class A and class B carbapenemase-producing enterobacterales. Microbiol Spectr. 2022;10(6):e0213722. doi: 10.1128/spectrum.02137-22
- Benavides JA, Salgado-Caxito M, Opazo-Capurro A, et al. ESBL-producing Escherichia coli carrying CTX-M genes circulating among livestock, dogs, and wild mammals in small-scale farms of central Chile. Antibiotics (Basel). 2021;10(5):510. doi: 10.3390/antibiotics10050510
- Higgins O, Chueiri A, O’Connor L, et al. Portable differential detection of CTX-M ESBL gene variants, blaCTX-M-1 and blaCTX-M-15, from Escherichia coli isolates and animal fecal samples using loop-primer endonuclease cleavage loop-mediated isothermal amplification. Microbiol Spectr. 2023;11(1):e0331622. doi: 10.1128/spectrum.03316-22
- Schotte U, Ehlers J, Nieter J, et al. ESBL-type and AmpC-type beta-lactamases in third generation cephalosporin-resistant Enterobacterales isolated from animal feces in Madagascar. Animals (Basel). 2024;14(5):741. doi: 10.3390/ani14050741
- Shen J, Long X, Jiang Q, et al. Genomic characterization of a vancomycin-resistant strain of Enterococcus faecium harboring a rep2 plasmid. Infect Drug Resist. 2023;16:1153-1158. doi: 10.2147/idr.s398913
- Mascini EM, Jansze M, Schouls LM, Verhoef J, Van Dijk H. Penicillin and clindamycin differentially inhibit the production of pyrogenic exotoxins A and B by group A streptococci. Int J Antimicrob Agents. 2001;18(4):395-398. doi: 10.1016/s0924-8579(01)00413-7
- Berg BR, Houseman JL, terSteeg ZE, LeBar WD, Newton DW. Antimicrobial susceptibilities of group B Streptococcus isolates from prenatal screening samples. J Clin Microbiol. 2014;52(9):3499-3500. doi: 10.1128/jcm.01781-14
- Farrell DJ, Morrissey I, Bakker S, Buckridge S, Felmingham D. Global distribution of TEM-1 and ROB-1 β-lactamases in Haemophilus influenzae. J Antimicrob Chemother. 2005;56(4):773-776. doi: 10.1093/jac/dki281
- Haemophilus influenzae Infections Treatment and Management. 2024. Available from: https://emedicine. medscape.com/article/218271-treatment?form=fpf [Last accessed on 2025 Mar 03].
- Conradie F, Bagdasaryan TR, Borisov S, et al. Bedaquiline-pretomanid-linezolid regimens for drug-resistant tuberculosis. N Engl J Med. 2022;387(9):810-823. doi: 10.1056/nejmoa2119430
- Elsener TA, Jolley KA, Sanders E, Maiden MCJ, Cehovin A, Tang CM. There are three major Neisseria gonorrhoeae β-lactamase plasmid variants which are associated with specific lineages and carry distinct TEM alleles. Microb Genom. 2023;9(7):mgen001057. doi: 10.1099/mgen.0.001057
- Lin EY, Adamson PC, Klausner JD. Epidemiology, treatments, and vaccine development for antimicrobial-resistant Neisseria gonorrhoeae: Current strategies and future directions. Drugs. 2021;81(10):1153-1169. doi: 10.1007/s40265-021-01530-0
- Wen SCH, Best E, Nourse C. Non‐typhoidal Salmonella infections in children: Review of literature and recommendations for management. J Paediatr Child Health. 2017;53(10):936-941. doi: 10.1111/jpc.13585
- Halat DH, Ayoub Moubareck C. The intriguing carbapenemases of Pseudomonas aeruginosa: Current status, genetic profile, and global epidemiology. Yale J Biol Med. 2022;95:507-515.
- Chatham-Stephens K, Medalla F, Hughes M, et al. Emergence of extensively drug-resistant Salmonella typhi infections among travelers to or from Pakistan - United States, 2016- 2018. MMWR Morb Mortal Wkly Rep. 2019;68(1):11-13. doi: 10.15585/mmwr.mm6801a3
- Christopher PRH, David KV, John SM, Sankarapandian V. Antibiotic therapy for Shigella dysentery. Cochrane Database Syst Rev. 2010;2010(8):CD006784. doi: 10.1002/14651858.cd006784.pub4
- Voladri RK, Tummuru MK, Kernodle DS. Structure-function relationships among wild-type variants of Staphylococcus aureus beta-lactamase: Importance of amino acids 128 and 216. J Bacteriol. 1996;178(24):7248-7253. doi: 10.1128/jb.178.24.7248-7253.1996
- Majhi A, Adhikary R, Bhattacharyya A, Mahanti S, Bishayi B. Levofloxacin-ceftriaxone combination attenuates lung inflammation in a mouse model of bacteremic pneumonia caused by multidrug-resistant Streptococcus pneumoniae via inhibition of cytolytic activities of pneumolysin and autolysin. Antimicrob Agents Chemother. 2014;58(9):5164-5180. doi: 10.1128/aac.03245-14
- Mei H, Arbeithuber B, Cremona MA, DeGiorgio M, Nekrutenko A. A high-resolution view of adaptive event dynamics in a Plasmid. Genome Biol Evol. 2019; 11(10):3022-3034. doi: 10.1093/gbe/evz197
- Bedhomme S, Perez Pantoja D, Bravo IG. Plasmid and clonal interference during post horizontal gene transfer evolution. Mol Ecol. 2017;26(7):1832-1847. doi: 10.1111/mec.14056