In silico mutation analysis of the SARS-CoV-2 Spike glycoprotein in the Omicron (B.1.1.529) variant isolated from the Iraqi patients
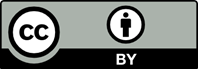
Since its first breakout in December 2019, the severe acute respiratory syndrome coronavirus 2 (SARS-CoV-2) has impacted the lives of millions of people worldwide. The virus has been rapidly mutating, and the accumulation of various mutations has precipitated the emergence of several new variants. The Omicron variant (B.1.1.529 lineage) was first identified in Botswana and South Africa back in November 2021. Since then, several Omicron sub-lineages have emerged as a result of hypermutations. In this study, a computational analysis of the 381 spike glycoprotein (S protein) of the SARS-CoV-2 Omicron variants isolated from Iraqi patients was performed. The full-length S protein sequences (1273 amino acids) were obtained from the publicly accessible Global Initiative on Sharing All Influenza Data database. A total of 60 mutation sites were recognized: 49 substitution sites, ten deletions, and one insertion. K417N and N440K were the most prevalent mutations (n = 379, 99.4%), followed by G339D (n = 377, 98.9%) and S373P and S375F (both n = 367, 96.3%). Both BA.1.1 (n = 198, 52%) and BA.1 (n = 91, 14%) were the predominant variant types encountered throughout this study. The current work offers the data of SARS-CoV-2 Omicron variants derived from the Iraqi patients. The data from this study could assist in the molecular design of more potent vaccines and/or antiviral drugs against the virus and also provide a fundamental understanding of SARS-CoV-2 evolution with concerns about their pathogenicity.
- Coronaviridae Study Group of the International Committee on Taxonomy of Viruses, 2020, The species Severe acute respiratory syndrome-related coronavirus: Classifying 2019- nCoV and naming it SARS-CoV-2. Nat Microbiol, 5: 536–544. https://doi.org/10.1038/s41564-020-0695-z
- Zhou P, Yang XL, Wang XG, et al., 2020, A pneumonia outbreak associated with a new coronavirus of probable bat origin. Nature, 579: 270–273. https://doi.org/10.1038/s41586-020-2012-7
- Waris A, Atta UK, Ali M, et al., 2020, COVID-19 outbreak: Current scenario of Pakistan. New Microbes New Infect, 35: 100681. https://doi.org/10.1016/j.nmni.2020.100681
- Waris A, Ali M, Khan AU, et al., 2020, COVID-19 incidence in Pakistan: Gender disparity. Iran J Psychiatry Behav Sci, 14: e105990.
- World Health Organization, 2023, COVID-19 Weekly Epidemiological Update. Geneva: World Health Organization.
- World Health Organization, 2022, World Health Organization (WHO) Coronavirus (COVID-19) Dashboard. World Health Organization. Available from: https://covid19who.int [Last accessed on 2022 Feb 28].
- Alsayed R, Ali A, Makia R, et al., 2020, Challenges facing Iraq to tackle the spread of COVID-19: An overview. J Univ Anbar Pure Sci, 14: 22–27.
- Fayad N, Abi Habib W, Kandeil A, et al., 2021, SARS-CoV-2 variants in Lebanon: Evolution and current situation. Biology (Basel), 10: 531. https://doi.org/10.3390/biology10060531
- Kim D, Lee JY, Yang JS, et al., 2020, The architecture of SARS-CoV-2 transcriptome. Cell, 181: 914–921.e10. https://doi.org/10.1016/j.cell.2020.04.011
- Wang R, Hozumi Y, Yin C, et al., 2020, Mutations on COVID-19 diagnostic targets. Genomics, 112: 5204–5213. https://doi.org/10.1016/j.ygeno.2020.09.028
- Sabir DK, 2022, Analysis of SARS-COV2 spike protein variants among Iraqi isolates. Gene Rep, 26: 101420. https://doi.org/10.1016/j.genrep.2021.101420
- Yang H, Rao Z, 2021, Structural biology of SARS-CoV-2 and implications for therapeutic development. Nat Rev Microbiol, 19: 685–700. https://doi.org/10.1038/s41579-021-00630-8
- Tilli CMLJ, Van Steensel MA, Krekels GA, et al., 2005, Molecular aetiology and pathogenesis of basal cell carcinoma. Br J Dermatol, 152: 1108–1124. https://doi.org/10.1111/j.1365-2133.2005.06587.x
- Brüssow H, 2022, COVID-19: Omicron-the latest, the least virulent, but probably not the last variant of concern of SARS-CoV-2. Microb Biotechnol, 15: 1927–1939. https://doi.org/10.1111/1751-7915.14064
- Lauring AS, Hodcroft E, 2021, Genetic variants of SARS-CoV-2-what do they mean? JAMA, 325: 529–531. https://doi.org/10.1001/jama.2020.27124
- Ledford H, 2021, How severe are Omicron infections. Nature, 600: 577–578. https://doi.org/10.1038/d41586-021-03794-8
- Burki TK, 2022, Omicron variant and booster COVID-19 vaccines. Lancet Respir Med, 10: e17. https://doi.org/10.1016/S2213-2600(21)00559-2
- da Silva SJR, Kohl A, Pena L, et al., 2022, Recent insights into SARS-CoV-2 omicron variant. Rev Med Virol, 33: e2373. https://doi.org/10.1002/rmv.2373
- Zamel D, Khan AU, 2022, Covid-19 vaccines: Overview, comparative analysis and dynamics. Acta Sci Biotechnol Vol, 3: 24–33.
- Karim SSA, Karim QA, 2021, Omicron SARS-CoV-2 variant: A new chapter in the COVID-19 pandemic. Lancet, 398: 2126–2128. https://doi.org/10.1016/S0140-6736(21)02758-6
- Sun J, Xie T, Jamal M, et al., 2020, CLEC3B as a potential diagnostic and prognostic biomarker in lung cancer and association with the immune microenvironment. Cancer Cell Int, 20: 106. https://doi.org/10.1186/s12935-020-01183-1
- Hulo C, de Castro E, Masson P, et al., 2011, ViralZone: A knowledge resource to understand virus diversity. Nucleic Acids Res, 39: D576–D582. https://doi.org/10.1093/nar/gkq901
- Hadfield J, Megill C, Bell SM, et al., 2018, Nextstrain: Real-time tracking of pathogen evolution. Bioinformatics, 34: 4121–4123. https://doi.org/10.1093/bioinformatics/bty407
- Worldometers.info. Available from: https://www. worldometers.info/coronavirus/country/iraq [Last accessed on 2023 May 31].
- Sabir DK, Khwarahm NR, Ali SM, et al., 2020, Children protection against COVID-19 at the pandemic outbreak. J Immunol Sci, 4: 8–12. https://doi.org/10.29245/2578-3009/2020/2.1188
- Zhou Y, Zhi H, Teng Y, 2023, The outbreak of SARS-CoV-2 Omicron lineages, immune escape, and vaccine effectivity. J Med Virol, 95: e28138. https://doi.org/10.1002/jmv.28138
- Tian D, Sun Y, Xu H, et al., 2022, The emergence and epidemic characteristics of the highly mutated SARS-CoV-2 Omicron variant. J Med Virol, 94: 2376–2383. https://doi.org/10.1002/jmv.27643
- Majumdar S, Sarkar R, 2022, Mutational and phylogenetic analyses of the two lineages of the Omicron variant. J Med Virol, 94: 1777–1779. https://doi.org/10.1002/jmv.27558
- Xia S, Wang L, Zhu Y, et al., 2022, Origin, virological features, immune evasion and intervention of SARS-CoV-2 Omicron sublineages. Signal Transduct Target Ther, 7: 241.
- Abdullah HM, Ali SM, Sabir DK, 2020, Data suggesting that COVID-19 may have existed in the kurdistan region of Iraq at the time of the outbreak in wuhan province of China. J Kermanshah Univ Med Sci, 24: e105758.
- Shrestha LB, Foster C, Rawlinson W, et al., 2022, Evolution of the SARS-CoV-2 omicron variants BA. 1 to BA. 5: Implications for immune escape and transmission. Rev Med Virol, 32: e2381. https://doi.org/10.1002/rmv.2381
- Markov PV, Ghafari M, Beer M, et al., 2023, The evolution of SARS-CoV-2. Nat Rev Microbiol, 21: 361–379. https://doi.org/10.1038/s41579-023-00878-2
- He Y, Ma W, Dang S, et al., 2022, Possible recombination between two variants of concern in a COVID-19 patient. Emerg Microbes Infect, 11: 552–555. https://doi.org/10.1080/22221751.2022.2032375
- Balakrishnan KN, Yew CW, Chong ET, et al., 2023, Timeline of SARS-CoV-2 transmission in Sabah, Malaysia: Tracking the molecular evolution. Pathogens, 12: 1047. https://doi.org/10.3390/pathogens12081047
- Fan Y, Li X, Zhang L, et al., 2022, SARS-CoV-2 Omicron variant: Recent progress and future perspectives. Signal Transduct Target Ther, 7: 141. https://doi.org/10.1038/s41392-022-00997-x
- West AP Jr., Wertheim JO, Wang JC, et al., 2021, Detection and characterization of the SARS-CoV-2 lineage B.1.526 in New York. Nat Commun, 12: 4886. https://doi.org/10.1038/s41467-021-25168-4
- Klinakis A, Cournia Z, Rampias T, 2021, N-terminal domain mutations of the spike protein are structurally implicated in epitope recognition in emerging SARS-CoV-2 strains. Comput Struct Biotechnol J, 19: 5556–5567. https://doi.org/10.1016/j.csbj.2021.10.004
- Suryadevara N, Shrihari S, Gilchuk P, et al., 2021, Neutralizing and protective human monoclonal antibodies recognizing the N-terminal domain of the SARS-CoV-2 spike protein. Cell, 184: 2316–2331.e15. https://doi.org/10.1016/j.cell.2021.03.029
- Meng B, Kemp SA, Papa G, et al., 2021, Recurrent emergence of SARS-CoV-2 spike deletion H69/V70 and its role in the Alpha variant B.1.1.7. Cell Rep, 35: 109292. https://doi.org/10.1016/j.celrep.2021.109292
- Hong Q, Han W, Li J, et al., 2022, Molecular basis of receptor binding and antibody neutralization of Omicron. Nature, 604: 546–552. https://doi.org/10.1038/s41586-022-04581-9
- Cao Y, Yisimayi A, Jian F, et al., 2022, BA.2.12.1, BA.4 and BA.5 escape antibodies elicited by Omicron infection. Nature, 608: 593–602. https://doi.org/10.1038/s41586-022-04980-y
- Barton MI, MacGowan SA, Kutuzov MA, et al., 2021, Effects of common mutations in the SARS-CoV-2 Spike RBD and its ligand, the human ACE2 receptor on binding affinity and kinetics. Elife, 10: e70658. https://doi.org/10.7554/eLife.70658
- Cao Y, Wang J, Jian F, et al., 2022, Omicron escapes the majority of existing SARS-CoV-2 neutralizing antibodies. Nature, 602: 657–663. https://doi.org/10.1038/s41586-021-04385-3
- Verma S, Patil VM, Gupta MK, 2022, Mutation informatics: SARS-CoV-2 receptor-binding domain of the spike protein. Drug Discov Today, 27: 103312. https://doi.org/10.1016/j.drudis.2022.06.012
- Kumar S, Thambiraja TS, Karuppanan K, et al., 2022, Omicron and Delta variant of SARS-CoV-2: A comparative computational study of spike protein. J Med Virol, 94: 1641–1649. https://doi.org/10.1002/jmv.27526
- Ali F, Kasry A, Amin M, 2021, The new SARS-CoV-2 strain shows a stronger binding affinity to ACE2 due to N501Y mutant. Med Drug Discov, 10: 100086. https://doi.org/10.1016/j.medidd.2021.100086
- Quarleri J, Galvan V, Delpino MV, 2022, Omicron Variant of the SARS-CoV-2: A Quest to Define the Consequences of its High Mutational Load. Berlin: Springer. pp1–4.
- Chakraborty C, Saha A, Sharma AR, et al., 2021, D614G mutation eventuates in all VOI and VOC in SARS-CoV-2: Is it part of the positive selection pioneered by Darwin? Mol Ther Nucleic Acids, 26: 237–241. https://doi.org/10.1016/j.omtn.2021.07.011
- Mittal A, Khattri A, Verma V, 2022, Structural and antigenic variations in the spike protein of emerging SARS-CoV-2 variants. PLoS Pathog, 18: e1010260. https://doi.org/10.1371/journal.ppat.1010260
- Tian D, Sun Y, Zhou J, et al., 2021, The global epidemic of the SARS-CoV-2 Delta variant, key spike mutations and immune escape. Front Immunol, 12: 751778. https://doi.org/10.3389/fimmu.2021.751778
- Jackson CB, Zhang L, Farzan M, et al., 2021, Functional importance of the D614G mutation in the SARS-CoV-2 spike protein. Biochem Biophys Res Commun, 538: 108–115. https://doi.org/10.1016/j.bbrc.2020.11.026
- Wang C, Zheng Y, Niu Z, 2021, The virological impacts of SARS-CoV-2 D614G mutation. J Mol Cell Biol, 13: 712–720. https://doi.org/10.1093/jmcb/mjab045
- Ozono S, Zhang Y, Ode H, et al., 2021, SARS-CoV-2 D614G spike mutation increases entry efficiency with enhanced ACE2-binding affinity. Nat Commun, 12: 848. https://doi.org/10.1038/s41467-021-21118-2
- Lubinski B, Fernandes MHV, Frazier L, et al., 2022, Functional evaluation of the P681H mutation on the proteolytic activation of the SARS-CoV-2 variant B.1.1.7 (Alpha) spike. iScience, 25: 103589.
- Saito A, Irie T, Suzuki R, et al., 2022, Enhanced fusogenicity and pathogenicity of SARS-CoV-2 Delta P681R mutation. Nature, 602: 300–306. https://doi.org/10.1038/s41586-021-04266-9
- Xia S, Liu M, Wang C, et al., 2020, Inhibition of SARS-CoV-2 (previously 2019-nCoV) infection by a highly potent pan-coronavirus fusion inhibitor targeting its spike protein that harbors a high capacity to mediate membrane fusion. Cell Res, 30: 343–355. https://doi.org/10.1038/s41422-020-0305-x