Analysis of multi-disease targeting effect of phytochemicals by AMPK stimulation– diabetes: A computational approach
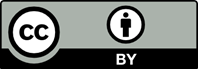
Diabetes is a silent killer and a metabolic syndrome characterized by hyperglycemia that has been exponentially increasing in recent years. There is a need to develop therapeutic agents to control hyperglycemia and its secondary complications as well as protect and revive beta cells in diabetic patients. The target for first-line diabetes treatment is the adenosine monophosphate protein kinase (AMPK), which participates in cellular energy metabolism through phosphorylation of metabolic enzymes and transcription regulators. This study examined the drug-related properties as well as lead preparation of Catharanthus roseus alkaloids and testing molecular interaction at the AMPK targets to confirm their anti-diabetic effect. A control drug metformin and a library of 85 molecules of C. roseus alkaloids were crossed with the ADMET test, followed by the investigation of molecular interaction tested on AMPK1 and AMPK2 targets through an in silico docking process. Vindolinine (CID: 24148538), vindoline (CID: 425978), (+)-vindorosine (CID: 261578), Cr-1 (CID: 5315746), and Cr-2 (CID: 59908094) had passed the ADMET test. Molecular interaction of the tested C. roseus alkaloids on AMPK1 and AMPK2 targets had potential energy that varied from −7.4 to −5.3 kcal/mol, whereas binding energies of −4.0 kcal/mol for AMPK1-metformin interaction and −4.2 kcal/mol for AMPK2-metformin interaction were observed. The tested C. roseus alkaloids were shown to be more potent activators of AMPK than the control drug. All five biomolecules of C. roseus acted as modulators that have the potential to stimulate AMPK, reduce glucose production, and increase glucose utilization in hepatocytes. In addition, they diminished insulin resistance and secondary complications of diabetes by inhibiting acetyl-CoA carboxylase, regulating cholesterol levels and macrophage, and reviving beta cells in Type 2 diabetes. These results provided the foundation for developing new multi-disease-targeting drugs that can treat diabetes, obesity, cardiovascular disease, cancer, and other diseases by the stimulation of AMPK1 and AMPK2 targets.
- Tripathi KD, 2010, Essentials of Medical Pharmacology. 6th ed. India: Jaypee Publication.
- Hall JE, Guyton AC, 2011, Guyton and Hall Textbook of Medical Physiology. 12th ed. USA: Saunders Elsevier Publication.
- Kasper DL, Fauci AS, Longo DL, et al., 2015, Harrison’s Principles of Internal Medicine. 19th ed. USA: McGraw Hill Publication.
- Saeedi P, Petersohn I, Salpea P, et al., 2019, Global and regional diabetes prevalence estimates for 2019 and projections for 2030 and 2045: Results from the International Diabetes Federation Diabetes Atlas, 9th edition. Diabetes Res Clin Pract, 157: 107843. https://doi.org/10.1016/j.diabres.2019.107843
- Singh U, 2016, Prevalence of diabetes and other health related problems across India and worldwide: An overview. J Appl Nat Sci, 8(1): 500–505. https://doi.org/10.31018/jans.v8i1.825
- Proença C, Ribeiro D, Freitas M, et al., 2021, Flavonoids as potential agents in the management of Type 2 diabetes through the modulation of α-amylase and α-glucosidase activity: A review. Crit Rev Food Sci Nutr, 62: 3137–3207. https://doi.org/10.1080/10408398.2020.1862755
- Kashtoh H, Baek KH, 2022, Recent updates on phytoconstituent alpha-glucosidase inhibitors: An approach towards the treatment of type two diabetes. Plants (Basel), 11: 2722. https://doi.org/10.3390/plants11202722
- Lebovitz HE, 1997, Alpha-glucosidase inhibitors. Endocrinol Metab Clin North Am, 26(3): 539–551. https://doi.org/10.1016/s0889-8529(05)70266-8
- Alam S, Sarker MM, Sultana TN, et al., 2022, Antidiabetic phytochemicals from medicinal plants: Prospective candidates for new drug discovery and development. Front Endocrinol (Lausanne), 13: 800714. https://doi.org/10.3389/fendo.2022.800714
- Woods A, Salt I, Scott J, et al., 1996, The alpha1 and alpha2 isoforms of the AMP-activated protein kinase have similar activities in rat liver but exhibit differences in substrate specificity in vitro. FEBS Lett, 397: 347–351. https://doi.org/10.1016/s0014-5793(96)01209-4
- Wang YG, Han XG, Yang Y, et al., 2016, Functional differences between AMPK α1 and α2 subunits in osteogenesis, osteoblast-associated induction of osteoclastogenesis, and adipogenesis. Sci Rep, 6: 32771. https://doi.org/10.1038/srep32771
- PRKAA1 Protein Kinase AMPK-activated Catalytic Subunit Alpha 1 [Homo sapiens (Human)]. Available from: https://www.ncbi.nlm.nih.gov [Last accessed on 2023 Apr 15].
- Da Silva Xavier G, Leclerc I, Varadi A, et al., 2003, Role for AMP-activated protein kinase in glucose-stimulated insulin secretion and preproinsulin gene expression. Biochem J, 371(3): 761–774. https://doi.org/10.1042/bj20021812
- Trillo-Tinoco J, Sierra RA, Mohamed E, et al., 2019, AMPK alpha-1 intrinsically regulates the function and differentiation of tumor myeloid-derived suppressor cells. Cancer Res, 79(19): 5034–5047. https://doi.org/10.1158/0008-5472.CAN-19-0880
- Mounier R, Lantier L, Viollet B, et al., 2009, Important role for AMPKalpha1 in limiting skeletal muscle cell hypertrophy. FASEB J, 23(7): 2264–2273. https://doi.org/10.1096/fj.08-119057
- Phair IR, Nisr RB, Viollet B, et al., 2023, AMPK integrates metabolite and kinase-based immunometabolic control in macrophages. Mol Metab, 68: 101661. https://doi.org/10.1016/j.molmet.2022.101661
- UniProt Consortium, 2021, UniProt: The universal protein knowledgebase in 2021. Nucl Acids Res, 49: D480–D489. https://doi.org/10.1093/nar/gkaa1100
- PRKAA2 Protein Kinase AMP-activated Catalytic Subunit Alpha2 [Homo sapiens (Human)]. Available from: https://www.ncbi.nlm.nih.gov [Last accessed on 2023 Apr 15].
- Foretz M, Guigas B, Bertrand L, et al., 2014, Metformin: From mechanisms of action to therapies. Cell Metab, 20(2): 953–966. https://doi.org/10.1016/j.cmet.2014.09.018
- Foretz M, Guigas B, Viollet B, 2019, Understanding the glucoregulatory mechanisms of metformin in Type 2 diabetes mellitus. Nat Rev Endocrinol, 15(10): 569–589. https://doi.org/10.1038/s41574-019-0242-2
- Ohadoma SC, Akpan JL, Odey PA, et al., 2021, Mechanistic considerations of Catharanthus roseus on the hypoglycemic activity of alpha glucosidase inhibitors and biguanides: A review. J Pharm Adv Res, 4(10): 1390–1398.
- Francini F, Schinella GR, Ríos JL, 2019, Activation of AMPK by medicinal plants and natural products: Its role in Type 2 diabetes mellitus. Mini Rev Med Chem, 19(11): 880–901. https://doi.org/10.2174/1389557519666181128120726
- Srivastava V, Yadav A, Sarkar P, 2022, Molecular docking and ADMET study of bioactive compounds of Glycyrrhiza glabra against main protease of SARS-CoV2. Mater Today Proc, 49: 2999–3007. https://doi.org/10.1016/j.matpr.2020.10.055
- Adhikari B, 2021, Roles of alkaloids from medicinal plants in the management of diabetes mellitus. J Chem, 10: 2691525. https://doi.org/10.1155/2021/2691525
- Bennouna J, Delord JP, Campone M, et al., 2008, Vinflunine: A new microtubule inhibitor agent. Clin Cancer Res, 14: 1625–1632. https://doi.org/10.1158/1078-0432.CCR-07-2219
- Newman DJ, Cragg GM, 2007, Natural products as sources of new drugs over the last 25 years. J Nat Prod, 70(3): 461–477. https://doi.org/10.1021/np068054v
- Balaji H, 2014, Versatile therapeutic effects of Vinca rosea linn. Int J Pharm Sci Health Care, 1(4): 56–76.
- Mohan SC, Anand T, Priyadharshini GS, et al., 2015, GC-MS analysis of phytochemicals and hypoglycemic effect of Catharanthus roseus in alloxan-induced diabetic rats. Int J Pharm Sci Rev Res, 31(1): 123–128.
- Tolambiya P, Mathur S, 2016, A study on potential phytopharmaceuticals assets in Catharanthus roseus L. (Alba). Int J Life Sci Biotechnol Pharm Res, 5: 1–6. https://doi.org/10.18178/ijlbpr.5.1.1-6
- Nisar A, Mamat AS, Hatim MI, et al., 2016, An updated review on Catharanthus roseus: Phytochemical and pharmacological analysis. Indian Res J Pharm Sci, 9: 631–653.
- John J, 2017, Evaluation of hypoglycemic effect of Aloe vera on allaxon induced diabetic rats. Int J Inf Res Rev, 4(3): 3865–3868.
- Kalhotra P, Chittepu CS, Osorio-Revilla G, et al., 2020, Phytochemicals in garlic extract inhibit therapeutic enzyme DPP-4 and induce skeletal muscle cell proliferation: A possible mechanism of action to benefit the treatment of diabetes mellitus. Biomolecules, 10: 305. https://doi.org/10.3390/biom10020305
- Bharadwaj S, Dubey A, Yadava U, et al., 2021, Exploration of natural compounds with anti-SARS-CoV-2 activity via inhibition of SARS-CoV-2 Mpro. Brief Bioinform, 22(2): 1361–1377. https://doi.org/10.1093/bib/bbaa382
- Yousif E, Sherif R, Abeer AE, et al., 2021, Vinca (Catharanthus roseus) extracts attenuate alloxan-induced hyperglycemia and oxidative stress in journal rats. Am Food Sci Technol, 9(4): 161–172. https://doi.org/10.12691/ajfst-9-4-8
- Schwede T, Kopp J, Guex N, et al., 2003, SWISS-MODEL: An automated protein homology-modeling server. Nucleic Acids Res, 31(13): 3381–3385. https://doi.org/10.1093/nar/gkg520
- Gasteiger E, Hoogland C, Gattiker A, et al., 2005, Protein identification and analysis tools on the expasy server. In: Walker JM, editor. The Proteomics Protocols Handbook. United States: Humana Press. p571-607. https://doi.org/10.1385/1-59259-890-0:571
- Geourjon C, Deléage G, 1995, SOPMA: Significant improvements in protein secondary structure prediction by consensus prediction from multiple alignments. Comput Appl Biosci, 11(6): 681–684. https://doi.org/10.1093/bioinformatics/11.6.681
- Hirokawa T, Boon-Chieng S, Mitaku S, 1998, SOSUI: Classification and secondary structure prediction system for membrane proteins. Bioinformatics, 14(4): 378–379. https://doi.org/10.1093/bioinformatics/14.4.378
- Hollingsworth SA, Karplus PA, 2010, A fresh look at the Ramachandran plot and the occurrence of standard structures in proteins. Biomol Concepts, 1(3–4): 271–283. https://doi.org/10.1515/BMC.2010.022
- Binkowski TA, Naghibzadeh S, Liang J, 2003, CASTp: Computed atlas of surface topography of proteins. Nucleic Acids Res, 31(13): 3352–3335. https://doi.org/10.1093/nar/gkg512
- Guex N, Peitsch MC, 1997, SWISS-MODEL and the Swiss- PdbViewer: An environment for comparative protein modeling. Electrophoresis, 18: 2714–2723. https://doi.org/10.1002/elps.1150181505
- Pettersen EF, Goddard TD, Huang CC, et al., 2004, UCSF Chimera--a visualization system for exploratory research and analysis. J Comput Chem, 25(13): 1605–1612. https://doi.org/10.1002/jcc.20084
- Eberhardt J, Santos-Martins D, Tillack AF, et al., 2021, AutoDock Vina 1.2.0: New docking methods, expanded force field, and python bindings. J Chem Inf Model, 61: 3891–3898. https://doi.org/10.1021/acs.jcim.1c00203
- Tiong SH, Looi CY, Hazni H, et al., 2013, Antidiabetic and antioxidant properties of alkaloids from Catharanthus roseus (L.) G. Don. Molecules, 18: 9770–9784. https://doi.org/10.3390/molecules18089770
- O’Boyle NM, Banck M, James CA, et al., 2011, Open babel: An open chemical toolbox. J Cheminform, 3: 33. https://doi.org/10.1186/1758-2946-3-33
- Sander T, 2001, OSIRIS Property Explorer. Switzerland: Organic Chemistry Portal. Available from: https://www.organic-chemistry.org/prog/peo [Last accessed on 2022 Dec 25].
- Bickerton GR, Paolini GV, Besnard J, et al., 2012, Quantifying the chemical beauty of drugs. Nat Chem, 4: 90–98. https://doi.org/10.1038/nchem.1243
- Hanwell MD, Curtis DE, Lonie DC, et al., 2012, Avogadro: An advanced semantic chemical editor, visualization, and analysis platform. J Cheminform, 4: 17. https://doi.org/10.1186/1758-2946-4-17
- BIOVIA, Dassault Systems, 2021, BIOVIA Discovery Studio Visualizer 4.5, 21.1. San Diego: Dassault System. Available from: https://biovia-discovery-studio-2021-client.software. informer.com [Last accessed on 2023 Jan 02].
- Kim S, Chen J, Cheng T, et al., 2021, PubChem in 2021: New data content and improved web interfaces. Nucleic Acids Res, 49(D1): D1388–D1395. https://doi.org/10.1093/nar/gkaa971
- Sharma B, Mittal A, Dabur R, 2018, Mechanistic approach of anti-diabetic compounds identified from natural sources. Chem Biol Lett, 5(2): 63–99.
- Stapleton D, Mitchelhill KI, Gao G, et al., 1996, Mammalian AMP-activated protein kinase subfamily. J Biol Chem, 271(2): 611–614. https://doi.org/10.1074/jbc.271.2.611
- Kim J, Yang G, Kim Y, et al., 2016, AMPK activators: Mechanisms of action and physiological activities. Exp Mol Med, 48: e224. https://doi.org/10.1038/emm.2016.16
- Tarasiuk O, Miceli M, Di Domizio A, et al., 2022, AMPK and diseases: State of the art regulation by AMPK-targeting molecules. Biology (Basel), 11: 1041. https://doi.org/10.3390/biology11071041
- Coughlan KA, Valentine RJ, Ruderman NB, et al., 2014, AMPK activation: A therapeutic target for Type 2 diabetes? Diabetes Metab Syndr Obes, 7: 241–253. https://doi.org/10.2147/DMSO.S43731
- Jensen TE, Schjerling P, Viollet B, et al., 2008, AMPK α1 activation is required for stimulation of glucose uptake by twitch contraction, but not by H2O2, in mouse skeletal muscle. PLoS One, 3(5): e2102. https://doi.org/10.1371/journal.pone.0002102
- Sertel S, Fu Y, Zu Y, et al., 2011, Molecular docking and pharmacogenomics of Vinca alkaloids and their monomeric precursors, vindoline and catharanthine. Biochem Pharmacol, 81(6): 723–735. https://doi.org/10.1016/j.bcp.2010.12.026
- Goboza M, Meyer M, Aboua YG, et al., 2020, In vitro antidiabetic and antioxidant effects of different extracts of Catharanthus roseus and its indole alkaloid, vindoline. Molecules, 25: 5546. https://doi.org/10.3390/molecules25235546
- Goyal R, Kumar M, Mallick MA, 2023, Drug Innovation Studies Targeting Diabetes: A Computational Docking Approach on Muti-drug Targets Including COVID Inhibitors. North Carolina: Research Square. https://doi.org/10.21203/rs.3.rs-2457415/v1
- Goboza M, Aboua YG, Chegou N, et al., 2019, Vindoline effectively ameliorated diabetes-induced hepatotoxicity by docking oxidative stress, inflammation and hypertriglyceridemia in Type 2 diabetes-induced male Wistar rats. Biomed Pharmacother, 112: 108638. https://doi.org/10.1016/j.biopha.2019.108638
- Heidary MR, Samimi Z, Asgary S, et al., 2022, Natural AMPK activators in cardiovascular disease prevention. Front Pharmacol, 12: 738420. https://doi.org/10.3389/fphar.2021.738420
- Foretz M, Even PC, Viollet B, 2018, AMPK activation reduces hepatic lipid content by increasing fat oxidation in vivo. Int J Mol Sci, 19: 2826. https://doi.org/10.3390/ijms19092826
- Zhou G, Myers R, Li Y, et al., 2001, Role of AMP-activated protein kinase in mechanism of metformin action. J Clin Invest, 108: 1167–1174. https://doi.org/10.1172/JCI13505
- Habegger KM, Hoffman NJ, Ridenour CM, et al., 2012, AMPK enhances insulin-stimulated GLUT4 regulation via lowering membrane cholesterol. Endocrinology, 153(5): 2130–2141. https://doi.org/10.1210/en.2011-2099
- Oguntibeju OO, Aboua Y, Goboza M, 2019, Vindoline-a natural product from Catharanthus roseus reduces hyperlipidemia and renal pathophysiology in experimental Type 2 diabetes. Biomedicines, 7: 59. https://doi.org/10.3390/biomedicines7030059
- Mishra JN, Verma NK, 2017, A brief study on Catharanthus roseus: A review. Int J Res Pharm Pharm Sci, 2(2): 20–23.
- Sujatha S, Alhaji NM, 2022, Mechanism of action of alkaloids in the management of diabetes mellitus. J Adv Sci Res, 13(3): 4–7. https://doi.org/10.55218/JASR.202213302