Gene-modified T cell therapy for cancer: Current challenges and potential solutions
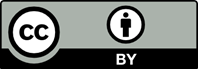
Immunotherapy has achieved significant breakthroughs in patients with hematological diseases and various cancers. The adoptive transfer of T cells, especially gene-modified T cells such as chimeric antigen receptor T (CAR-T) cells and T cell receptor-engineered T (TCR-T) cells, is developing rapidly. Since 2017, eight CAR-T cell products have been approved for the treatment of hematological diseases, such as refractory or relapsed acute B lymphoblastic leukemia, specific subtypes of B cell lymphoma, and multiple myeloma. The first TCR-T cell product, Kimmtrak, was approved for the treatment of unresectable or metastatic uveal melanoma in March 2022. However, there are still many problems, including side effects, relapse, high demand for individualization, and expensive cost in hematological diseases, tumor heterogeneity, antigen escape, poor immune cell infiltration ability, immunosuppressive microenvironment, and low response in solid tumors. With the in-depth exploration of tumor immunology and the development of genetic engineering technology, many novel strategies for improving the anti-tumor effect and safety of gene-modified T cell immunotherapy have been attempted. This paper presents a systematic review of the literature on CAR-T cell and TCR-T cell therapy, focusing on applications, clinical trials, problems, and potential solutions.
Neelapu SS, Dickinson M, Munoz J, et al., 2022, Axicabtagene ciloleucel as first-line therapy in high-risk large B-cell lymphoma: The phase 2 ZUMA-12 trial. Nat Med, 28(4): 735–742. https://doi.org/10.1038/s41591-022-01731-4
Locke FL, Miklos DB, Jacobson CA, et al., 2022, Axicabtagene ciloleucel as second-line therapy for large B-cell lymphoma. N Engl J Med, 386(7): 640–654. https://doi.org/10.1056/NEJMoa2116133
Mullard A, 2021, FDA approves first BCMA-targeted CAR-T cell therapy. Nat Rev Drug Discov, 20(5): 332. https://doi.org/10.1038/d41573-021-00063-1
Greenbaum U, Dumbrava EI, Biter AB, et al., 2021, Engineered T-cell receptor T cells for cancer immunotherapy. Cancer Immunol Res, 9(11): 1252–1261. https://doi.org/10.1158 / 2326-6066.CIR-21-0269
Robbins PF, Morgan RA, Feldman SA, et al., 2011, Tumor regression in patients with metastatic synovial cell sarcoma and melanoma using genetically engineered lymphocytes reactive with NY-ESO-1. J Clin Oncol, 29(7): 917–924. https://doi.org/10.1200/JCO.2010.32.2537
Schank TE, Hassel JC, 2022, Tebentafusp for the treatment of metastatic uveal melanoma. Future Oncol, 18(11): 1303–1311. https://doi.org/10.2217/fon-2021-1260
Ecsedi M, McAfee MS, Chapuis AG, 2021, The anticancer potential of T cell receptor-engineered T cells. Trends Cancer, 7(1): 48–56. https://doi.org/10.1016/j.trecan.2020.09.002
Raes L, De Smedt SC, Raemdonck K, et al., 2021, Non-viral transfection technologies for next-generation therapeutic T cell engineering. Biotechnol Adv, 49: 107760. https://doi.org/10.1016/j.biotechadv.2021.107760
Bailey SR, Maus MV, 2019, Gene editing for immune cell therapies. Nat Biotechnol, 37(12): 1425–1434. https://doi.org/10.1038/s41587-019-0137-8
Kuwana Y, Asakura Y, Utsunomiya N, et al., 1987, Expression of chimeric receptor composed of immunoglobulin-derived V regions and T-cell receptor-derived C regions. Biochem Biophys Res Commun, 149(3): 960–968. https://doi.org/10.1016 / 0006-291x(87)90502-x
Wilkins O, Keeler AM, Flotte TR, 2017, CAR T-Cell therapy: Progress and prospects. Hum Gene Ther Methods, 28(2): 61–66. https://doi.org/10.1089/hgtb.2016.153
Kochenderfer JN, Feldman SA, Zhao Y, et al., 2009, Construction and preclinical evaluation of an anti-CD19 chimeric antigen receptor. J Immunother, 32(7): 689–702. https://doi.org/10.1097/CJI.0b013e3181ac6138
Zhang C, Liu J, Zhong JF, et al., 2017, Engineering CAR-T cells. Biomark Res, 5: 22. https://doi.org/10.1186/s40364-017-0102-y
Jacobson CA, Chavez JC, Sehgal AR, et al., 2022, Axicabtagene ciloleucel in relapsed or refractory indolent non-Hodgkin lymphoma (ZUMA-5): A single-arm, multicentre, phase 2 trial. Lancet Oncol, 23(1): 91–103. https://doi.org/10.1016/S1470-2045(21)00591-X
Sterner RC, Sterner RM, 2021, CAR-T cell therapy: Current limitations and potential strategies. Blood Cancer J, 11(4): 69.
Guercio M, Manni S, Boffa I, et al., 2021, Inclusion of the inducible caspase 9 suicide gene in CAR construct increases safety of CAR.CD19 T cell therapy in B-Cell malignancies. Front Immunol, 12: 755639. https://doi.org/10.3389/fimmu.2021.755639
Spiegel JY, Patel S, Muffly L, et al., 2021, CAR T cells with dual targeting of CD19 and CD22 in adult patients with recurrent or refractory B cell malignancies: A phase 1 trial. Nat Med, 27(8): 1419–1431. https://doi.org/10.1038/s41591-021-01436-0
van der Schans JJ, van de Donk N, Mutis T, 2020, Dual targeting to overcome current challenges in multiple myeloma CAR T-cell treatment. Front Oncol, 10: 1362. https://doi.org/10.3389/fonc.2020.01362
Huang R, Li X, He Y, et al., 2020, Recent advances in CAR-T cell engineering. J Hematol Oncol, 13(1): 86. https://doi.org/10.1186/s13045-020-00910-5
Shah NN, Lee DW, Yates B, et al., 2021, Long-term follow-up of CD19-CAR T-cell therapy in children and young adults with B-ALL. J Clin Oncol, 39(15): 1650–1659. https://doi.org/10.1200/JCO.20.02262
Chong EA, Ruella M, Schuster SJ, 2021, Lymphoma program investigators at the university of P: Five-year outcomes for refractory B-Cell lymphomas with CAR T-Cell therapy. N Engl J Med, 384(7): 673–674. https://doi.org/10.1056/NEJMc2030164
Wang M, Munoz J, Goy A, et al., 2020, KTE-X19 CAR T-cell therapy in relapsed or refractory mantle-cell lymphoma. N Engl J Med, 382(14): 1331–1342. https://doi.org/10.1056/NEJMoa1914347
Ramos CA, Grover NS, Beaven AW, et al., 2020, Anti-CD30 CAR-T cell therapy in relapsed and refractory hodgkin lymphoma. J Clin Oncol, 38(32): 3794–3804. https://doi.org/10.1200/JCO.20.01342
Raje N, Berdeja J, Lin Y, et al., 2019, Anti-BCMA CAR T-cell therapy bb2121 in relapsed or refractory multiple myeloma. N Engl J Med, 380(18): 1726–1737. https://doi.org/10.1056/NEJMoa1817226
Neelapu SS, Tummala S, Kebriaei P, et al., 2018, Chimeric antigen receptor T-cell therapy assessment and management of toxicities. Nat Rev Clin Oncol, 15(1): 47–62. https://doi.org/10.1038/nrclinonc.2017.148
Grover P, Veilleux O, Tian L, et al., 2022, Chimeric antigen receptor T-cell therapy in adults with B-cell acute lymphoblastic leukemia. Blood Adv, 6(5): 1608–1618. https://doi.org/10.1182/bloodadvances.2020003482
Shah BD, Bishop MR, Oluwole OO, et al., 2021, KTE-X19 anti-CD19 CAR T-cell therapy in adult relapsed/refractory acute lymphoblastic leukemia: ZUMA-3 phase 1 results. Blood, 138(1): 11–22. https://doi.org/10.1182/blood.2020009098
Samur MK, Fulciniti M, Samur AA, et al., 2021, Biallelic loss of BCMA as a resistance mechanism to CAR T cell therapy in a patient with multiple myeloma. Nat Commun, 12(1): 868. https://doi.org/10.1038/s41467-021-21177-5
Shah NN, Fry TJ, 2019, Mechanisms of resistance to CAR T cell therapy. Nat Rev Clin Oncol, 16(6): 372–385. https://doi.org/10.1038/s41571-019-0184-6
Brudno JN, Kochenderfer JN, 2019, Recent advances in CAR T-cell toxicity: Mechanisms, manifestations and management. Blood Rev, 34: 45–55. https://doi.org/10.1016/j.blre.2018.11.002
Maude SL, Frey N, Shaw PA, et al., 2014, Chimeric antigen receptor T cells for sustained remissions in leukemia. N Engl J Med, 371(16): 1507–1517. https://doi.org/10.1056/NEJMoa1407222
Gust J, Hay KA, Hanafi LA, et al., 2017, Endothelial activation and blood-brain barrier disruption in neurotoxicity after adoptive immunotherapy with CD19 CAR-T cells. Cancer Discov, 7(12): 1404–1419. https://doi.org/10.1158 / 2159-8290.CD-17-0698
Giavridis T, van der Stegen SJ, Eyquem J, et al., 2018, CAR T cell-induced cytokine release syndrome is mediated by macrophages and abated by IL-1 blockade. Nat Med, 24(6): 731–738. https://doi.org/10.1038/s41591-018-0041-7
Fischer JW, Bhattarai N, 2021, CAR-T cell therapy: Mechanism, management, and mitigation of inflammatory toxicities. Front Immunol, 12: 693016. https://doi.org/10.3389/fimmu.2021.693016
Chen X, Li X, Liu Y, et al., 2020, A phase I clinical trial of chimeric antigen receptor-modified T cells in patients with relapsed and refractory lymphoma. Immunotherapy, 12(10): 681–696. https://doi.org/10.2217/imt-2020-0022
Norelli M, Camisa B, Barbiera G, et al., 2018, Monocyte-derived IL-1 and IL-6 are differentially required for cytokine-release syndrome and neurotoxicity due to CAR T cells. Nat Med, 24(6): 739–748. https://doi.org/10.1038/s41591-018-0036-4
Staedtke V, Bai RY, Kim K, et al., 2018, Disruption of a self-amplifying catecholamine loop reduces cytokine release syndrome. Nature, 564(7735): 273–277. https://doi.org/10.1038/s41586-018-0774-y
Liu Y, Fang Y, Chen X, et al., 2020, Gasdermin E-mediated target cell pyroptosis by CAR T cells triggers cytokine release syndrome. Sci Immunol, 5(43): eaax7969. https://doi.org/10.1126/sciimmunol.aax7969
Liu Y, Chen X, Wang D, et al., 2018, Hemofiltration successfully eliminates severe cytokine release syndrome following CD19 CAR-T-cell therapy. J Immunother, 41(9): 406–410. https://doi.org/10.1097/CJI.0000000000000243
Xiao X, He X, Li Q, et al., 2019, Plasma exchange can be an alternative therapeutic modality for severe cytokine release syndrome after chimeric antigen receptor-T cell infusion: A case report. Clin Cancer Res, 25(1): 29–34. https://doi.org/10.1158 / 1078-0432.CCR-18-1379
Huarte E, O’Connor RS, Peel MT, et al., 2020, Itacitinib (INCB039110), a JAK1 inhibitor, reduces cytokines associated with cytokine release syndrome induced by CAR T-cell therapy. Clin Cancer Res, 26(23): 6299–6309. https://doi.org/10.1158 / 1078-0432.CCR-20-1739
Casucci M, Falcone L, Camisa B, et al., 2018, Extracellular NGFR spacers allow efficient tracking and enrichment of fully functional CAR-T cells co-expressing a suicide gene. Front Immunol, 9: 507. https://doi.org/10.3389/fimmu.2018.00507
Ying Z, Huang XF, Xiang X, et al., 2019, A safe and potent anti-CD19 CAR T cell therapy. Nat Med, 25(6): 947–953. https://doi.org/10.1038/s41591-019-0421-7
Brudno JN, Lam N, Vanasse D, et al., 2020, Safety and feasibility of anti-CD19 CAR T cells with fully human binding domains in patients with B-cell lymphoma. Nat Med, 26(2): 270–280. https://doi.org/10.1038/s41591-019-0737-3
Fraietta JA, Lacey SF, Orlando EJ, et al., 2018, Determinants of response and resistance to CD19 chimeric antigen receptor (CAR) T cell therapy of chronic lymphocytic leukemia. Nat Med, 24(5): 563–571. https://doi.org/10.1038/s41591-018-0010-1
Deng Q, Han G, Puebla-Osorio N, et al., 2020, Characteristics of anti-CD19 CAR T cell infusion products associated with efficacy and toxicity in patients with large B cell lymphomas. Nat Med, 26(12): 1878–1887. https://doi.org/10.1038/s41591-020-1061-7
Wang X, Brea LT, Yu J, 2019, Immune modulatory functions of EZH2 in the tumor microenvironment: Implications in cancer immunotherapy. Am J Clin Exp Urol, 7(2): 85–91.
John LB, Devaud C, Duong CP, et al., 2013, Anti-PD-1 antibody therapy potently enhances the eradication of established tumors by gene-modified T cells. Clin Cancer Res, 19(20): 5636–5646. https://doi.org/10.1158 / 1078-0432.CCR-13-0458
Choi BD, Yu X, Castano AP, et al., 2019, CRISPR-Cas9 disruption of PD-1 enhances activity of universal EGFRvIII CAR T cells in a preclinical model of human glioblastoma. J Immunother Cancer, 7(1): 304. https://doi.org/10.1186/s40425-019-0806-7
Chong EA, Alanio C, Svoboda J, et al., 2022, Pembrolizumab for B-cell lymphomas relapsing after or refractory to CD19-directed CAR T-cell therapy. Blood, 139(7): 1026–1038. https://doi.org/10.1182/blood.2021012634
Liu M, Wang X, Li Z, et al., 2020, Synergistic effect of ibrutinib and CD19 CAR-T cells on Raji cells in vivo and in vitro. Cancer Sci, 111(11): 4051–4060. https://doi.org/10.1111/cas.14638
Liu M, Deng H, Mu J, et al., 2021, Ibrutinib improves the efficacy of anti-CD19-CAR T-cell therapy in patients with refractory non-Hodgkin lymphoma. Cancer Sci, 112(7): 2642–2651. https://doi.org/10.1111/cas.14915
Prinzing B, Zebley CC, Petersen CT, et al., 2021, Deleting DNMT3A in CAR T cells prevents exhaustion and enhances antitumor activity. Sci Transl Med, 13(620): eabh0272. https://doi.org/10.1126/scitranslmed.abh0272
Wang Y, Tong C, Dai H, et al., 2021, Low-dose decitabine priming endows CAR T cells with enhanced and persistent antitumour potential via epigenetic reprogramming. Nat Commun, 12(1): 409. https://doi.org/10.1038/s41467-020-20696-x
Lynn RC, Weber EW, Sotillo E, et al., 2019, c-Jun overexpression in CAR T cells induces exhaustion resistance. Nature, 576(7786): 293–300. https://doi.org/10.1038/s41586-019-1805-z
Seo H, Gonzalez-Avalos E, Zhang W, et al., 2021, BATF and IRF4 cooperate to counter exhaustion in tumor-infiltrating CAR T cells. Nat Immunol, 22(8): 983–995. https://doi.org/10.1038/s41590-021-00964-8
Tong C, Zhang Y, Liu Y, et al., 2020, Optimized tandem CD19/CD20 CAR-engineered T cells in refractory/relapsed B-cell lymphoma. Blood, 136(14): 1632–1644. https://doi.org/10.1182/blood.2020005278
Wei G, Zhang Y, Zhao H, et al., 2021, CD19/CD22 dual-targeted CAR T-cell therapy for relapsed/refractory aggressive B-cell lymphoma: A safety and efficacy study. Cancer Immunol Res, 9(9): 1061–1070. https://doi.org/10.1158 / 2326-6066.CIR-20-0675
Cordoba S, Onuoha S, Thomas S, et al., 2021, CAR T cells with dual targeting of CD19 and CD22 in pediatric and young adult patients with relapsed or refractory B cell acute lymphoblastic leukemia: A phase 1 trial. Nat Med, 27(10): 1797–1805. https://doi.org/10.1038/s41591-021-01497-1
Tu S, Zhou X, Guo Z, et al., 2019, CD19 and CD70 dual-target chimeric antigen receptor T-cell therapy for the treatment of relapsed and refractory primary central nervous system diffuse large B-cell lymphoma. Front Oncol, 9: 1350. https://doi.org/10.3389/fonc.2019.01350
Kang L, Zhang J, Li M, et al., 2020, Characterization of novel dual tandem CD19/BCMA chimeric antigen receptor T cells to potentially treat multiple myeloma. Biomark Res, 8: 14. https://doi.org/10.1186/s40364-020-00192-6
Mei H, Li C, Jiang H, et al., 2021, A bispecific CAR-T cell therapy targeting BCMA and CD38 in relapsed or refractory multiple myeloma. J Hematol Oncol, 14(1): 161. https://doi.org/10.1186/s13045-021-01170-7
Hegde M, Mukherjee M, Grada Z, et al., 2021, Tandem CAR T cells targeting HER2 and IL13Ralpha2 mitigate tumor antigen escape. J Clin Invest, 131(13): e152477. https://doi.org/10.1172/JCI152477
Zhao J, Lin Q, Song Y, et al., 2018, Universal CARs, universal T cells, and universal CAR T cells. J Hematol Oncol, 11(1): 132. https://doi.org/10.1186/s13045-018-0677-2
Tang TCY, Xu N, Nordon R, et al., 2022, Donor T cells for CAR T cell therapy. Biomark Res, 10(1): 14. https://doi.org/10.1186/s40364-022-00359-3
Lin H, Cheng J, Mu W, et al., 2021, Advances in universal CAR-T cell therapy. Front Immunol, 12: 744823. https://doi.org/10.3389/fimmu.2021.744823
Torikai H, Reik A, Liu PQ, et al., 2012, A foundation for universal T-cell based immunotherapy: T cells engineered to express a CD19-specific chimeric-antigen-receptor and eliminate expression of endogenous TCR. Blood, 119(24): 5697–5705. https://doi.org/10.1182/blood-2012-01-405365
Qasim W, Zhan H, Samarasinghe S, et al., 2017, Erratum for the report: Molecular remission of infant B-ALL after infusion of universal TALEN gene-edited CAR T cells. Sci Transl Med, 9: aam9292. https://doi.org/10.1126/scitranslmed.aam9292
Hu Y, Zhou Y, Zhang M, et al., 2021, CRISPR/Cas9- engineered universal CD19/CD22 dual-targeted CAR-T cell therapy for relapsed/refractory B-cell acute lymphoblastic leukemia. Clin Cancer Res, 27(10): 2764–2772. https://doi.org/10.1158 / 1078-0432.CCR-20-3863
Benjamin R, Graham C, Yallop D, et al., 2020, Genome-edited, donor-derived allogeneic anti-CD19 chimeric antigen receptor T cells in paediatric and adult B-cell acute lymphoblastic leukaemia: results of two phase 1 studies. Lancet, 396(10266): 1885–1894. https://doi.org/10.1016/S0140-6736(20)32334-5
Rafiq S, Hackett CS, Brentjens RJ, 2020, Engineering strategies to overcome the current roadblocks in CAR T cell therapy. Nat Rev Clin Oncol, 17(3): 147–167. https://doi.org/10.1038/s41571-019-0297-y
Shi D, Shi Y, Kaseb AO, et al., 2020, Chimeric antigen receptor-glypican-3 T-cell therapy for advanced hepatocellular carcinoma: Results of phase I trials. Clin Cancer Res, 26(15): 3979–3989. https://doi.org/10.1158 / 1078-0432.CCR-19-3259
Beatty GL, O’Hara MH, Lacey SF, et al., 2018, Activity of mesothelin-specific chimeric antigen receptor T cells against pancreatic carcinoma metastases in a phase 1 trial. Gastroenterology, 155(1): 29–32. https://doi.org/10.1053/j.gastro.2018.03.029
Liu Y, Guo Y, Wu Z, et al., 2020, Anti-EGFR chimeric antigen receptor-modified T cells in metastatic pancreatic carcinoma: A phase I clinical trial. Cytotherapy, 22(10): 573–580. https://doi.org/10.1016/j.jcyt.2020.04.088
Qi C, Gong J, Li J, et al., 2022, Claudin18.2-specific CAR T cells in gastrointestinal cancers: phase 1 trial interim results. Nat Med, 28(6): 1189–1198. https://doi.org/10.1038/s41591-022-01800-8
Zhang C, Wang Z, Yang Z, et al., 2017, Phase I escalating-dose trial of CAR-T therapy targeting CEA(+) metastatic colorectal cancers. Mol Ther, 25(5): 1248–1258. https://doi.org/10.1016/j.ymthe.2017.03.010
O’Rourke DM, Nasrallah MP, Desai A, et al., 2017, A single dose of peripherally infused EGFRvIII-directed CAR T cells mediates antigen loss and induces adaptive resistance in patients with recurrent glioblastoma. Sci Transl Med, 9(399): eaaa0984. https://doi.org/10.1126/scitranslmed.aaa0984
Capsomidis A, Benthall G, Van Acker HH, et al., 2018, Chimeric antigen receptor-engineered human gamma delta T cells: Enhanced cytotoxicity with retention of cross presentation. Mol Ther, 26(2): 354–365. https://doi.org/10.1016/j.ymthe.2017.12.001
Wang Y, Wang J, Yang X, et al., 2021, Chemokine receptor CCR2b enhanced anti-tumor function of chimeric antigen receptor T cells targeting mesothelin in a non-small-cell lung carcinoma model. Front Immunol, 12: 628906. https://doi.org/10.3389/fimmu.2021.628906
Li G, Zhang Q, Han Z, et al., 2021, IL-7 and CCR2b Co-expression-mediated enhanced CAR-T survival and infiltration in solid tumors. Front Oncol, 11: 734593. https://doi.org/10.3389/fonc.2021.734593
Liu G, Rui W, Zheng H, et al., 2020, CXCR2-modified CAR-T cells have enhanced trafficking ability that improves treatment of hepatocellular carcinoma. Eur J Immunol, 50(5): 712–724. https://doi.org/10.1002/eji.201948457
Adachi K, Kano Y, Nagai T, et al., 2018, IL-7 and CCL19 expression in CAR-T cells improves immune cell infiltration and CAR-T cell survival in the tumor. Nat Biotechnol, 36(4): 346–351. https://doi.org/10.1038/nbt.4086
Pang N, Shi J, Qin L, et al., 2021, IL-7 and CCL19-secreting CAR-T cell therapy for tumors with positive glypican-3 or mesothelin. J Hematol Oncol, 14(1): 118. https://doi.org/10.1186/s13045-021-01128-9
Hou AJ, Chen LC, Chen YY, 2021, Navigating CAR-T cells through the solid-tumour microenvironment. Nat Rev Drug Discov, 20(7): 531–550. https://doi.org/10.1038/s41573-021-00189-2
Tian Y, Li Y, Shao Y, et al., 2020, Gene modification strategies for next-generation CAR T cells against solid cancers. J Hematol Oncol, 13(1): 54.
Liu G, Rui W, Zhao X, et al., 2021, Enhancing CAR-T cell efficacy in solid tumors by targeting the tumor microenvironment. Cell Mol Immunol, 18(5): 1085–1095. https://doi.org/10.1038/s41423-021-00655-2
Shi R, Tang YQ, Miao H, 2020, Metabolism in tumor microenvironment: Implications for cancer immunotherapy. MedComm (2020), 1(1): 47–68. https://doi.org/10.1002/mco2.6
Chmielewski M, Kopecky C, Hombach AA, et al., 2011, IL-12 release by engineered T cells expressing chimeric antigen receptors can effectively Muster an antigen-independent macrophage response on tumor cells that have shut down tumor antigen expression. Cancer Res, 71(17): 5697–5706. https://doi.org/10.1158 / 0008-5472.CAN-11-0103
Hu B, Ren J, Luo Y, et al., 2017, Augmentation of Antitumor Immunity by Human and Mouse CAR T Cells Secreting IL-18. Cell Rep, 20(13): 3025–3033. https://doi.org/10.1016/j.celrep.2017.09.002
Ma X, Shou P, Smith C, et al., 2020, Interleukin-23 engineering improves CAR T cell function in solid tumors. Nat Biotechnol, 38(4): 448–459. https://doi.org/10.1038/s41587-019-0398-2
Mariathasan S, Turley SJ, Nickles D, et al., 2018, TGFbeta attenuates tumour response to PD-L1 blockade by contributing to exclusion of T cells. Nature, 554(7693): 544– 548. https://doi.org/10.1038/nature25501
Chen X, Wang L, Li P, et al., 2018, Dual TGF-beta and PD-1 blockade synergistically enhances MAGE-A3-specific CD8(+) T cell response in esophageal squamous cell carcinoma. Int J Cancer, 143(10): 2561–2574. https://doi.org/10.1002/ijc.31730
Tang N, Cheng C, Zhang X, et al., 2020, TGF-beta inhibition via CRISPR promotes the long-term efficacy of CAR T cells against solid tumors. JCI Insight, 5(4): e133977. https://doi.org/10.1172/jci.insight.133977
Narayan V, Barber-Rotenberg JS, Jung IY, et al., 2022, PSMA-targeting TGFbeta-insensitive armored CAR T cells in metastatic castration-resistant prostate cancer: A phase 1 trial. Nat Med, 28(4): 724–734. https://doi.org/10.1038/s41591-022-01726-1
Rodriguez-Garcia A, Lynn RC, Poussin M, et al., 2021, CAR-T cell-mediated depletion of immunosuppressive tumor-associated macrophages promotes endogenous antitumor immunity and augments adoptive immunotherapy. Nat Commun, 12(1): 877. https://doi.org/10.1038/s41467-021-20893-2
Ping Y, Liu C, Zhang Y, 2018, T-cell receptor-engineered T cells for cancer treatment: current status and future directions. Protein Cell, 9(3): 254–266. https://doi.org/10.1007/s13238-016-0367-1
Zhao Q, Jiang Y, Xiang S, et al., 2021, Engineered TCR-T cell immunotherapy in anticancer precision medicine: Pros and cons. Front Immunol, 12: 658753. https://doi.org/10.3389/fimmu.2021.658753
Shafer P, Kelly LM, Hoyos V, 2022, Cancer therapy with TCR-engineered T cells: Current strategies, challenges, and prospects. Front Immunol, 13: 835762. https://doi.org/10.3389/fimmu.2022.835762
Chen X, Wang L, Yue D, et al., 2017, Correlation between the high expression levels of cancer-germline genes with clinical characteristics in esophageal squamous cell carcinoma. Histol Histopathol, 32(8): 793–803. https://doi.org/10.14670/HH-11-847
Liu Y, Yan X, Zhang F, et al., 2021, TCR-T immunotherapy: The challenges and solutions. Front Oncol, 11: 794183. https://doi.org/10.3389/fonc.2021.794183
Kageyama S, Ikeda H, Miyahara Y, et al., 2015, Adoptive transfer of MAGE-A4 T-cell receptor gene-transduced lymphocytes in patients with recurrent esophageal cancer. Clin Cancer Res, 21(10): 2268–2277. https://doi.org/10.1158 / 1078-0432.CCR-14-1559
Morgan RA, Chinnasamy N, Abate-Daga D, et al., 2013, Cancer regression and neurological toxicity following anti- MAGE-A3 TCR gene therapy. J Immunother, 36(2): 133–151. https://doi.org/10.1097/CJI.0b013e3182829903
Linette GP, Stadtmauer EA, Maus MV, et al., 2013, Cardiovascular toxicity and titin cross-reactivity of affinity-enhanced T cells in myeloma and melanoma. Blood, 122(6): 863–871. https://doi.org/10.1182/blood-2013-03-490565
Kirtane K, Elmariah H, Chung CH, et al., 2021, Adoptive cellular therapy in solid tumor malignancies: Review of the literature and challenges ahead. J Immunother Cancer, 9(7): e002723. https://doi.org/10.1136/jitc-2021-002723
Wei F, Cheng XX, Xue JZ, et al., 2022, Emerging strategies in TCR-engineered T cells. Front Immunol, 13: 850358. https://doi.org/10.3389/fimmu.2022.850358
Chandran SS, Klebanoff CA, 2019, T cell receptor-based cancer immunotherapy: Emerging efficacy and pathways of resistance. Immunol Rev, 290(1): 127–147. https://doi.org/10.1111/imr.12772
Ahmadzadeh M, Johnson LA, Heemskerk B, et al., 2009, Tumor antigen-specific CD8 T cells infiltrating the tumor express high levels of PD-1 and are functionally impaired. Blood, 114(8): 1537–1544. https://doi.org/10.1182/blood-2008-12-195792
Tokunaga Y, Sasaki T, Goto S, et al., 2022, Enhanced antitumor responses of tumor antigen-specific TCR T cells genetically engineered to produce IL7 and CCL19. Mol Cancer Ther, 21(1): 138–148. https://doi.org/10.1158 / 1535-7163.MCT-21-0400
Sailer N, Fetzer I, Salvermoser M, et al., 2022, T-Cells expressing a highly potent PRAME-specific T-cell receptor in combination with a chimeric PD1-41BB co-stimulatory receptor show a favorable preclinical safety profile and strong anti-tumor reactivity. Cancers (Basel), 14(8): 1998. https://doi.org/10.3390/cancers14081998
Hu Z, Anandappa AJ, Sun J, et al., 2018, A cloning and expression system to probe T-cell receptor specificity and assess functional avidity to neoantigens. Blood, 132(18): 1911–1921. https://doi.org/10.1182/blood-2018-04-843763