Melt electrospinning writing PCL scaffolds after alkaline modification with outstanding cytocompatibility and osteoinduction
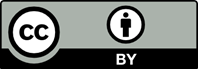
Melt electrospinning writing (MEW) is a promising three-dimensional (3D) printing technology that enables the creation of scaffolds with highly ordered microfibers. Polycaprolactone (PCL) is an ideal material for MEW scaffold fabrication due to its exceptional printability. However, its low cellular affinity can hinder its performance in bone tissue engineering. This study aimed to explore the potential of NaOH treatment as a means of enhancing the cytocompatibility and osteoinductive properties of PCL scaffolds. After modification with a NaOH solution, the physiochemical properties of the MEW PCL scaffold were analyzed. The surface of the scaffold was found to have nanopits and nanogrooves, which differed from the smooth surface of the PCL scaffold. Atomic force microscopy and automatic water contact angle assays revealed an increase in surface roughness and wettability, both of which were found to be beneficial for cell proliferation and adhesion. In vitro experiments demonstrated that the NaOH-treated surface was able to induce osteogenic differentiation of rat bone marrow mesenchymal stem cells (BMSCs) via the integrinα2/β1-PI3K-Akt signaling pathway, which had not been previously observed. The study involved implanting PCL scaffold to repair a cranial defect. After 1 and 3 months of implantation, histological analysis and micro-computed tomography scans showed a higher amount of newly formed bone on the NaOH-treated PCL scaffolds compared to the PCL scaffold. The study concluded that NaOH treatment was a simple and effective way to enhance cellular affinity and osteoinductive property of MEW PCL scaffold. This strategy may provide a cost-efficient method for promoting bone regeneration.
- Maruyama T, Stevens R, Boka A, et al., 2021, BMPR1A maintains skeletal stem cell properties in craniofacial development and craniosynostosis. Sci Transl Med, 13(583): eabb4416. https://doi.org/10.1126/scitranslmed.abb4416
- Stahl A, Yang YP, 2021, Regenerative approaches for the treatment of large bone defects. Tissue Eng Part B Rev, 27(6): 539–547. https://doi.org/10.1089/ten.TEB.2020.0281
- Dimitriou R, Jones E, Mcgonagle D, et al., 2011, Bone regeneration: Current concepts and future directions. BMC Med, 9: 66. https://doi.org/10.1186/1741-7015-9-66.
- Azi ML, Aprato A, Santi I, et al., 2016, Autologous bone graft in the treatment of post-traumatic bone defects: A systematic review and meta-analysis. BMC Musculoskelet Disord, 17(1): 465. https://doi.org/10.1186/s12891-016-1312-4
- Charbe NB, Tambuwala M, Palakurthi SS, et al., 2023, Biomedical applications of three-dimensional bioprinted craniofacial tissue engineering. Bioeng Transl Med, 8(1): e10333. https://doi.org/10.1002/btm2.10333
- Shi Y, Yu L, Gong C, et al., 2021, A bioactive magnesium phosphate cement incorporating chondroitin sulfate for bone regeneration. Biomed Mater, 16(3). https://doi.org/10.1088/1748-605X/abf5c4
- Roseti L, Parisi V, Petretta M, et al., 2017 Scaffolds for bone tissue engineering: State of the art and new perspectives. Mater Sci Eng C Mater Biol Appl, 78: 1246–1262. https://doi.org/10.1016/j.msec.2017.05.017
- Wunner FM, Wille ML, Noonan TG, et al., 2018, Melt electrospinning writing of highly ordered large volume scaffold architectures. Adv Mater, 30(20): e1706570. https://doi.org/10.1002/adma.201706570
- Kade JC, Dalton PD, 2021, Polymers for melt electrowriting. Adv Healthc Mater, 10(1): e2001232. https://doi.org/10.1002/adhm.202001232
- Chen Z, Liu Y, Huang J, et al., 2022, Influences of process parameters of near-field direct-writing melt electrospinning on performances of polycaprolactone/nano-hydroxyapatite scaffolds. Polymers (Basel), 14(16): 3404. https://doi.org/10.3390/polym14163404
- He J, Zhang B, Li Z, et al., 2020, High-resolution electrohydrodynamic bioprinting: A new biofabrication strategy for biomimetic micro/nanoscale architectures and living tissue constructs. Biofabrication, 12(4): 042002. https://doi.org/10.1088/1758-5090/aba1fa
- Mirzaei M, Dodi G, Gardikiotis I, et al., 2023, 3D high-precision melt electro written polycaprolactone modified with yeast derived peptides for wound healing. Biomater Adv, 149: 213361. https://doi.org/10.1016/j.bioadv.2023.213361
- Daghrery A, Ferreira JA, De Souza Araújo IJ, et al., 2021, A highly ordered, nanostructured fluorinated CaP-coated melt electrowritten scaffold for periodontal tissue regeneration. Adv Healthc Mater, 10(21): e2101152. https://doi.org/10.1002/adhm.202101152
- Santschi MXT, Huber S, Bujalka J, et al., 2022, Mechanical and biological evaluation of melt-electrowritten polycaprolactone scaffolds for acetabular labrum restoration. Cells, 11(21): 3450. https://doi.org/10.3390/cells11213450
- Eichholz KF, Freeman FE, Pitacco P, et al., 2022, Scaffold microarchitecture regulates angiogenesis and the regeneration of large bone defects. Biofabrication, 14(4). https://doi.org/10.1088/1758-5090/ac88a1
- Steele JaM, Moore AC, St-Pierre JP, et al., 2022, In vitro and in vivo investigation of a zonal microstructured scaffold for osteochondral defect repair. Biomaterials, 286: 121548. https://doi.org/10.1016/j.biomaterials.2022.121548
- Abbasi N, Lee RSB, Ivanovski S, et al., 2020, In vivo bone regeneration assessment of offset and gradient melt electrowritten (MEW) PCL scaffolds. Biomater Res, 24: 17. https://doi.org/10.1186/s40824-020-00196-1
- Daghrery A, De Souza Araújo IJ, Castilho M, et al., 2023, Unveiling the potential of melt electrowriting in regenerative dental medicine. Acta Biomater, 156: 88–109. https://doi.org/10.1016/j.actbio.2022.01.010
- Jing L, Wang X, Leng B, et al., 2021, Engineered nanotopography on the microfibers of 3D-printed PCL scaffolds to modulate cellular responses and establish an in vitro tumor model. ACS Appl Bio Mater, 4(2): 1381–1394. https://doi.org/10.1021/acsabm.0c01243
- Meng J, Boschetto F, Yagi S, et al., 2022, Enhancing the bioactivity of melt electrowritten PLLA scaffold by convenient, green, and effective hydrophilic surface modification. Mater Sci Eng C Mater Biol Appl, 135: 112686. https://doi.org/10.1016/j.msec.2022.112686
- Rasperini G, Pilipchuk SP, Flanagan CL, et al., 2015, 3D-printed bioresorbable scaffold for periodontal repair. J Dent Res, 94: 153s–157s. https://doi.org/10.1177/0022034515588303
- Wong HM, Wu S, Chu PK, et al., 2013, Low-modulus Mg/PCL hybrid bone substitute for osteoporotic fracture fixation. Biomaterials, 34(29): 7016–7032. https://doi.org/10.1016/j.biomaterials.2013.05.062
- Feng B, Tu H, Yuan H, et al., 2012, Acetic-acid-mediated miscibility toward electrospinning homogeneous composite nanofibers of GT/PCL. Biomacromolecules, 13(12): 3917–3925. https://doi.org/10.1021/bm3009389
- Zhou Q, Zhang H, Zhou Y, et al., 2017, Alkali-mediated miscibility of gelatin/polycaprolactone for electrospinning homogeneous composite nanofibers for tissue scaffolding. Macromol Biosci, 17(12). https://doi.org/10.1002/mabi.201700268
- Zheng R, Duan H, Xue J, et al., 2014, The influence of Gelatin/PCL ratio and 3-D construct shape of electrospun membranes on cartilage regeneration. Biomaterials, 35(1): 152–164. https://doi.org/10.1016/j.biomaterials.2013.09.082
- Jing X, Mi HY, Wang XC, et al., 2015, Shish-kebab-structured poly(ε-caprolactone) nanofibers hierarchically decorated with chitosan-poly(ε-caprolactone) copolymers for bone tissue engineering. ACS Appl Mater Interfaces, 7(12): 6955– 6965. https://doi.org/10.1021/acsami.5b00900
- Jin S, Yang R, Chu C, et al., 2021, Topological structure of electrospun membrane regulates immune response, angiogenesis and bone regeneration. Acta Biomater, 129: 148–158. https://doi.org/10.1016/j.actbio.2021.05.042
- Hao M, Liu Y, Chen Z, et al., 2022, Cross-linked gamma polyglutamic acid/human hair keratin electrospun nanofibrous scaffolds with excellent biocompatibility and biodegradability. Polymers (Basel), 14(24): 5505. https://doi.org/10.3390/polym14245505
- Crowder SW, Leonardo V, Whittaker T, et al., 2016, Material cues as potent regulators of epigenetics and stem cell function. Cell Stem Cell, 18(1): 39–52. https://doi.org/10.1016/j.stem.2015.12.012
- Lv L, Tang Y, Zhang P, et al., 2018, Biomaterial cues regulate epigenetic state and cell functions-A systematic review. Tissue Eng Part B Rev, 24(2): 112–132. https://doi.org/10.1089/ten.teb.2017.0287
- Veiseh O, Doloff JC, Ma M, et al., 2015, Size- and shape-dependent foreign body immune response to materials implanted in rodents and non-human primates. Nat Mater, 14(6): 643–651. https://doi.org/10.1038/nmat4290
- Leal-Egaña A, Díaz-Cuenca A, Boccaccini AR, 2013, Tuning of cell-biomaterial anchorage for tissue regeneration. Adv Mater, 25(29): 4049–4057. https://doi.org/10.1002/adma.201301227
- Yeo A, Wong WJ, Teoh SH, 2010, Surface modification of PCL-TCP scaffolds in rabbit calvaria defects: Evaluation of scaffold degradation profile, biomechanical properties and bone healing patterns. J Biomed Mater Res A, 93(4): 1358–1367. https://doi.org/10.1002/jbm.a.32633
- Tiaw KS, Goh SW, Hong M, et al., 2005, Laser surface modification of poly(epsilon-caprolactone) (PCL) membrane for tissue engineering applications. Biomaterials, 26(7): 763–769. https://doi.org/10.1016/j.biomaterials.2004.03.010
- Chen Z, Liu Y, Huang J, et al., 2022, Enhanced in vitro biocompatible polycaprolactone/nano-hydroxyapatite scaffolds with near-field direct-writing melt electrospinning technology. J Funct Biomater, 13(4): 161. https://doi.org/10.3390/jfb13040161
- Chen Z, Hao M, Qian X, et al., 2021, Characterization on modification and biocompatibility of PCL scaffold prepared with near-field direct-writing melt electrospinning. Chem Res Chin Univ, 37(3): 578–583. https://doi.org/10.1007/s40242-021-1129-z
- Vaquette C, Ivanovski S, Hamlet SM, et al., 2013, Effect of culture conditions and calcium phosphate coating on ectopic bone formation. Biomaterials, 34(22): 5538–5551. https://doi.org/10.1016/j.biomaterials.2013.03.088
- Gupta D, Singh AK, Kar N, et al., 2019, Modelling and optimization of NaOH-etched 3-D printed PCL for enhanced cellular attachment and growth with minimal loss of mechanical strength. Mater Sci Eng C Mater Biol Appl, 98: 602–611. https://doi.org/10.1016/j.msec.2018.12.084
- Zamani Y, Mohammadi J, Amoabediny G, et al., 2018, Enhanced osteogenic activity by MC3T3-E1 pre-osteoblasts on chemically surface-modified poly(ε-caprolactone) 3D-printed scaffolds compared to RGD immobilized scaffolds. Biomed Mater, 14(1): 015008. https://doi.org/10.1088/1748-605X/aaeb82
- Zhou ZX, Chen YR, Zhang JY, et al., 2020, Facile strategy on hydrophilic modification of poly(ε-caprolactone) scaffolds for assisting tissue-engineered meniscus constructs in vitro. Front Pharmacol, 11: 471. https://doi.org/10.3389/fphar.2020.00471
- Lam CX, Hutmacher DW, Schantz JT, et al., 2009, Evaluation of polycaprolactone scaffold degradation for 6 months in vitro and in vivo. J Biomed Mater Res A, 90(3): 906–919. https://doi.org/10.1002/jbm.a.32052
- Yao C, Qiu Z, Li X, et al., 2023, Electrohydrodynamic printing of microfibrous architectures with cell-scale spacing for improved cellular migration and neurite outgrowth. Small, 19(19): e2207331. https://doi.org/10.1002/smll.202207331
- Eichholz KF, Hoey DA, 2018, Mediating human stem cell behaviour via defined fibrous architectures by melt electrospinning writing. Acta Biomater, 75: 140–151. https://doi.org/10.1016/j.actbio.2018.05.048
- Karageorgiou V, Kaplan D, 2005, Porosity of 3D biomaterial scaffolds and osteogenesis. Biomaterials, 26(27): 5474–5491. https://doi.org/10.1016/j.biomaterials.2005.02.002
- Tian Y, Zheng H, Zheng G, et al., 2022, Hierarchical microgroove/nanopore topography regulated cell adhesion to enhance osseointegration around intraosseous implants in vivo. Biomater Sci, 10(2): 560–580. https://doi.org/10.1039/d1bm01657a
- Zhang P, Chen J, Sun Y, et al., 2023, A 3D multifunctional bi-layer scaffold to regulate stem cell behaviors and promote osteochondral regeneration. J Mater Chem B, 11(6): 1240– 1261. https://doi.org/10.1039/d2tb02203f
- Shegarfi H, Reikeras O, 2009, Review article: Bone transplantation and immune response. J Orthop Surg (Hong Kong), 17(2): 206–211. https://doi.org/10.1177/230949900901700218
- Kanchanawong P, Shtengel G, Pasapera AM, et al., 2010, Nanoscale architecture of integrin-based cell adhesions. Nature, 468(7323): 580–594. https://doi.org/10.1038/nature09621
- Prasopthum A, Cooper M, Shakesheff KM, et al., 2019, Three-dimensional printed scaffolds with controlled micro-/ nanoporous surface topography direct chondrogenic and osteogenic differentiation of mesenchymal stem cells. ACS Appl Mater Interfaces, 11(21): 18896–18906. https://doi.org/10.1021/acsami.9b01472
- Kumar G, Waters MS, Farooque TM, et al., 2012, Freeform fabricated scaffolds with roughened struts that enhance both stem cell proliferation and differentiation by controlling cell shape. Biomaterials, 33(16): 4022–4030. https://doi.org/10.1016/j.biomaterials.2012.02.048
- Neves SC, Mota C, Longoni A, et al., 2016, Additive manufactured polymeric 3D scaffolds with tailored surface topography influence mesenchymal stromal cells activity. Biofabrication, 8(2): 025012. https://doi.org/10.1088/1758-5090/8/2/025012