Development of a low-cost quad-extrusion 3D bioprinting system for multi-material tissue constructs
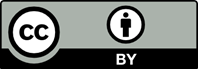
This study presents the development and characterization of a low-cost bioprinting system with a compact low-profile quad-extrusion bioprinting head for producing multi-material tissue constructs. The system, created by modifying an off-the-shelf three-dimensional (3D) printer, enables larger print volumes compared to extant systems. Incorporating gelatin methacrylate (GelMA) as a bioink model, the bioprinting system was systematically tested with two different printing techniques, namely the traditional in-air printing (IAP) mode along with an emerging support bath printing (SBP) paradigm. Structural fidelity was assessed by comparing printed structures under different conditions to the computer-aided design (CAD) model. To evaluate biological functionality, a placental model was created using HTR-8 trophoblasts known for their invasive phenotype. Biological assays of cell viability and invasion revealed that the cells achieved high cell proliferation rates and had over 93% cell viability for a 3-day incubation period. The multi-compartmental 3D-bioprinted in vitro placenta model demonstrates the potential for studying native cell phenotypes and specialized functional outcomes enabled by the multi-material capability of the quad-extrusion bioprinter (QEB). This work represents a significant advancement in bioprinting technology, allowing for the printing of complex and highly organized tissue structures at scale. Moreover, the system’s total build cost is only US$ 297, making it an affordable resource for researchers.
- Correia Carreira S, Begum R, Perriman AW. 3D bioprinting: The emergence of programmable biodesign. Adv Healthc Mater. 2020;9(15):1–14. doi: 10.1002/adhm.201900554
- Zhang Z, Wu C, Dai C, et al. A multi-axis robot-based bioprinting system supporting natural cell function preservation and cardiac tissue fabrication. Bioact Mater. 2022;18(February):138–150. doi: 10.1016/j.bioactmat.2022.02.009
- Ramiah P, du Toit LC, Choonara YE, Kondiah PPD, Pillay V. Hydrogel-based bioinks for 3D bioprinting in tissue regeneration. Front Mater. 2020;7(April):1–13. doi: 10.3389/fmats.2020.00076
- Mandrycky C, Wang Z, Kim K, Kim D-H. 3D bioprinting for engineering complex tissues. Biotechnol Adv. 2016; 34(4): 422–434. doi: 10.1016/j.biotechadv.2015.12.011
- Zaeri A, Cao K, Zhang F, Chang RC. A review of the structural and physical properties that govern cell interactions with structured biomaterials enabled by additive manufacturing. Bioprinting. 2022; 26(January):e00201. doi: 10.1016/j.bprint.2022.e00201
- Moroni L, Boland T, Burdick JA, et al. Biofabrication: A guide to technology and terminology. Trends Biotechnol. 2018;36(4):384–402. doi: 10.1016/j.tibtech.2017.10.015
- Duan J, Cao Y, Shen Z, et al. 3D bioprinted GelMA/PEGDA hybrid scaffold for establishing in-vitro model of melanoma. J Microbiol Biotechnol. 2022;32(3):531–540. doi: 10.4014/jmb.2111.11003
- Rostam-Alilou AA, Jafari H, Zolfagharian A, Serjouei A, Bodaghi M. Experimentally validated vibro-acoustic modeling of 3D bio-printed grafts for potential use in human tympanic membrane regeneration. Bioprinting. 2022;25(January):e00186. doi: 10.1016/j.bprint.2021.e00186
- Naghieh S, Lindberg G, Tamaddon M, Liu C. Biofabrication strategies for musculoskeletal disorders: Evolution towards clinical applications. Bioengineering. 2021;8(9). doi: 10.3390/bioengineering8090123
- Yu HW, Kim BS, Lee JY, et al. Tissue printing for engineering transplantable human parathyroid patch to improve parathyroid engraftment, integration, and hormone secretion in vivo. Biofabrication. 2021;13(3). doi: 10.1088/1758-5090/abf740
- Hwang DG, Jo Y, Kim M, et al. A 3D bioprinted hybrid encapsulation system for delivery of human pluripotent stem cell-derived pancreatic islet-like aggregates. Biofabrication. 2022;14(1). doi: 10.1088/1758-5090/ac23ac
- Celikkin N, Presutti D, Maiullari F, et al. Tackling current biomedical challenges with frontier biofabrication and organ-on-a-chip technologies. Front Bioeng Biotechnol. 2021;9(September):1–26. doi: 10.3389/fbioe.2021.732130
- Zaeri A, Zgeib R, Cao K, Zhang F, Chang RC. Numerical analysis on the effects of microfluidic-based bioprinting parameters on the microfiber geometrical outcomes. Sci Rep. 2022;12(1):1–16. doi: 10.1038/s41598-022-07392-0
- Murphy CA, Lim KS, Woodfield TBF. Next evolution in organ-scale biofabrication: Bioresin design for rapid high-resolution vat polymerization. Adv Mater. 2022;34 (February). doi: 10.1002/adma.202107759
- Kang YJ. Microfluidic-based biosensor for blood viscosity and erythrocyte sedimentation rate using disposable fluid delivery system. Micromachines. 2020;11(2):1–25. doi: 10.3390/mi11020215
- Kang HW, Lee SJ, Ko IK, Kengla C, Yoo JJ, Atala A. A 3D bioprinting system to produce human-scale tissue constructs with structural integrity. Nat Biotechnol. 2016;34(3):312–319. doi: 10.1038/nbt.3413
- Mironov V, Kasyanov V, Markwald RR. Organ printing: From bioprinter to organ biofabrication line. Curr Opin Biotechnol. 2011;22(5):667–673. doi: 10.1016/j.copbio.2011.02.006
- Kang DH, Louis F, Liu H, et al. Engineered whole cut meat-like tissue by the assembly of cell fibers using tendon-gel integrated bioprinting. Nat Commun. 2021;12(1). doi: 10.1038/s41467-021-25236-9
- Ramachandraiah K. Potential development of sustainable 3d-printed meat analogues: A review. Sustain. 2021; 13(2):1–20. doi: 10.3390/su13020938
- Warburton L, Lou L, Rubinsky B. A modular three-dimensional bioprinter for printing porous scaffolds for tissue engineering. J Heat Transfer. 2022;144(3):1–7. doi: 10.1115/1.4053198
- Tavafoghi M, Darabi MA, Mahmoodi M, et al. Multimaterial bioprinting and combination of processing techniques towards the fabrication of biomimetic tissues and organs. Biofabrication. 2021;13(4). doi: 10.1088/1758-5090/ac0b9a
- Tong A, Pham QL, Abatemarco P, et al. Review of low-cost 3D bioprinters: State of the market and observed future trends. SLAS Technol. 2021;26(4):333–366. doi: 10.1177/24726303211020297
- Choudhury D, Anand S, Naing MW. The arrival of commercial bioprinters - Towards 3D bioprinting revolution! Int J Bioprinting. 2018;4(2):1–20. doi: 10.18063/IJB.v4i2.139
- Ravanbakhsh H, Karamzadeh V, Bao G, Luc Mongeau, Juncker D, Zhang YS. Emerging technologies in multi-material bioprinting. Adv Mater. 2021;33(49). doi: 10.1002/adma.202104730.
- Zhang YS, Haghiashtiani G, Hübscher T, Kelly DJ, Malda J. 3D extrusion bioprinting. Nat Rev Methods Prim. 2021;1(1):75. doi: 10.1038/s43586-021-00073-8
- Shen EM, McCloskey KE. Affordable, high-resolution bioprinting with embedded concentration gradients. Bioprinting. 2021;21(October 2020):e00113. doi: 10.1016/j.bprint.2020.e00113
- Kahl M, Gertig M, Hoyer P, Friedrich O, Gilbert DF. Ultra-low-cost 3D bioprinting: Modification and application of an off-the-shelf desktop 3D-printer for biofabrication. Front Bioeng Biotechnol. 2019;7(JUL):1–12. doi: 10.3389/fbioe.2019.00184
- Wagner M, Karner A, Gattringer P, Buchegger B, Hochreiner A. A super low-cost bioprinter based on DVD-drive components and a raspberry pi as controller. Bioprinting. 2021;23(November 2020):e00142. doi: 10.1016/j.bprint.2021.e00142
- Pusch K, Hinton TJ, Feinberg AW. Large volume syringe pump extruder for desktop 3D printers. HardwareX. 2018;3:49–61. doi: 10.1016/j.ohx.2018.02.001
- Krige A, Haluška J, Rova U. Design and implementation of a low cost bio-printer modification, allowing for switching between plastic and gel extrusion. HardwareX. 2021;9:e00186. doi: 10.1016/j.ohx.2021.e00186
- Yenilmez B, Temirel M, Knowlton S, Lepowsky E. Development and characterization of a low-cost 3D bioprinter. Bioprinting. 2019;13(December 2018):e00044. doi: 10.1016/j.bprint.2019.e00044
- Bessler N, Ogiermann D, Buchholz MB, Zhang F, Cao K, Chang RC. Nydus one syringe extruder (NOSE): A Prusa i3 3D printer conversion for bioprinting applications utilizing the FRESH-method. HardwareX. 2019;6:e00069. doi: 10.1016/j.ohx.2019.e00069
- Tashman JW, Shiwarski DJ, Feinberg AW. A high performance open-source syringe extruder optimized for extrusion and retraction during FRESH 3D bioprinting. HardwareX. 2021;9(2021):e00170. doi: 10.1016/j.ohx.2020.e00170
- Garciamendez-Mijares CE, Agrawal P, García Martínez G, Juárez EC. State-of-art affordable bioprinters: A guide for the DiY community. Appl Phys Rev. 2021;8(3):47818. doi: 10.1063/5.0047818
- Ding H, Illsley NP, Chang RC. 3D bioprinted GelMA based models for the study of trophoblast cell invasion. Sci Rep. 2019;9(1):1–14. doi: 10.1038/s41598-019-55052-7
- Salahuddin B, Wang S, Sangian D, Aziz S. Hybrid gelatin hydrogels in nanomedicine applications. ACS Appl Bio Mater. 2021;4(4):2886–2906. doi: 10.1021/acsabm.0c01630
- Li H, Tan YJ, Kiran R, Shu Beng T, Kun Z. Submerged and non-submerged 3D bioprinting approaches for the fabrication of complex structures with the hydrogel pair GelMA and alginate/methylcellulose. Addit Manuf. 2021;37(October 2020):101640. doi: 10.1016/j.addma.2020.101640
- S. Alsoufi M, W. Alhazmi M, K. Suker D, et al. Experimental characterization of the influence of nozzle temperature in FDM 3D printed pure PLA and advanced PLA+. Am J Mech Eng. 2019;7(2):45–60. doi: 10.12691/ajme-7-2-1
- Zhu W, Qu X, Zhu J, et al. Direct 3D bioprinting of prevascularized tissue constructs with complex microarchitecture. Biomaterials. 2017;124(April 2017):106–115. doi: 10.1016/j.biomaterials.2017.01.042.Direct
- Ding H, Chang RC. Printability study of bioprinted tubular structures using liquid hydrogel precursors in a support bath. Appl Sci. 2018;8(3). doi: 10.3390/app8030403
- Gu Y, Schwarz B, Forget A, Barbero A, Martin I, Prasad Shastri V. Advanced bioink for 3D bioprinting of complex free-standing structures with high stiffness. Bioengineering. 2020;7(4):1–15. doi: 10.3390/bioengineering7040141
- Schwab A, Levato R, D’Este M, Piluso S, Eglin D, Malda J. Printability and shape fidelity of bioinks in 3D bioprinting. Chem Rev. 2020;120(19):11028–11055. doi: 10.1021/acs.chemrev.0c00084
- Bonatti AF, Chiesa I, Vozzi G, De Maria C. Open-source CAD-CAM simulator of the extrusion-based bioprinting process. Bioprinting. 2021;24(July):e00172. doi: 10.1016/j.bprint.2021.e00172
- Paxton N, Smolan W, Böck T, Melchels F, Groll J, Jungst T. Proposal to assess printability of bioinks for extrusion-based bioprinting and evaluation of rheological properties governing bioprintability. Biofabrication. 2017;9(4). doi: 10.1088/1758-5090/aa8dd8
- Hölzl K, Lin S, Tytgat L, Van Vlierberghe S, Gu L, Ovsianikov A. Bioink properties before, during and after 3D bioprinting. Biofabrication. 2016;8(3). doi: 10.1088/1758-5090/8/3/032002
- Suntornnond R, An J, Chua CK. Bioprinting of thermoresponsive hydrogels for next generation tissue engineering: A review. Macromol Mater Eng. 2017;302(1). doi: 10.1002/mame.201600266
- Li H, Zheng H, Tan YJ, Tor SB, Zhou K. Development of an ultrastretchable double-network hydrogel for flexible strain sensors. ACS Appl Mater Interfaces. 2021;13(11): 12814–12823. doi: 10.1021/acsami.0c19104
- Boularaoui S, Al Hussein G, Khan KA, Christoforou N. An overview of extrusion-based bioprinting with a focus on induced shear stress and its effect on cell viability. Bioprinting. 2020;20(August):e00093. doi: 10.1016/j.bprint.2020.e00093
- Liu W, Heinrich MA, Zhou Y, et al. Extrusion bioprinting of shear-thinning gelatin methacryloyl bioinks. Adv Healthc Mater. 2017;6(12):1–11. doi: 10.1002/adhm.201601451
- Emmermacher J, Spura D, Cziommer J, et al. Engineering considerations on extrusion-based bioprinting: interactions of material behavior, mechanical forces and cells in the printing needle. Biofabrication. 2020;12(2). doi: 10.1088/1758-5090/ab7553
- Han S, Kim CM, Jin S, Kim TY. Study of the process-induced cell damage in forced extrusion bioprinting. Biofabrication. 2021;13(3). doi: 10.1088/1758-5090/ac0415
- Poologasundarampillai G, Haweet A, Jayash SN, Morgan G, Moore JE. , Alessia C. Real-time imaging and analysis of cell-hydrogel interplay within an extrusion-bioprinting capillary. Bioprinting. 2021;23(May):e00144. doi: 10.1016/j.bprint.2021.e00144
- Boularaoui S, Shanti A, Lanotte M, et al. Nanocomposite conductive bioinks based on low-concentration GelMA and MXene nanosheets/gold nanoparticles providing enhanced printability of functional skeletal muscle tissues. ACS Biomater Sci Eng. 2021;7(12):5810–5822. doi: 10.1021/acsbiomaterials.1c01193
- Yin J, Yan M, Wang Y, Fu J, Suo H. 3D bioprinting of low-concentration cell-laden gelatin methacrylate (GelMA) bioinks with a two-step cross-linking strategy. ACS Appl Mater Interfaces. 2018;10(8):6849–6857. doi: 10.1021/acsami.7b16059
- Xu W, Molino BZ, Cheng F, et al. On low-concentration inks formulated by nanocellulose assisted with gelatin methacrylate (GelMA) for 3D printing toward wound healing application. ACS Appl Mater Interfaces. 2019;11(9):8838–8848. doi: 10.1021/acsami.8b21268
- Ouyang L, Yao R, Zhao Y, Sun W. Effect of bioink properties on printability and cell viability for 3D bioplotting of embryonic stem cells. Biofabrication. 2016;8(3):035020. doi: 10.1088/1758-5090/8/3/035020
- Abou-Kheir W, Barrak J, Hadadeh O, Daoud G. HTR- 8/SVneo cell line contains a mixed population of cells. Placenta. 2017;50:1–7. doi: 10.1016/j.placenta.2016.12.007
- Msheik H, Azar J, El Sabeh M, Abou-Kheir W, Daoud G. HTR- 8/SVneo: A model for epithelial to mesenchymal transition in the human placenta. Placenta. 2020;90(September 2019):90–97. doi: 10.1016/j.placenta.2019.12.013
- 59. Kuo CY, Eranki A, Placone JK, et al. Development of a 3D printed, bioengineered placenta model to evaluate the role of trophoblast migration in preeclampsia. ACS Biomater Sci Eng. 2016;2(10):1817–1826. doi: 10.1021/acsbiomaterials.6b00031