3D bioprinting and scaffold-free strategies for fabrication of multi-cellular tissues or organoids
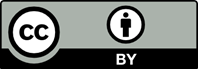
The biofabrication of multi-cellular tissues or organoids (MTOs) has been challenging in regenerative medicine for decades. Currently, two primary technological approaches are being explored: scaffold-based strategies utilizing three-dimensional (3D) bioprinting techniques and scaffold-free strategies employing bioassembly techniques. 3D bioprinting techniques include jetting-based, extrusion-based, and vat photopolymerization-based methods, and bioassembly techniques include Kenzan, fluid-based manipulation and microfluid, bioprinting-assisted tissue emergence, and aspiration-assisted technology methods. Scaffold-based strategies primarily concentrate on the construction of scaffold structures to provide an extracellular environment, while scaffold-free strategies primarily emphasize the assembly methods of building blocks. Different biofabrication technologies have their advantages and limitations. This review provides an overview of the mechanisms, advantages, and limitations of scaffold-based and scaffold-free strategies in tissue engineering. It also compares the strengths and weaknesses of these two strategies, along with their respective suitability under different conditions. Moreover, the significant challenges in the future development of convergence strategies, specifically the integration of scaffold-based and scaffold-free approaches, are examined in an objective manner. This review concludes that integrating scaffold-based and scaffold-free strategies could overcome the problems in the biofabrication of MTOs. A novel fabrication method, the BioMicroMesh method, is proposed based on the convergence strategy. Concurrently, the development of a desktop-scale integrated intelligent biofabrication device, the BioMicroMesh system, is underway. This system is tailored to the BioMicroMesh method and incorporates cell aggregate spheroids preparation, 3D bioprinting, bioassembly, and multi-organoid co-culture functions, providing an objective perspective on its capabilities.
- Prior N, Inacio P, Huch M, 2019, Liver organoids: From basic research to therapeutic applications. Gut, 68(12): 2228–2237. https://doi.org/10.1136/gutjnl-2019-319256
- Lancaster MA, Knoblich JA, 2014, Organogenesis in a dish: Modeling development and disease using organoid technologies. Science, 345(6194): 1247125. https://doi.org/10.1126/science.1247125
- Clevers H, 2016, Modeling development and disease with organoids. Cell, 165(7): 1586–1597. https://doi.org/10.1016/j.cell.2016.05.082
- Meran L, Tullie L, Eaton S, et al., 2022, Bioengineering human intestinal mucosal grafts using patient-derived organoids, fibroblasts and scaffolds. Nat Protoc, 18(1): 108–135. https://doi.org/10.1038/s41596-022-00751-1
- Carosio S, Barberi L, Rizzuto E, et al., 2013, Generation of ex vivo-vascularized muscle engineered tissue (X-MET). Sci Rep, 3(1): 1–9. https://doi.org/10.1038/srep01420
- Kratochvil MJ, Seymour AJ, Li TL, et al., 2019, Engineered materials for organoid systems. Nat Rev Mater, 4(9): 606–622. https://doi.org/10.1038/s41578-019-0129-9
- Hofer M, Lutolf MP, 2021, Engineering organoids. Nat Rev Mater, 6(5): 402–420. https://doi.org/10.1038/s41578-021-00279-y
- Groll J, Boland T, Blunk T, et al., 2016, Biofabrication: Reappraising the definition of an evolving field. Biofabrication, 8(1): 013001. https://doi.org/10.1088/1758-5090/8/1/013001
- Heinrich MA, Liu W, Jimenez A, et al., 2019, 3D-Bioprinting: From benches to translational applications. Small, 15(23): 1805510. https://doi.org/10.1002/smll.201970126
- Moroni L, Burdick JA, Highley C, et al., 2018, Biofabrication strategies for 3D in vitro models and regenerative medicine. Nat Rev Mater, 36(4): 21–37.
- Martin I, Malda J, Rivron NC, 2019, Organs by design: Can bioprinting meet self-organization? Curr Opin Organ Transplant, 24(5): 562–567. https://doi.org/10.1097/MOT.0000000000000679
- Kačarević Ž, Rider P, Alkildani S, et al., 2018, An introduction to 3D-bioprinting: Possibilities, challenges and future aspects. Materials, 11(11): 2199. https://doi.org/10.3390/ma11112199
- Ahn CB, Lee J-H, Kim JH, et al., 2022, Development of a 3D subcutaneous construct containing insulin-producing beta cells using bioprinting. Bio-Des Manuf, 5(2): 265–276. https://doi.org/10.1007/s42242-021-00178-9
- Chen EP, Toksoy Z, Davis BA, et al., 2021, 3D-bioprinting of vascularized tissues for in vitro and in vivo applications. Front Bioeng Biotechnol, 9: 664188. https://doi.org/10.3389/fbioe.2021.664188
- Zhang X-Y, Yanagi Y, Sheng Z, et al., 2018, Regeneration of diaphragm with bio-3D cellular patch. Biomaterials, 167: 1–14. https://doi.org/10.1016/j.biomaterials.2018.03.012
- Burdis R, Kelly DJ, 2021, Biofabrication and bioprinting using cellular aggregates, microtissues and organoids for the engineering of musculoskeletal tissues. Acta Biomater, 126: 1–14. https://doi.org/10.1016/j.actbio.2021.03.016
- Lawlor KT, Vanslambrouck JM, Higgins JW, et al., 2021, Cellular extrusion bioprinting improves kidney organoid reproducibility and conformation. Nat Mater, 20(2): 260–271. https://doi.org/10.1038/s41563-020-00853-9
- Ren Y, Yang X, Ma Z, et al., 2021, Developments and opportunities for 3D bioprinted organoids. Int J Bioprint, 7(3): 364. https://doi.org/10.18063/ijb.v7i3.364
- Chan BP, Leong KW, 2008, Scaffolding in tissue engineering: general approaches and tissue-specific considerations. Eur Spine J, 17(S4): 467–479. https://doi.org/10.1007/s00586-008-0745-3
- Ng WL, Huang X, Shkolnikov V, et al., 2021, Controlling droplet impact velocity and droplet volume: Key factors to achieving high cell viability in sub-nanoliter droplet-based bioprinting. Int J Bioprint, 8(1): 424. https://doi.org/10.18063/ijb.v8i1.424
- Willerth SM, Sakiyama-Elbert SE, 2019, Combining stem cells and biomaterial scaffolds for constructing tissues and cell delivery. StemJournal, 1(1): 1–25. https://doi.org/10.3233/STJ-180001
- Ji S, Guvendiren M, 2019, 3D printed wavy scaffolds enhance mesenchymal stem cell osteogenesis. Micromachines (Basel), 11(1): 31. https://doi.org/10.3390/mi11010031
- Di Marzio N, Eglin D, Serra T, et al., 2020, Bio-fabrication: Convergence of 3D-Bioprinting and nano-biomaterials in tissue engineering and regenerative medicine. Front Bioeng Biotechnol, 8: 326. https://doi.org/10.3389/fbioe.2020.00326
- Mei Q, Rao J, Bei HP, et al., 2021, 3D-Bioprinting photo-crosslinkable hydrogels for bone and cartilage repair. Int J Bioprint, 7(3): 367. https://doi.org/10.18063/ijb.v7i3.367
- Ozbolat IT, Hospodiuk M, 2016, Current advances and future perspectives in extrusion-based bioprinting. Biomaterials, 76: 321–343. https://doi.org/10.1016/j.biomaterials.2015.10.076
- Ng WL, Lee JM, Zhou M, et al., 2020, Vat polymerization-based bioprinting—process, materials, applications and regulatory challenges. Biofabrication, 12(2): 022001. https://doi.org/10.1088/1758-5090/ab6034
- Seol Y-J, Kang H-W, Lee SJ, et al., 2014, Bioprinting technology and its applications. Eur J Cardiothorac Surg, 46(3): 342–348. https://doi.org/10.1093/ejcts/ezu148
- Cui X, Boland T, D’Lima DD, et al., 2012, Thermal inkjet printing in tissue engineering and regenerative medicine. Recent Pat Drug Deliv Formul, 6(2): 149–155. https://doi.org/10.2174/187221112800672949
- Nakamura M, Iwanaga S, Henmi C, et al., 2010, Biomatrices and biomaterials for future developments of bioprinting and biofabrication. Biofabrication, 2(1): 014110. https://doi.org/10.1088/1758-5082/2/1/014110
- Wijshoff H, 2010, The dynamics of the piezo inkjet printhead operation. Phys Rep, 491(4–5): 77–177. https://doi.org/10.1016/j.physrep.2010.03.003
- Christensen K, Xu C, Chai W, et al., 2015, Freeform inkjet printing of cellular structures with bifurcations. Biotechnol Bioeng, 112(5): 1047–1055. https://doi.org/10.1002/bit.25501
- Park J-U, Hardy M, Kang S, et al., 2007, High-resolution electrohydrodynamic jet printing. Nat Mater, 6(10): 782–789. https://doi.org/10.1038/nmat1974
- Poellmann MJ, Barton KL, Mishra S, et al., 2011, Patterned hydrogel substrates for cell culture with electrohydrodynamic jet printing. Macromol Biosci, 11(9): 1164–1168. https://doi.org/10.1002/mabi.201100004
- Li X, Liu B, Pei B, et al., 2020, Inkjet bioprinting of biomaterials. Chem Rev, 120(19): 10793–10833. https://doi.org/10.1021/acs.chemrev.0c00008
- Xu T, Zhao W, Zhu J-M, et al., 2007, Bio-printing of living organized tissues using an inkjet technology. FASEB J, 21(5): A636–A636. https://doi.org/10.1096/fasebj.21.5.A636-b
- Zhou Y, Zhao Q, Wang M, 2023, Biomanufacturing of biomimetic three-dimensional nanofibrous multicellular constructs for tissue regeneration. Colloids Surf B, 223: 113189. https://doi.org/10.1016/j.colsurfb.2023.113189
- Jiang T, Munguia-Lopez JG, Flores-Torres S, et al., 2019, Extrusion bioprinting of soft materials: An emerging technique for biological model fabrication. Appl Phys Rev, 6(1): 011310. https://doi.org/10.1063/1.5059393
- Cuvellier M, Ezan F, Oliveira H, et al., 2021, 3D culture of HepaRG cells in GelMa and its application to bioprinting of a multicellular hepatic model. Biomaterials, 269: 120611. https://doi.org/10.1016/j.biomaterials.2020.120611
- Janani G, Priya S, Dey S, et al., 2022, Mimicking native liver lobule microarchitecture in vitro with parenchymal and non-parenchymal cells using 3D-bioprinting for drug toxicity and drug screening applications. ACS Appl Mater Interfaces, 14(8): 10167–10186. https://doi.org/10.1021/acsami.2c00312
- Jian H, Li X, Dong Q, et al., 2023, In vitro construction of liver organoids with biomimetic lobule structure by a multicellular 3D-Bioprinting strategy. Cell Prolif, 56(5): e13465. https://doi.org/10.1111/cpr.13465
- Du L, Qin C, Zhang H, et al., 2023, Multicellular bioprinting of biomimetic inks for Tendon-to-Bone regeneration. Adv Sci, 2301309. http://doi.org/10.1002/advs.202301309
- Zhu W, Ma X, Gou M, et al., 2016, 3D printing of functional biomaterials for tissue engineering. Curr Opin Biotechnol, 40: 103–112. https://doi.org/10.1016/j.copbio.2016.03.014
- Murata D, Arai K, Nakayama K, 2020, Scaffold-free bio- 3D printing using spheroids as “bio-inks” for tissue (re-) construction and drug response tests. Adv Healthc Mater, 9(15): e1901831. https://doi.org/10.1002/adhm.201901831
- Tavares-Negrete JA, Babayigit C, Najafikoshnoo S, et al., n.d., A novel 3D-bioprinting technology of orderly extruded multi-materials via photopolymerization. Adv Mater Technol, 8(12): 2201926. https://doi.org/10.1002/admt.202201926
- Kiratitanaporn W, Guan J, Berry DB, et al., 2023, Multi-modal vat photopolymerization for microscale modulation of scaffold stiffness assisted via machine learning. SSRN https://doi.org/10.2139/ssrn.4450557
- Vijayavenkataraman S, Yan W-C, Lu WF, et al., 2018, 3D-Bioprinting of tissues and organs for regenerative medicine. Adv Drug Deliv Rev, 132: 296–332. https://doi.org/10.1016/j.addr.2018.07.004
- Burks HE, Phamduy TB, Azimi MS, et al., 2016, Laser direct-write onto live tissues: A novel model for studying cancer cell migration. J Cel Physiol, 231(11): 2333–2338. https://doi.org/10.1002/jcp.25363
- Jahangirian H, Azizi S, Rafiee-Moghaddam R, et al., 2019, Status of plant protein-based green scaffolds for regenerative medicine applications. Biomolecules, 9(10): 619. https://doi.org/10.3390/biom9100619
- Mekhileri NV, Lim KS, Brown GCJ, et al., 2018, Automated 3D bioassembly of micro-tissues for biofabrication of hybrid tissue engineered constructs. Biofabrication, 10(2): 024103. https://doi.org/10.1088/1758-5090/aa9ef1
- Halbleib JM, Nelson WJ, 2006, Cadherins in development: Cell adhesion, sorting, and tissue morphogenesis. Genes Dev, 20(23): 3199–3214. https://doi.org/10.1101/gad.1486806
- Turksen K, ed., 2018, Cell biology and translational medicine, Volume 3: Stem cells, bio-materials and tissue engineering, Springer International Publishing, Cham, 1107. https://doi.org/10.1007/978-3-030-04185-4
- Zhang Q, Ma L, Ji X, et al., 2022, High-strength hydroxyapatite scaffolds with minimal surface macrostructures for load-bearing bone regeneration. Adv Funct Mater, 32(33): 2204182. https://doi.org/10/gqpwqm
- Forgacs G, 2018, In 3D Bioprinting in regenerative engineering: Applications of extrusion bioprinting, CRC Press, Boca Raton: Taylor & Francis, 51–75. https://doi.org/10.1201/b21916-3
- dos Santos BC, Noritomi PY, da Silva JVL, et al., 2022, Biological multiscale computational modeling: A promising tool for 3D-Bioprinting and tissue engineering. Bioprinting, 28: e00234. https://doi.org/10.1016/j.bprint.2022.e00234
- Sung K, Patel NR, Ashammakhi N, et al., 2021, 3-Dimensional bioprinting of cardiovascular tissues. JACC, 6(5): 467–482. https://doi.org/10.1016/j.jacbts.2020.12.006
- Wang Y, Wang J, Ji Z, et al., 2022, Application of bioprinting in ophthalmology. Int J Bioprint, 8(2): 552. https://doi.org/10.18063/ijb.v8i2.552
- Murata D, Fujimoto R, Nakayama K, 2020, Osteochondral regeneration using adipose tissue-derived mesenchymal stem cells. IJMS, 21(10): 3589. https://doi.org/10.3390/ijms21103589
- Aguilar IN, Smith LJ, Olivos DJ, et al., 2019, Scaffold-free bioprinting of mesenchymal stem cells with the regenova printer: Optimization of printing parameters. Bioprinting, 15: e00048. https://doi.org/10.1016/j.bprint.2019.e00048
- Ayan B, Heo DN, Zhang Z, et al., 2020, Aspiration-assisted bioprinting for precise positioning of biologics. Sci Adv, 10(1): 13148. https://doi.org/10.1126/sciadv.aaw5111
- Moldovan NI, Hibino N, Nakayama K, 2017, Principles of the Kenzan method for robotic cell spheroid-based three-dimensional bioprinting, Tissue Eng Part B Rev, 23(3): 237. https://doi.org/10.1089/ten.teb.2016.0322
- Smith LJ, Li P, Holland MR, et al., 2018, FABRICA: A bioreactor platform for printing, perfusing, observing, & stimulating 3D tissues. Sci Rep, 8(1): 7561. https://doi.org/10.1038/s41598-018-25663-7
- Holland I, Logan J, Shi J, et al., 2018, 3D biofabrication for tubular tissue engineering. Bio-Design Manuf, 1(2): 89–100. https://doi.org/10.1007/s42242-018-0013-2
- Manning KL, Thomson AH, Morgan JR, 2018, Funnel-guided positioning of multicellular microtissues to build macrotissues. Tissue Eng Part C Methods, 24(10): 557–565. https://doi.org/10.1089/ten.tec.2018.0137
- van Pel DM, Harada K, Song D, et al., 2018, Modelling glioma invasion using 3D-Bioprinting and scaffold-free 3D culture. J Cell Commun Signal, 12(4): 723–730. https://doi.org/10.1007/s12079-018-0469-z
- Nakanishi Y, Okada T, Takeuchi N, et al., 2019, Histological evaluation of tendon formation using a scaffold-free three-dimensional-bioprinted construct of human dermal fibroblasts under in vitro static tensile culture. Regen Ther, 11: 47–55. https://doi.org/10.1016/j.reth.2019.02.002
66. Taniguchi D, Matsumoto K, Tsuchiya T, et al., 2018, Scaffold-free trachea regeneration by tissue engineering with bio- 3D printing. Interact CardioVasc Thorac Surg, 26(5): 745–752.
- Yurie H, Ikeguchi R, Aoyama T, et al., 2017, The efficacy of a scaffold-free Bio 3D conduit developed from human fibroblasts on peripheral nerve regeneration in a rat sciatic nerve model. PloS One, 12(2): e0171448. https://doi.org/10.1371/journal.pone.0171448
- Ozbolat IT, 2015, Scaffold-based or scaffold-free bioprinting: Competing or complementing approaches? J Nanotechnol Eng Med, 6(2): 024701. https://doi.org/10.1115/1.4030414
- Guan Y, Niu H, Liu Z, et al., 2021, Sustained oxygenation accelerates diabetic wound healing by promoting epithelialization and angiogenesis and decreasing inflammation. Sci Adv, 7(35): eabj0153. https://doi.org/10.1126/sciadv.abj0153
- Banerjee D, Singh YP, Datta P, et al., 2022, Strategies for 3D bioprinting of spheroids: A comprehensive review. Biomaterials, 291: 121881. http://doi.org/10.1016/j.biomaterials.2022.121881
- Huang TJ, 2019, Acoustofluidics: Merging acoustics and microfluidics for biomedical applications. J Acoust Soc Am, 145(3): 1786–1786. https://doi.org/10.1121/1.5101531
- Devendran C, Carthew J, Frith JE, et al., 2019, Cell adhesion, morphology, and metabolism variation via acoustic exposure within microfluidic cell handling systems. Adv Sci, 6(24): 1902326. https://doi.org/10.1002/advs.201902326
- Karthick S, Pradeep PN, Kanchana P, et al., 2018, Acoustic impedance-based size-independent isolation of circulating tumour cells from blood using acoustophoresis. Lab Chip, 18(24): 3802–3813. https://doi.org/10.1039/C8LC00921J
- Ozcelik A, Rufo J, Guo F, et al., 2018, Acoustic tweezers for the life sciences. Nat Methods, 15(12): 1021–1028. https://doi.org/10.1038/s41592-018-0222-9
75. Jonnalagadda US, Hill M, Messaoudi W, et al., 2018, Acoustically modulated biomechanical stimulation for human cartilage tissue engineering. Lab Chip, 18(3): 473–485. https://doi.org/10.1039/C7LC01195D
- Bouyer C, Chen P, Demirci U, et al., 2016, Bio-acoustic levitational assembly of heterocellular multilayer constructs for tissue engineering. J Acoust Soc Am, 140(4): 3370–3370. https://doi.org/10.1121/1.4970762
- Bouyer C, Chen P, Güven S, et al., 2016, A bio-acoustic levitational (BAL) assembly method for engineering of multilayered, 3D brain-like constructs, using human embryonic stem cell derived neuro-progenitors. Adv Mater, 28(1): 161–167. https://doi.org/10.1002/adma.201503916
- Tian Z, Wang Z, Zhang P, et al., 2020, Generating multifunctional acoustic tweezers in Petri dishes for contactless, precise manipulation of bioparticles. Sci Adv, 6(37): eabb0494. https://doi.org/10.1126/sciadv.abb0494
- Melde K, Mark AG, Qiu T, et al., 2016, Holograms for acoustics. Nature, 537(7621): 518–522. https://doi.org/10.1038/nature19755
- Afsaneh H, Mohammadi R, 2022, Microfluidic platforms for the manipulation of cells and particles. Talanta Open, 5: 100092. https://doi.org/10.1016/j.talo.2022.100092
- Guimarães CF, Soto F, Wang J, et al., 2022, Engineered living bioassemblies for biomedical and functional material applications. Curr Opin Biotechnol, 77: 102756. https://doi.org/10.1016/j.copbio.2022.102756
- Shi L, Zhong X, Ding H, et al., 2022, Continuous separation of microparticles based on optically induced dielectrophoresis. Microfluid Nanofluid, 26(1): 6. https://doi.org/10.1007/s10404-021-02512-0
- Yang C-M, Yu J-C, Chu P-Y, et al., 2022, The utilization of tunable transducer elements formed by the manipulation of magnetic beads with different sizes via optically induced dielectrophoresis (ODEP) for high signal-to-noise ratios (SNRs) and multiplex fluorescence-based biosensing applications. Biosensors, 12(9): 755. https://doi.org/10.3390/bios12090755
- Bhattacharjee T, Gil CJ, Marshall SL, et al., 2016, Liquid-like solids support cells in 3D. ACS Biomater Sci Eng, 2(10): 1787–1795. https://doi.org/10.1021/acsbiomaterials.6b00218
- Noor N, Shapira A, Edri R, et al., 2019, 3D printing of personalized thick and perfusable cardiac patches and hearts. Adv Sci, 6(11): 1900344. https://doi.org/10.1002/advs.201900344
- Jeon O, Lee YB, Jeong H, et al., 2019, Individual cell-only bioink and photocurable supporting medium for 3D printing and generation of engineered tissues with complex geometries. Mater Horiz, 6(8): 1625–1631. https://doi.org/10.1039/C9MH00375D
- Skylar-Scott MA, Uzel SGM, Nam LL, et al., 2019, Biomanufacturing of organ-specific tissues with high cellular density and embedded vascular channels. Sci Adv, 5(9): eaaw2459. https://doi.org/10.1126/sciadv.aaw2459
- Brassard JA, Nikolaev M, Hübscher T, et al., 2021, Recapitulating macro-scale tissue self-organization through organoid bioprinting. Nat Mater, 20(1): 22–29. https://doi.org/10.1038/s41563-020-00803-5
- Ovsianikov A, Khademhosseini A, Mironov V, 2018, The synergy of scaffold-based and scaffold-free tissue engineering strategies. Trends Biotechnol, 36(4): 348–357. https://doi.org/10.1016/j.tibtech.2018.01.005
- Poh PS, Lingner T, Kalkhof S, et al., 2022, Enabling technologies towards personalization of scaffolds for large bone defect regeneration. Curr Opin Biotechnol, 74: 263–270. https://doi.org/10.1016/j.copbio.2021.12.002
- Ponnada S, Babu Gorle D, Chandra Bose RS, et al., 2022, Current insight into 3D printing in solid-state lithium-ion batteries: A perspective. Batter Supercaps, 5(8): e202200223. https://doi.org/10.1002/batt.202200223
- Badhe RV, Chatterjee A, Bijukumar D, et al., 2023, Current advancements in bio-ink technology for cartilage and bone tissue engineering. Bone, 171: 116746. https://doi.org/10.1016/j.bone.2023.116746
- Jafari A, Ajji Z, Mousavi A, et al., 2022, Latest advances in 3D-bioprinting of cardiac tissues. Adv Mater Technol, 7(11): 2101636. https://doi.org/10.1002/admt.202101636
- Caprio ND, Burdick JA, 2022, Engineered biomaterials to guide spheroid formation, function, and fabrication into 3D tissue constructs. Acta Biomater, 165: 4–18.. https://doi.org/10.1016/j.actbio.2022.09.052
- Cooper SM, Rainbow RS, 2022, The developing field of scaffold-free tissue engineering for articular cartilage repair. Tissue Eng Part B Rev, 28(5): 995–1006. https://doi.org/10.1089/ten.teb.2021.0130
- Pignatelli C, Campo F, Neroni A, et al., 2022, Bioengineering the vascularized endocrine pancreas: A fine-tuned interplay between vascularization, extracellular-matrix-based scaffold architecture, and insulin-producing cells. Transpl Int, 35: 10555. https://doi.org/10.3389/ti.2022.10555
- Levato R, Jungst T, Scheuring RG, et al., 2020, From shape to function: The next step in bioprinting. Adv Mater, 32(12): 1906423. https://doi.org/10.1002/adma.201906423
- de Ruijter M, Ribeiro A, Dokter I, et al., 2019, Simultaneous micropatterning of fibrous meshes and bioinks for the fabrication of living tissue constructs. Adv Healthc Mater, 8(7): 1800418.
- Lv S, Nie J, Gao Q, et al., 2020, Micro/nanofabrication of brittle hydrogels using 3D printed soft ultrafine fiber molds for damage-free demolding. Biofabrication, 12(2): 025015. https://doi.org/10.1088/1758-5090/ab57d8
- Chakraborty J, Ghosh S, 2020, Cellular proliferation, self-assembly, and modulation of signaling pathways in silk fibroin gelatin-based 3D bioprinted constructs. ACS Appl Bio Mater, 3(12): 8309–8320. https://doi.org/10.1021/acsabm.0c01252
- Yu Y, Moncal KK, Li J, et al., 2016, Three-dimensional bioprinting using self-assembling scalable scaffold-free “tissue strands” as a new bioink. Sci Rep, 6(1): 28714. https://doi.org/10.1038/srep28714
- Dissanayaka WL, Zhu L, Hargreaves KM, et al., 2014, Scaffold-free prevascularized microtissue spheroids for pulp regeneration. J Dent Res, 93(12): 1296–1303. https://doi.org/10.1177/0022034514550040
- Laurent J, Blin G, Chatelain F, et al., 2017, Convergence of microengineering and cellular self-organization towards functional tissue manufacturing. Nat Biomed Eng, 1(12): 939–956. https://doi.org/10.1038/s41551-017-0166-x
- McMaster R, Hoefner C, Hrynevich A, et al., 2019, Tailored melt electrowritten scaffolds for the generation of sheet‐like tissue constructs from multicellular spheroids. Adv Healthc Mater, 8(7): 1801326. https://doi.org/10.1002/adhm.201801326
- Daly AC, Davidson MD, Burdick JA, 2021, 3D-Bioprinting of high cell-density heterogeneous tissue models through spheroid fusion within self-healing hydrogels. Nat Commun, 12(1): 1–13. https://doi.org/10.1038/s41467-021-21029-2
- Faraji Rad Z, Prewett PD, Davies GJ, 2021, High-resolution two-photon polymerization: the most versatile technique for the fabrication of microneedle arrays. Microsyst Nanoeng, 7(1): 1–17. https://doi.org/10.1038/s41378-021-00298-3
- Santoni S, Gugliandolo SG, Sponchioni M, et al., 2022, 3D-Bioprinting: Current status and trends—a guide to the literature and industrial practice. Bio-Des Manuf, 5(1, SI): 14–42. https://doi.org/10.1007/s42242-021-00165-0
- Struzyna LA, Watt ML, 2021, The emerging role of neuronal organoid models in drug discovery: Potential applications and hurdles to implementation. Mol Pharmacol, 99(4): 256–265. https://doi.org/10.1124/molpharm.120.000142
- Zhang Y, Li W, Pan P, et al., 2022, Programmable construction of vasculature by printing in cementitious materials for self-healing application. Compos Part B Eng, 242: 110056. https://doi.org/10.1016/j.compositesb.2022.110056
- Liu H, Zhou H, Lan H, et al., 2018, Multinozzle multichannel temperature deposition system for construction of a blood vessel. SLAS Technol, 23(1): 64–69. https://doi.org/10.1177/2472630317712221
- Liu H, Zhou H, Lan H, et al., 2017, 3D printing of artificial blood vessel: Study on multi-parameter optimization design for vascular molding effect in alginate and gelatin. Micromachines, 8(8): 237. https://doi.org/10.3390/mi8080237
- Wang S, Chen X, Han X, et al., 2023, A review of 3D printing technology in pharmaceutics: Technology and applications, now and future. Pharmaceutics, 15(2): 416. https://doi.org/10.3390/pharmaceutics15020416
- Murata D, Arai K, Nakayama K, 2020, Scaffold‐free bio‐3D printing using spheroids as “bio‐inks” for tissue (re‐) construction and drug response tests. Adv Healthc Mater, 267(1): 1901831. https://doi.org/10.1002/adhm.201901831
- Yaman S, Anil-Inevi M, Ozcivici E, et al., 2018, Magnetic force-based microfluidic techniques for cellular and tissue bioengineering. Front Bioeng Biotechnol, 6: 192. https://doi.org/10.3389/fbioe.2018.00192
- Hsiao Y-C, Wang C-H, Lee W-B, et al., 2018, Automatic cell fusion via optically-induced dielectrophoresis and optically-induced locally-enhanced electric field on a microfluidic chip. Biomicrofluidics, 12(3): 034108. https://doi.org/10.1063/1.5028158
- Hinton TJ, Jallerat Q, Palchesko RN, et al., 2015, Three-dimensional printing of complex biological structures by freeform reversible embedding of suspended hydrogels. Sci Adv, 1(9): e1500758. https://doi.org/10.1126/sciadv.1500758
- Augustine R, Nuthana Kalva S, Ahmed R, et al., 2021, 3D bioprinted cancer models: Revolutionizing personalized cancer therapy. Transl Oncol, 14(4): 101015. https://doi.org/10.1016/j.tranon.2021.101015
- Xu F, Celli J, Rizvi I, et al., 2011, A three-dimensional in vitro ovarian cancer coculture model using a high-throughput cell patterning platform. Biotechnol J, 6(2): 204–212. https://doi.org/10.1002/biot.201000340
- Chang R, Nam J, Sun W, 2008, Direct cell writing of 3D microorgan for in vitro pharmacokinetic model. Tissue Eng Part C Methods, 14(2): 157–166. https://doi.org/10.1089/ten.tec.2007.0392
- Norotte C, Marga FS, Niklason LE, et al., 2009, Scaffold-free vascular tissue engineering using bioprinting. Biomaterials, 30(30): 5910–5917. https://doi.org/10.1016/j.biomaterials.2009.06.034
- Ren Y, Yang X, Ma Z, et al., 2021, Developments and opportunities for 3D bioprinted organoids. Int J Bioprint, 7(3): 364. https://doi.org/10.18063/ijb.v7i3.364
- Leberfinger AN, Ravnic DJ, Dhawan A, et al., 2017, Concise review: Bioprinting of stem cells for transplantable tissue fabrication. Stem Cell Transl Med, 6(10): 1940–1948. https://doi.org/10.1002/sctm.17-0148
- Lam EHY, Yu F, Zhu S, et al., 2023, 3D-bioprinting for next-generation personalized medicine. Int J Mol Sci, 24(7): 6357. https://doi.org/10.3390/ijms24076357
- Chansoria P, Schuchard K, Shirwaiker RA, 2021, Process hybridization schemes for multiscale engineered tissue biofabrication. WIREs Nanomed Nanobiotechnol, 13(2): e1673. https://doi.org/10.1002/wnan.1673
- Kang B, Shin J, Park H-J, et al., 2018, High-resolution acoustophoretic 3D cell patterning to construct functional collateral cylindroids for ischemia therapy. Nat Commun, 9(1): 1–13. https://doi.org/10.1038/s41467-018-07823-5
- Morita T, Watanabe S, Sasaki S, 2023, Multiaxis printing method for bent tubular structured gels in support bath for achieving high dimension and shape accuracy. Precis Eng, 79: 109–118. https://doi.org/10.1016/j.precisioneng.2022.09.004
- Wang X, 2013, Overview on biocompatibilities of implantable biomaterials, in Advances in Biomaterials Science and Biomedical Applications, IntechOpen, Vienna, Austria. https://doi.org/10.5772/53461
- Shao L, Gao Q, Xie C, et al., 2020, Sacrificial microgel-laden bioink-enabled 3D-Bioprinting of mesoscale pore networks. Bio-Des Manuf, 3(1): 30–39. https://doi.org/10.1007/s42242-020-00062-y
- Brunel LG, Hull SM, Heilshorn SC, 2022, Engineered assistive materials for 3D-Bioprinting: Support baths and sacrificial inks. Biofabrication, 14(3): 032001. https://doi.org/10.1088/1758-5090/ac6bbe
- Lee JM, Sing SL, Zhou M, et al., 2018, 3D-Bioprinting processes: A perspective on classification and terminology. Int J Bioprint, 4(2). https://doi.org/10.18063/ijb.v4i2.151