Recent progress on 3D-printed gelatin methacrylate-based biomaterials for articular cartilage repair
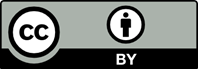
The structure and composition of articular cartilage is complex, and its self-healing ability is limited, and thus, it is difficult to achieve ideal healing once the articular cartilage is damaged. Recently, three-dimensional (3D) printing technology has provided a new possibility for the repair of articular cartilage. Engineered cartilage tissues can be fabricated by superimposing customized inks, considering different geometric structures and components of tissues. 3D printing can be effectively used to manufacture high-precision structures with complex geometry, solving the shortcomings of traditional scaffold fabrication techniques. Gelatin methacrylate (GelMA) is modified gelatin and is currently a widely used 3D printing ink due to its photocrosslinking properties. With good biocompatibility and tunable physical properties, it can provide a good scaffold platform for cell proliferation and growth factor release. Given that the role of 3D printing technology in cartilage repair has been widely reported, this article reviews the research progress of 3D-printed GelMA-based biomaterials in articular cartilage tissue engineering. We focus primarily on how 3D printing technology addresses the existing challenges inherent to the field of articular cartilage tissue engineering. We accentuate the modifications implemented in GelMA-based 3D printing scaffolds to optimize articular cartilage regeneration. Additionally, we provide a comprehensive summary of the utilization of GelMA-based biomaterials incorporating various cells, growth factors, or other tissue components and highlight how these adaptations, in conjunction with the benefits of 3D printing technology, facilitate improvements the articular cartilage repair.
- Ning L, Chen X, 2017, A brief review of extrusion-based tissue scaffold bio-printing. Biotechnol J, 12(8).
- Daly AC, Freeman FE, Gonzalez-Fernandez T, et al., 2017, 3D bioprinting for cartilage and osteochondral tissue engineering. Adv Healthc Mater, 6(22).
- Wu Y, Kennedy P, Bonazza N, et al., 2021, Three-dimensional bioprinting of articular cartilage: A systematic review. Cartilage, 12(1): 76–92.
- Messaoudi O, Henrionnet C, Bourge K, et al., 2020, Stem cells and extrusion 3D printing for hyaline cartilage engineering. Cells, 10(1).
- Oveissi F, Naficy S, Lee A, et al., 2020, Materials and manufacturing perspectives in engineering heart valves: A review. Mater Today Bio, 5: 100038.
- Zhang Y, Chen H, Li J, 2022, Recent advances on gelatin methacrylate hydrogels with controlled microstructures for tissue engineering. Int J Biol Macromol, 221: 91–107.
- Yilmaz B, Tahmasebifar A, Baran ET, 2020, Bioprinting technologies in tissue engineering. Adv Biochem Eng/ Biotechnol, 171: 279–319.
- Derakhshanfar S, Mbeleck R, Xu K, et al., 2018, 3D bioprinting for biomedical devices and tissue engineering: A review of recent trends and advances. Bioact Mater, 3(2): 144–156.
- Gorgieva S, Kokol V, 2011, Collagen- vs. gelatine-based biomaterials and their biocompatibility: Review and perspectives, in Biomaterials Applications for Nanomedicine, Norderstedt.
- Vandooren J, Van den Steen PE, Opdenakker G, et al., 2013, Biochemistry and molecular biology of gelatinase B or matrix metalloproteinase-9 (MMP-9). Crit Rev Biochem Mol Biol, 48(3): 222–72.
- Ozbolat IT, Hospodiuk M, 2016, Current advances and future perspectives in extrusion-based bioprinting. Biomaterials, 76(37): 321–343.
- Van Den Bulcke AI, Bogdanov B, De Rooze N, et al., 2000, Structural and rheological properties of methacrylamide modified gelatin hydrogels. Biomacromolecules, 1(1): 31–38.
- Kumar H, Sakthivel K, Mohamed MGA, et al., 2021, Designing gelatin methacryloyl (GelMA)-based bioinks for visible light stereolithographic 3D biofabrication. Macromol Biosci, 21(1): e2000317.
- Sun M, Sun X, Wang Z, et al., 2018, Synthesis and properties of gelatin methacryloyl (GelMA) hydrogels and their recent applications in load-bearing tissue. Polymers(Basel), 10(11): 1290.
- Schuurman W, Levett PA, Pot MW, et al., 2013, Gelatin-methacrylamide hydrogels as potential biomaterials for fabrication of tissue-engineered cartilage constructs. Macromol Biosci, 13(5): 551–561.
- Gan D, Xu T, Xing W, et al., 2019, Mussel-inspired dopamine oligomer intercalated tough and resilient gelatin methacryloyl (GelMA) hydrogels for cartilage regeneration. J Mater Chem B, 7(10): 1716–1725.
- Lim KS, Abinzano F, Bernal PN, et al., 2020, One-step photoactivation of a dual-functionalized bioink as cell carrier and cartilage-binding glue for chondral regeneration. Adv Healthc Mater, 9(15): e1901792.
- Wang M, Zhao J, Luo Y, et al., 2022, 3D contour printing of anatomically mimetic cartilage grafts with microfiber-reinforced double-network bioink. Macromol Biosci, 22(9): e2200179.
- Guan J, Yuan FZ, Mao ZM, et al., 2021, Fabrication of 3D-printed interpenetrating hydrogel scaffolds for promoting chondrogenic differentiation. Polymers (Basel), 13(13):2146.
- Han L , Xu J , Lu X, et al., 2017, Biohybrid methacrylated gelatin/polyacrylamide hydrogels for cartilage repair. J Mater Chem B, 5(4): 731–741.
- Trengove A, Duchi S, Onofrillo C, et al., 2021, Microbial transglutaminase improves ex vivo adhesion of gelatin methacryloyl hydrogels to human cartilage. Front Med Technol, 3: 773673.
- Suo H, Xu K, Zheng X, 2015, Using glucosamine to improve the properties of photocrosslinked gelatin scaffolds. J Biomater Appl, 29(7): 977–987.
- Suo H, Li L, Zhang C, et al., 2020, Glucosamine-grafted methacrylated gelatin hydrogels as potential biomaterials for cartilage repair. J Biomed Mater Res B Appl Biomater, 108(3): 990–999.
- Zhan X, 2020, Effect of matrix stiffness and adhesion ligand density on chondrogenic differentiation of mesenchymal stem cells. J Biomed Mater Res A, 108(3): 675–683.
- Brown GCJ, Lim KS, Farrugia BL, et al., 2017, Covalent incorporation of heparin improves chondrogenesis in photocurable gelatin-methacryloyl hydrogels. Macromol Biosci, 17(12): 1700158.
- Wang KY, Jin XY, Ma YH, et al., 2021, Injectable stress relaxation gelatin-based hydrogels with positive surface charge for adsorption of aggrecan and facile cartilage tissue regeneration. J Nanobiotechnol, 19(1): 214.
- Huang B, Li P, Chen M, et al., 2022, Hydrogel composite scaffolds achieve recruitment and chondrogenesis in cartilage tissue engineering applications. J Nanobiotechnol, 20(1): 25.
- Li X, Chen S, Li J, et al., 2016, 3D culture of chondrocytes in gelatin hydrogels with different stiffness. Polymers (Basel), 8(8): 269.
- Li X, Chen Y, Kawazoe N, et al., 2017, Influence of microporous gelatin hydrogels on chondrocyte functions. J Mater Chem B, 5(29): 5753–5762.
- Costantini M, Idaszek J, Szöke K, et al., 2016, 3D bioprinting of BM-MSCs-loaded ECM biomimetic hydrogels for in vitro neocartilage formation. Biofabrication, 8(3): 035002.
- Shopperly LK, Spinnen J, Krüger JP, et al., 2022, Blends of gelatin and hyaluronic acid stratified by stereolithographic bioprinting approximate cartilaginous matrix gradients. J Biomed Mater Res B Appl Biomater, 110(10): 2310–2322.
- Huang K, Li Q, Li Y, et al., 2018, Cartilage tissue regeneration: The roles of cells, stimulating factors and scaffolds. Curr Stem Cell Res Ther, 13(7): 547–567.
- Chen L, Liu J, Guan M, et al., 2020, Growth factor and its polymer scaffold-based delivery system for cartilage tissue engineering. Int J Nanomed, 15: 6097–6111.
- Wang B, Díaz-Payno PJ, Browe DC, et al., 2021, Affinity-bound growth factor within sulfated interpenetrating network bioinks for bioprinting cartilaginous tissues. Acta Biomater, 128: 130–142.
35. Cho H, Kim J, Kim S, et al., 2020, Dual delivery of stem cells and insulin-like growth factor-1 in coacervate-embedded composite hydrogels for enhanced cartilage regeneration in osteochondral defects. J Control Release, 327: 284–295.
- Zhang Z, Li L, Yang W, et al., 2017, The effects of different doses of IGF-1 on cartilage and subchondral bone during the repair of full-thickness articular cartilage defects in rabbits. Osteoarthr Cartil, 25(2): 309–320.
- Wu H, Shang Y, Sun W, et al., 2023, Seamless and early gap healing of osteochondral defects by autologous mosaicplasty combined with bioactive supramolecular nanofiber-enabled gelatin methacryloyl (BSN-GelMA) hydrogel. Bioact Mater, 19: 88–102.
- Zhu W, Cui H, Boualam B, et al., 2018, 3D bioprinting mesenchymal stem cell-laden construct with core-shell nanospheres for cartilage tissue engineering. Nanotechnology, 29(18): 185101.
- Wang B, Díaz-Payno PJ, Browe DC, et al., 2021, Affinity-bound growth factor within sulfated interpenetrating network bioinks for bioprinting cartilaginous tissues. Acta Biomater, 128: 130–142.
- Yang Z, Cao F, Li H, et al., 2022, Microenvironmentally optimized 3D-printed TGFβ-functionalized scaffolds facilitate endogenous cartilage regeneration in sheep. Acta Biomater, 150: 181–198.
- Ding X, Gao J, Yu X, et al., 2022, 3D-printed porous scaffolds of hydrogels modified with TGF-β1 binding peptides to promote in vivo cartilage regeneration and animal gait restoration. ACS Appl Mater Interfaces, 14(14): 15982– 15995.
- van Beuningen HM, Glansbeek HL, van der Kraan PM, et al., 2000, Osteoarthritis-like changes in the murine knee joint resulting from intra-articular transforming growth factor-beta injections. Osteoarthr Cartil, 8(1): 25–33.
- Bakker AC, van de Loo FA, van Beuningen HM, et al., 2001, Overexpression of active TGF-beta-1 in the murine knee joint: evidence for synovial-layer-dependent chondro-osteophyte formation. Osteoarthr Cartil, 9(2): 128–136.
- Gong L, Li J, Zhang J, et al., 2020, An interleukin-4-loaded bi-layer 3D printed scaffold promotes osteochondral regeneration. Acta Biomater, 117(Nov): 246–260.
- Liang Y, Li J, Wang Y, et al., Platelet Rich Plasma in the Repair of Articular Cartilage Injury: A Narrative Review. Cartilage, 2022. 13(3): p. 19476035221118419.
- Everts P, Onishi K, Jayaram P, et al., 2020, Platelet-rich plasma: new performance understandings and therapeutic considerations in 2020. Int J Mol Sci, 21(20): 7749.
- Szwedowski D, Szczepanek J, Paczesny Ł, et al., 2021, The effect of platelet-rich plasma on the intra-articular microenvironment in knee osteoarthritis. Int J Mol Sci, 22(11): 5492.
- Qian Y, Han Q, Chen W, et al., 2017, Platelet-rich plasma derived growth factors contribute to stem cell differentiation in musculoskeletal regeneration. Front Chem, 5: 89.
- Jiang G, Li S, Yu K, et al., 2021, A 3D-printed PRP-GelMA hydrogel promotes osteochondral regeneration through M2 macrophage polarization in a rabbit model. Acta Biomater, 128: 150–162.
- Irmak G, Gümüşderelioğlu M, 2020, Photo-activated platelet-rich plasma (PRP)-based patient-specific bio-ink for cartilage tissue engineering. Biomed Mater, 15(6): 065010.
- Irmak G, Gümüşderelioğlu M, 2021, Patients- and tissue-specific bio-inks with photoactivated PRP and methacrylated gelatin for the fabrication of osteochondral constructs. Mater Sci Eng C Mater Biol Appl, 125: 112092.
- Zheng K, Zheng X, Yu M, et al., 2023, BMSCs-seeded interpenetrating network GelMA/SF composite hydrogel for articular cartilage repair. J Funct Biomater, 14(1).
- Ma Q, Liao J, Cai X, 2018, Different sources of stem cells and their application in cartilage tissue engineering. Curr Stem Cell Res Ther, 13(7): 568–575.
- Pirosa A, Gottardi R, Alexander PG, et al., 2021, An in vitro chondro-osteo-vascular triphasic model of the osteochondral complex. Biomaterials, 272: 120773.
- Liu F, Wang X, Li Y, et al., 2022, Dendrimer-modified gelatin methacrylate hydrogels carrying adipose-derived stromal/ stem cells promote cartilage regeneration. Stem Cell Res Ther, 13(1): 26.
- Jiang Y, Tuan RS, 2015, Origin and function of cartilage stem/progenitor cells in osteoarthritis. Nat Rev Rheumatol, 11(4): 206–212.
- Kozhemyakina E, Zhang M, Ionescu A, et al., 2015, Identification of a Prg4-expressing articular cartilage progenitor cell population in mice. Arthritis Rheumatol, 67(5): 1261–1273.
- Levato R, Webb WR, Otto IA, et al., 2017, The bio in the ink: Cartilage regeneration with bioprintable hydrogels and articular cartilage-derived progenitor cells. Acta Biomater, 61: 41–53.
- Mouser VHM, Levato R, Mensinga A, et al., 2020, Bio-ink development for three-dimensional bioprinting of hetero-cellular cartilage constructs. Connect Tissue Res, 61(2): 137– 151.
- Gao G, Schilling AF, Hubbell K, et al., 2015, Improved properties of bone and cartilage tissue from 3D inkjet-bioprinted human mesenchymal stem cells by simultaneous deposition and photocrosslinking in PEG-GelMA. Biotechnol Lett, 37(11): 2349–2355.
- Luo C, Xie R, Zhang J, et al., 2020, Low-temperature three-dimensional printing of tissue cartilage engineered with gelatin methacrylamide. Tissue Eng Part C Methods, 26(6): 306–316.
- Shah SS, Mithoefer K, 2021, Scientific developments and clinical applications utilizing chondrons and chondrocytes with matrix for cartilage repair. Cartilage, 13(1_suppl): 1195s–1205s.
- Levett PA, Melchels FP, Schrobback K, et al., 2014, Chondrocyte redifferentiation and construct mechanical property development in single-component photocrosslinkable hydrogels. J Biomed Mater Res Part A, 102(8): 2544–2553.
- Hölzl K, Fürsatz M, Göcerler H, et al., 2022, Gelatin methacryloyl as environment for chondrocytes and cell delivery to superficial cartilage defects. J Tissue Eng Regen Med, 16(2): 207–222.
- Wang G, An Y, Zhang X, et al., 2021, Chondrocyte spheroids laden in GelMA/HAMA hybrid hydrogel for tissue-engineered cartilage with enhanced proliferation, better phenotype maintenance, and natural morphological structure. Gels, 7(4): 247.
- Levett PA, Melchels FP, Schrobback K, et al., 2014, A biomimetic extracellular matrix for cartilage tissue engineering centered on photocurable gelatin, hyaluronic acid and chondroitin sulfate. Acta Biomater, 10(1): 214–223.
- Agten H, Van Hoven I, Viseu SR, et al., 2022, In vitro and in vivo evaluation of 3D constructs engineered with human iPSC-derived chondrocytes in gelatin methacryloyl hydrogel. Biotechnol Bioeng, 119(10): 2950–2963.
- Tang P, Song P, Peng Z, et al., 2021, Chondrocyte-laden GelMA hydrogel combined with 3D printed PLA scaffolds for auricle regeneration. Mater Sci Eng C Mater Biol Appl, 130: 112423.
- Chen P, Zheng L, Wang Y, et al., 2019, Desktop-stereolithography 3D printing of a radially oriented extracellular matrix/ mesenchymal stem cell exosome bioink for osteochondral defect regeneration. Theranostics, 9(9): 2439–2459.
- Cucchiarini M, Madry H, Guilak F, et al., 2014, A vision on the future of articular cartilage repair. Eur Cell Mater, 27: 12–16.
- Cheng J, Rong G, Wang Z, et al., 2022, ECM-mimicking hydrogels loaded with bone mesenchymal stem cell-derived exosomes for the treatment of cartilage defects. Evid Based Complement Alternat Med, 2022: 3450672.
- Guan P, Cui R, Wang Q, et al., 2022, A 3D hydrogel loaded with exosomes derived from bone marrow stem cells promotes cartilage repair in rats by modulating immunological microenvironment. Nan Fang Yi Ke Da Xue Xue Bao, 42(4): 528–537.