A new 3D-printed polylactic acid-bioglass composite for bone tissue engineering induces angiogenesis in vitro and in ovo
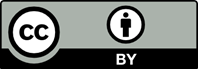
Large bone defects such as those that occur after trauma or resections due to cancer still are a challenge for surgeons. Main challenge in this area is to find a suitable alternative to the gold-standard therapy, which is highly risky, and a promising option is to use biomaterials manufactured by 3D printing. In former studies, we demonstrated that the combination of polylactic acid (PLA) and bioglass (BG) resulted in a stable 3D-printable material, and porous and finely structured scaffolds were printed. These scaffolds exhibited osteogenic and anti-inflammatory properties. This 3D-printed material fulfills most of the requirements described in the diamond concept of bone healing. However, the question remains as to whether it also meets the requirements concerning angiogenesis. Therefore, the aim of this study was to analyze the effects of the 3D-printed PLA-BG composite material on angiogenesis. In vitro analyses with human umbilical vein endothelial cells (HUVECs) showed a positive effect of increasing BG content on viability and gene expression of endothelial markers. This positive effect was confirmed by an enhanced vascular formation analyzed by Matrigel assay and chicken chorioallantoic membrane (CAM) assay. In this work, we demonstrated the angiogenic efficiency of a 3D-printed PLA–BG composite material. Recalling the osteogenic potential of this material demonstrated in former work, we manufactured a mechanically stable, 3D-printable, osteogenic and angiogenic material, which could be used for bone tissue engineering.
Karalashvili L, Kakabadze A, Uhryn M, et al., 2018, Bone grafts for reconstruction of bone defects (review). Georgian Med News, 282:44–49.
Schmidt AH, 2021, Autologous bone graft: Is it still the gold standard? Injury, 52(Suppl 2):S18–S22. https://doi.org/10.1016/j.injury.2021.01.043
Arif ZU, Khalid MY, Noroozi R, et al., 2022, Recent advances in 3D-printed polylactide and polycaprolactone-based biomaterials for tissue engineering applications. Int J Biol Macromol, 218:930–968. https://doi.org/10.1016/j.ijbiomac.2022.07.140
Kaya I, Sahin MC, Cingoz ID, et al., 2019, Three dimensional printing and biomaterials in the repairment of bone defects; hydroxyapatite PLA filaments. Turk J Med Sci, 49(3):922–927. https://doi.org/10.3906/sag-1901-184
Liu T, Li B, Chen G, et al., 2022, Nano tantalum-coated 3D printed porous polylactic acid/beta-tricalcium phosphate scaffolds with enhanced biological properties for guided bone regeneration. Int J Biol Macromol, 221:371–380. https://doi.org/10.1016/j.ijbiomac.2022.09.003
Alksne M, Kalvaityte M, Simoliunas E, et al., 2020, In vitro comparison of 3D printed polylactic acid/hydroxyapatite and polylactic acid/bioglass composite scaffolds: Insights into materials for bone regeneration. J Mech Behav Biomed Mater, 104:103641. https://doi.org/10.1016/j.jmbbm.2020.103641
Hench LL, Jones JR, 2015, Bioactive glasses: Frontiers and challenges. Front Bioeng Biotechnol, 3:194.
https://doi.org/10.3389/fbioe.2015.00194
Zare S, Mohammadpour M, Izadi Z, et al., 2022, Nanofibrous hydrogel nanocomposite based on strontium-doped bioglass nanofibers for bone tissue engineering applications. Biology (Basel), 11(10). https://doi.org/10.3390/biology11101472
Kumari S, Singh D, Srivastava P, et al., 2022, Generation of graphene oxide and nano-bioglass based scaffold for bone tissue regeneration. Biomed Mater, 17(6). https://doi.org/10.1088/1748-605X/ac92b4
Kukulka EC, de Souza JR, de Araujo JCR, et al., 2023, Polycaprolactone/chlorinated bioglass scaffolds doped with Mg and Li ions: Morphological, physicochemical, and biological analysis. J Biomed Mater Res B Appl Biomater, 111(1):140–150. https://doi.org/10.1002/jbm.b.35140
Daskalakis E, Huang B, Vyas C, et al., 2022, Novel 3D bioglass scaffolds for bone tissue regeneration. Polymers (Basel), 14(3). https://doi.org/10.3390/polym14030445
Blaker JJ, Gough JE, Maquet V, et al., 2003, In vitro evaluation of novel bioactive composites based on bioglass-filled polylactide foams for bone tissue engineering scaffolds. J Biomed Mater Res A, 67(4):1401–1411. https://doi.org/10.1002/jbm.a.20055
Maquet V, Boccaccini AR, Pravata L, et al., 2003, Preparation, characterization, and in vitro degradation of bioresorbable and bioactive composites based on bioglass-filled polylactide foams. J Biomed Mater Res A, 66(2):335–346. https://doi.org/10.1002/jbm.a.10587
Roether JA, Boccaccini AR, Hench LL, et al., 2002, Development and in vitro characterisation of novel bioresorbable and bioactive composite materials based on polylactide foams and bioglass for tissue engineering applications. Biomaterials, 23(18):3871–3878. https://doi.org/10.1016/s0142-9612(02)00131-x
Lyyra I, Leino K, Hukka T, et al., 2021, Impact of glass composition on hydrolytic degradation of polylactide/ bioactive glass composites. Materials (Basel), 14(3). https://doi.org/10.3390/ma14030667
Söhling N, Neijhoft J, Nienhaus V, et al., 2020, 3D-printing of hierarchically designed and osteoconductive bone tissue engineering scaffolds. Materials (Basel), 13(8). https://doi.org/10.3390/ma13081836
Schätzlein E, Kicker C, Söhling N, et al., 2022, 3D-printed PLA-bioglass scaffolds with controllable calcium release and MSC adhesion for bone tissue engineering. Polymers (Basel), 14(12). https://doi.org/10.3390/polym14122389
Söhling N, Al Zoghool S, Schätzlein E, et al., 2022, In vitro evaluation of a 20% bioglass-containing 3D printable PLA composite for bone tissue engineering. Int J Bioprint, 8(4):602. https://doi.org/10.18063/ijb.v8i4.602
Giannoudis PV, Atkins R, 2007, Management of long-bone non-unions. Injury, 38(Suppl 2):S1–S2.
Andrzejowski P, Giannoudis PV, 2019, The ‘diamond concept’ for long bone non-union management. J Orthop Traumatol, 20(1):21. https://doi.org/10.1186/s10195-019-0528-0
Mahapatra C, Kumar P, Paul MK, et al., 2022, Angiogenic stimulation strategies in bone tissue regeneration. Tissue Cell, 79:101908. https://doi.org/10.1016/j.tice.2022.101908
Rather HA, Jhala D, Vasita R, 2019, Dual functional approaches for osteogenesis coupled angiogenesis in bone tissue engineering. Mater Sci Eng C Mater Biol Appl, 103:109761. https://doi.org/10.1016/j.msec.2019.109761
Tsiridis E, Upadhyay N, Giannoudis P, 2007, Molecular aspects of fracture healing: Which are the important molecules? Injury, 38(Suppl 1):S11–S25. https://doi.org/10.1016/j.injury.2007.02.006
Zhao D, Zhu T, Li J, et al., 2021, Poly(lactic-co-glycolic acid)-based composite bone-substitute materials. Bioact Mater, 6(2):346–360. https://doi.org/10.1016/j.bioactmat.2020.08.016
Zhu T, Jiang M, Zhang M, et al., 2022, Biofunctionalized composite scaffold to potentiate osteoconduction, angiogenesis, and favorable metabolic microenvironment for osteonecrosis therapy. Bioact Mater, 9:446–460. https://doi.org/10.1016/j.bioactmat.2021.08.005
Li H, He J, Yu H, et al., 2016, Bioglass promotes wound healing by affecting gap junction connexin 43 mediated endothelial cell behavior. Biomaterials, 84:64–75. https://doi.org/10.1016/j.biomaterials.2016.01.033
Handel M, Hammer TR, Nooeaid P, et al., 2013, 45S5- bioglass((R))-based 3D-scaffolds seeded with human adipose tissue-derived stem cells induce in vivo vascularization in the CAM angiogenesis assay. Tissue Eng Part A, 19(23–24):2703–2712. https://doi.org/10.1089/ten.TEA.2012.0707
Deb S, Mandegaran R, Di Silvio L, 2010, A porous scaffold for bone tissue engineering/45S5 bioglass derived porous scaffolds for co-culturing osteoblasts and endothelial cells. J Mater Sci Mater Med, 21(3):893–905. https://doi.org/10.1007/s10856-009-3936-5
Stähli C, James-Bhasin M, Hoppe A, et al., 2015, Effect of ion release from Cu-doped 45S5 bioglass(R) on 3D endothelial cell morphogenesis. Acta Biomater, 19:15–22. https://doi.org/10.1016/j.actbio.2015.03.009
Eldesoqi K, Seebach C, Nguyen Ngoc C, et al., 2013, High calcium bioglass enhances differentiation and survival of endothelial progenitor cells, inducing early vascularization in critical size bone defects. PLoS One, 8(11):e79058. https://doi.org/10.1371/journal.pone.0079058
Barbeck M, Serra T, Booms P, et al., 2017, Analysis of the in vitro degradation and the in vivo tissue response to bi-layered 3D-printed scaffolds combining PLA and biphasic PLA/bioglass components—Guidance of the inflammatory response as basis for osteochondral regeneration. Bioact Mater, 2(4):208–223. https://doi.org/10.1016/j.bioactmat.2017.06.001
Livak KJ, Schmittgen TD, 2001, Analysis of relative gene expression data using real-time quantitative PCR and the 2(-Delta Delta C(T)) method. Methods, 25(4):402–408. https://doi.org/10.1006/meth.2001.1262
Carpentier G, Berndt S, Ferratge S, et al., 2020, Angiogenesis analyzer for ImageJ—A comparative morphometric analysis of “Endothelial Tube Formation Assay” and “Fibrin Bead Assay”. Sci Rep, 10(1):11568. https://doi.org/10.1038/s41598-020-67289-8
Buhr CR, Wiesmann N, Tanner RC, et al., 2020, The chorioallantoic membrane assay in nanotoxicological research—An alternative for in vivo experimentation. Nanomaterials (Basel), 10(12). https://doi.org/10.3390/nano10122328
Ribatti D, Annese T, Tamma R, 2020, The use of the chick embryo CAM assay in the study of angiogenic activiy of biomaterials. Microvasc Res, 131:104026. https://doi.org/10.1016/j.mvr.2020.104026
Yu Y, Yang B, Tian D, et al., 2022, Thiolated hyaluronic acid/silk fibroin dual-network hydrogel incorporated with bioglass nanoparticles for wound healing. Carbohydr Polym, 288:119334. https://doi.org/10.1016/j.carbpol.2022.119334
Wu Z, He D, Li H, 2021, Bioglass enhances the production of exosomes and improves their capability of promoting vascularization. Bioact Mater, 6(3):823–835. https://doi.org/10.1016/j.bioactmat.2020.09.011
El-Gendy R, Kirkham J, Newby PJ, et al., 2015, Investigating the vascularization of tissue-engineered bone constructs using dental pulp cells and 45S5 bioglass(R) scaffolds. Tissue Eng Part A, 21(13–14):2034–2043. https://doi.org/10.1089/ten.tea.2014.0485
El-Gendy R, Yang XB, Newby PJ, et al., 2013, Osteogenic differentiation of human dental pulp stromal cells on 45S5 bioglass(R) based scaffolds in vitro and in vivo. Tissue Eng Part A, 19(5–6):707–715. https://doi.org/10.1089/ten.TEA.2012.0112
Ponce ML, 2009, Tube formation: An in vitro Matrigel angiogenesis assay. Methods Mol Biol, 467:183–188. https://doi.org/10.1007/978-1-59745-241-0_10
Benton G, Arnaoutova I, George J, et al., 2014, Matrigel: From discovery and ECM mimicry to assays and models for cancer research. Adv Drug Deliv Rev, 79-80:3–18. https://doi.org/10.1016/j.addr.2014.06.005
Fischer D, Fluegen G, Garcia P, et al., 2022, The CAM model- Q&A with experts. Cancers (Basel), 15(1). https://doi.org/10.3390/cancers15010191
Weber J, Weber M, Steinle H, et al., 2021, An alternative in vivo model to evaluate pluripotency of patient-specific iPSCs. ALTEX, 38(3):442–450. https://doi.org/10.14573/altex.2005221
Ribatti D, 2014, The chick embryo chorioallantoic membrane as a model for tumor biology. Exp Cell Res, 328(2):314–324. https://doi.org/10.1016/j.yexcr.2014.06.010
Hagedorn M, Balke M, Schmidt A, et al., 2004, VEGF coordinates interaction of pericytes and endothelial cells during vasculogenesis and experimental angiogenesis. Dev Dyn, 230(1):23–33. https://doi.org/10.1002/dvdy.20020
Cohrs NH, Schulz-Schonhagen K, Mohn D, et al., 2019, Modification of silicone elastomers with bioglass 45S5(R) increases in ovo tissue biointegration. J Biomed Mater Res B Appl Biomater, 107(4):1180–1188. https://doi.org/10.1002/jbm.b.34211
Vargas GE, Mesones RV, Bretcanu O, et al., 2009, Biocompatibility and bone mineralization potential of 45S5 bioglass-derived glass-ceramic scaffolds in chick embryos. Acta Biomater, 5(1):374–380. https://doi.org/10.1016/j.actbio.2008.07.016
Mangir N, Dikici S, Claeyssens F, et al., 2019, Using ex ovo chick chorioallantoic membrane (CAM) assay to evaluate the biocompatibility and angiogenic response to biomaterials. ACS Biomater Sci Eng, 5(7):3190–3200. https://doi.org/10.1021/acsbiomaterials.9b00172
Baiguera S, Macchiarini P, Ribatti D, 2012, Chorioallantoic membrane for in vivo investigation of tissue-engineered construct biocompatibility. J Biomed Mater Res B Appl Biomater, 100(5):1425–1434. https://doi.org/10.1002/jbm.b.32653
Brezulier D, Chaigneau L, Jeanne S, et al., 2021, The challenge of 3D bioprinting of composite natural polymers PLA/bioglass: Trends and benefits in cleft palate surgery. Biomedicines, 9(11). https://doi.org/10.3390/biomedicines9111553
Kasten P, Beyen I, Niemeyer P, et al., 2008, Porosity and pore size of beta-tricalcium phosphate scaffold can influence protein production and osteogenic differentiation of human mesenchymal stem cells: An in vitro and in vivo study. Acta Biomater, 4(6):1904–1915. https://doi.org/10.1016/j.actbio.2008.05.017
Hoemann CD, Rodriguez Gonzalez J, Guzman-Morales J, et al., 2022, Chitosan coatings with distinct innate immune bioactivities differentially stimulate angiogenesis, osteogenesis and chondrogenesis in poly-caprolactone scaffolds with controlled interconnecting pore size. Bioact Mater, 10:430–442. https://doi.org/10.1016/j.bioactmat.2021.09.012
Zhang J, Tong D, Song H, et al., 2022, Osteoimmunity-regulating biomimetically hierarchical scaffold for augmented bone regeneration. Adv Mater, 34(36):e2202044. https://doi.org/10.1002/adma.202202044
Finotti PF, Costa LC, Capote TS, et al., 2017, Immiscible poly(lactic acid)/poly(epsilon-caprolactone) for temporary implants: Compatibility and cytotoxicity. J Mech Behav Biomed Mater, 68:155–162. https://doi.org/10.1016/j.jmbbm.2017.01.050
Diomede F, Gugliandolo A, Cardelli P, et al., 2018, Three-dimensional printed PLA scaffold and human gingival stem cell-derived extracellular vesicles: A new tool for bone defect repair. Stem Cell Res Ther, 9(1):104. https://doi.org/10.1186/s13287-018-0850-0
Carvalho JRG, Conde G, Antonioli ML, et al., 2021, Long-term evaluation of poly(lactic acid) (PLA) implants in a horse: An experimental pilot study. Molecules, 26(23). https://doi.org/10.3390/molecules26237224
Fernandes HR, Gaddam A, Rebelo A, et al., 2018, Bioactive glasses and glass-ceramics for healthcare applications in bone regeneration and tissue engineering. Materials (Basel), 11(12). https://doi.org/10.3390/ma11122530
Hoppe A, Guldal NS, Boccaccini AR, 2011, A review of the biological response to ionic dissolution products from bioactive glasses and glass-ceramics. Biomaterials, 32(11):2757–2774. https://doi.org/10.1016/j.biomaterials.2011.01.004
Zhou P, Garcia BL, Kotsakis GA, 2022, Comparison of antibacterial and antibiofilm activity of bioactive glass compounds S53P4 and 45S5. BMC Microbiol, 22(1):212. https://doi.org/10.1186/s12866-022-02617-8