Biomechanical properties of 3D printable material usable for synthetic personalized healthy human aorta
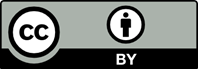
With the development of three-dimensional (3D) printing, 3D-printed products have been widely used in medical fields, such as plastic surgery, orthopedics, dentistry, etc. In cardiovascular research, 3D-printed models are becoming more realistic in shape. However, from a biomechanical point of view, only a few studies have explored printable materials that can represent the properties of the human aorta. This study focuses on 3D-printed materials that might simulate the stiffness of human aortic tissue. First, the biomechanical properties of a healthy human aorta were defined and used as reference. The main objective of this study was to identify 3D printable materials that possess similar properties to the human aorta. Three synthetic materials, NinjaFlex (Fenner Inc., Manheim, USA), FilasticTM (Filastic Inc., Jardim Paulistano, Brazil), and RGD450+TangoPlus (Stratasys Ltd.©, Rehovot, Israel), were printed in different thicknesses. Uniaxial and biaxial tensile tests were performed to compute several biomechanical properties, such as thickness, stress, strain, and stiffness. We found that with the mixed material RGD450+TangoPlus, it was possible to achieve a similar stiffness to healthy human aorta. Moreover, the 50-shore-hardness RGD450+TangoPlus had similar thickness and stiffness to the human aorta.
1. Ploch CC, Mansi CSSA, Jayamohan J, et al., 2016, Using 3D printing to create personalized brain models for neurosurgical training and preoperative planning. World Neurosurg, 90:668–674. https://doi.org/10.1016/j.wneu.2016.02.081
2. Hochman JB, Rhodes C, Wong D, et al., 2015, Comparison of cadaveric and isomorphic three‐dimensional printed models in temporal bone education. Laryngoscope, 125(10):2353–2357. https://doi.org/10.1002/lary.24919
3. Lynn AQ, Pflibsen LR, Smith AA, et al., 2021, Three-dimensional printing in plastic surgery: Current applications, future directions, and ethical implications. Plast Reconstr Surg Glob Open, 9(3):e3465. https://doi.org/10.1097/GOX.0000000000003465
4. Lal H., Patralekh MK, 2018, 3D printing and its applications in orthopaedic trauma: A technological marvel. J Clin Orthop Trauma, 9(3):260–268. https://doi.org/10.1016/j.jcot.2018.07.022
5. Khorsandi D, Fahimipour A, Abasian P, et al., 2021, 3D and 4D printing in dentistry and maxillofacial surgery: Printing techniques, materials, and applications. Acta Biomater, 122:26–49. https://doi.org/10.1016/j.actbio.2020.12.044
6. Wang Z, Wang L, Li T, et al., 2021, 3D bioprinting in cardiac tissue engineering. Theranostics, 11(16):7948–7969. https://doi.org/10.7150/thno.61621
7. Brantner P, Madaffari A, Fahrni G, et al., 2020, 3D-printed visualization of a complex coronary-venous fistula with additional feeders from the descending aorta. JACC Case Rep, 2(11):1736–1738. https://doi.org/10.1016/j.jaccas.2020.06.028
8. Santoro G, Pizzuto A, Rizza A, et al., 2021, Transcatheter treatment of ‘complex’ aortic coarctation guided by printed 3D model. JACC Case Rep, 3(6):900–904. https://doi.org/10.1016/j.jaccas.2021.04.036
9. Kim WK, Kim T, Lee S, et al., 2019, 3D-printing-based open repair of extensive thoracoabdominal aorta in severe scoliosis. Semin Thorac Cardiovasc Surg, 31(1):61–63. https://doi.org/10.1053/j.semtcvs.2018.09.017
10. Ooms JF, Wang DD, Rajani R, et al., 2021, Computed tomography–derived 3D modeling to guide sizing and planning of transcatheter mitral valve interventions. JACC Cardiovasc Imaging, 14(8): 1644–1658. https://doi.org/10.1016/j.jcmg.2020.12.034
11. Sherifova S, Holzapfel GA, 2019, Biomechanics of aortic wall failure with a focus on dissection and aneurysm: A review. Acta Biomater, 99:1–17. https://doi.org/10.1016/j.actbio.2019.08.017
12. Lin S, Morgant MC, Marín-Castrillón DM, et al., 2022, Aortic local biomechanical properties in ascending aortic aneurysms. Acta Biomater, 149:40–50 . https://doi.org/10.1016/j.actbio.2022.06.019
13. Community challenge towards consensus on characterization of biological tissue. C4Bio. https://c4bio.eu/ (accessed Dec. 10, 2022).
14. Caballero AD, Laín S, 2013, A review on computational fluid dynamics modelling in human thoracic aorta. Cardiovasc Eng Technol, 4(2):103–130. https://doi.org/10.1007/s13239-013-0146-6
15. Ong CW, Wee I, Syn N, et al., 2020, Computational fluid dynamics modeling of hemodynamic parameters in the human diseased aorta: A systematic review. Ann Vasc Surg, 63:336–381. https://doi.org/10.1016/j.avsg.2019.04.032
16. Mourato A, Valente R, Xavier J, et al., 2022, Computational modelling and simulation of fluid structure interaction in aortic aneurysms: A systematic review and discussion of the clinical potential. Appl Sci, 12(16):8049. https://doi.org/10.3390/app12168049
17. Vignali E, Gasparotti E, Celi S, et al., 2021, Fully-coupled FSI computational analyses in the ascending thoracic aorta using patient-specific conditions and anisotropic material properties. Front Physiol, 12:732561. https://doi.org/10.3389/fphys.2021.732561
18. Simão M, Ferreira J, Tomás AC, et al., 2017, Aorta ascending aneurysm analysis using CFD models towards possible anomalies. Fluids, 2(2):31. https://doi.org/10.3390/fluids2020031
19. Wee I, Ong CW, Syn N, et al., 2018, Computational fluid dynamics and aortic dissections: Panacea or panic? Vasc Endovasc Rev, 1(1):27–29. https://doi.org/10.15420/ver.2018.8.2
20. Wang Y, Joannic D, Juillion P, et al., 2016, Comparison of flow measurement by 4D flow magnetic resonance imaging and by particles image velocimetry on phantom of abdominal aortic aneurysm. SM Vasc Med, 1(2):1008. https://hal.archives-ouvertes.fr/hal-01463873
21. Kurenov SN, Ionita C, Sammons D, et al., 2015, Three-dimensional printing to facilitate anatomic study, device development, simulation, and planning in thoracic surgery. J Thorac Cardiovasc Surg, 149(4):973–979. https://doi.org/10.1016/j.jtcvs.2014.12.059
22. Vukicevic M, Puperi DS, Grande-Allen KJ, et al., 2016, Erratum to: 3D printed modeling of the mitral valve for catheter-based structural interventions. Ann Biomed Eng, 44(11):3432–3432. https://doi.org/10.1007/s10439-016-1690-7
23. Wang K, Wu C, Qian Z, et al., 2016. Dual-material 3D printed metamaterials with tunable mechanical properties for patient-specific tissue-mimicking phantoms. Addit Manuf, 12:31–37. https://doi.org/10.1016/j.addma.2016.06.006
24. Biglino G, Verschueren P, Zegels R, et al., 2013, Rapid prototyping compliant arterial phantoms for in-vitro studies and device testing. J Cardiovasc Magn Reson, 15(1):2. https://doi.org/10.1186/1532-429X-15-2
25. Duprey A, Khanafer K, Schlicht M, et al., 2010, In vitro characterisation of physiological and maximum elastic modulus of ascending thoracic aortic aneurysms using uniaxial tensile testing. Eur J Vasc Endovasc Surg, 39(6):700–707. https://doi.org/10.1016/j.ejvs.2010.02.015
26. Chung M, Radacsi N, Robert C, et al., 2018, On the optimization of low-cost FDM 3D printers for accurate replication of patient-specific abdominal aortic aneurysm geometry. 3D Print Med, 4(1):2. https://doi.org/10.1186/s41205-017-0023-2
27. Iliopoulos DC, Deveja RP, Kritharis EP, et al., 2009, Regional and directional variations in the mechanical properties of ascending thoracic aortic aneurysms. Med Eng Phys, 31(1):1–9. https://doi.org/10.1016/j.medengphy.2008.03.002
28. Ferrara A, Morganti S, Totaro P, et al., 2016, Human dilated ascending aorta: Mechanical characterization via uniaxial tensile tests. J Mech Behav Biomed Mater, 53:257–271. https://doi.org/10.1016/j.jmbbm.2015.08.021
29. Ferrara A, Totaro P, Morganti S, et al., 2018, Effects of clinico-pathological risk factors on in-vitro mechanical properties of human dilated ascending aorta. J Mech Behav Biomed Mater, 77:1–11. https://doi.org/10.1016/j.jmbbm.2017.08.032
30. Vande Geest JP, Sacks MS, Vorp DA, 2006, The effects of aneurysm on the biaxial mechanical behavior of human abdominal aorta. J Biomech, 39(7):1324–1334. https://doi.org/10.1016/j.jbiomech.2005.03.003
31. Azadani AN, Chitsaz S, Mannion A, et al., 2013, Biomechanical properties of human ascending thoracic aortic aneurysms. Ann Thorac Surg, 96(1):50–58. https://doi.org/10.1016/j.athoracsur.2013.03.094
32. Pham T, Martin C, Elefteriades J, et al., 2013, Biomechanical characterization of ascending aortic aneurysm with concomitant bicuspid aortic valve and bovine aortic arch. Acta Biomater, 9(8):7927–7936. https://doi.org/10.1016/j.actbio.2013.04.021
33. Raghavan ML, Webster MW, Vorp DA, 1996, Ex vivo biomechanical behavior of abdominal aortic aneurysm: Assessment using a new mathematical model. Ann Biomed Eng, 24(5):573–582. https://doi.org/10.1007/BF02684226
34. Vorp DA, Schiro BJ, Ehrlich MP, et al., 2003, Effect of aneurysm on the tensile strength and biomechanical behavior of the ascending thoracic aorta. Ann Thorac Surg, 75(4):1210–1214. https://doi.org/10.1016/S0003-4975(02)04711-2
35. Di Martino ES, Bohra A, Vande Geest JP, et al., 2006, Biomechanical properties of ruptured versus electively repaired abdominal aortic aneurysm wall tissue. J Vasc Surg, 43(3):570–576. https://doi.org/10.1016/j.jvs.2005.10.072
36. Sommer G, Sherifova S, Oberwalder PJ, et al., 2016, Mechanical strength of aneurysmatic and dissected human thoracic aortas at different shear loading modes. J Biomech, 49(12):2374–2382. https://doi.org/10.1016/j.jbiomech.2016.02.042
37. García-Herrera CM, Atienza JM, Rojo FJ, et al., 2012, Mechanical behaviour and rupture of normal and pathological human ascending aortic wall. Med Biol Eng Comput, 50(6):559–566. https://doi.org/10.1007/s11517-012-0876-x