3D-Printed multi-material liver model with simultaneous mechanical and radiological tissue-mimicking features for improved realism
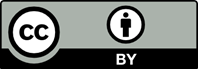
Anatomic models have an important role in the medical domain. However, soft tissue mechanical properties’ representation is limited in mass-produced and 3D-printed models. In this study, a multi-material 3D printer was used to print a human liver model featuring tuned mechanical and radiological properties, with the goal of comparing the printed model with its printing material and real liver tissue. The main target was mechanical realism, while radiological similarity was a secondary objective. Materials and internal structure were selected such that the printed model would resemble liver tissue in terms of tensile properties. The model was printed at 33% scaling and 40% gyroid infill with a soft silicone rubber, and silicone oil as a filler fluid. After printing, the liver model underwent CT scanning. Since the shape of the liver is incompatible with tensile testing, tensile testing specimens were also printed. Three replicates were printed with the same internal structure as the liver model and three more out of silicone rubber with 100% rectilinear infill to allow a comparison. All specimens were tested in a four-step cyclic loading test protocol to compare elastic moduli and dissipated energy ratios. The fluid-filled and full-silicone specimens had initial elastic moduli of 0.26 MPa and 0.37 MPa, respectively, and featured dissipated energy ratios of 0.140, 0.167, 0.183, and 0.118, 0.093, 0.081, respectively, in the second, third, and fourth loading cycles. The liver model showed 225 ± 30 Hounsfield units (HU) in CT, which is closer to real human liver (70 ± 30 HU) than the printing silicone (340 ± 50 HU). Results suggest that the liver model became more realistic in terms of mechanical and radiological properties with the proposed printing approach as opposed to printing only with silicone rubber. Thus, it has been demonstrated that this printing method enables new customization opportunities in the field of anatomic models.
1. Preece D, Williams SB, Lam R, et al., 2013, Let’s get physical: Advantages of a physical model over 3D computer models and textbooks in learning imaging anatomy. Anat Sci Educ, 6(4):216–224. https://doi.org/10.1002/ase.1345
2. Khot Z, Quinlan K, Norman GR, et al., 2013, The relative effectiveness of computer-based and traditional resources for education in anatomy. Anat Sci Educ, 6(4): 211–215. https://doi.org/10.1002/ase.1355
3. Wang K, Ho CC, Zhang C, et al., 2017, A review on the 3D printing of functional structures for medical phantoms and regenerated tissue and organ applications. Engineering, 3(5):653–662. https://doi.org/10.1016/J.ENG.2017.05.013
4. Pugliese L, Marconi S, Negrello E, et al., 2018, The clinical use of 3D printing in surgery. Updates Surg, 70(3): 381–388. https://doi.org/10.1007/s13304-018-0586-5
5. Tack P, Victor J, Gemmel P, et al., 2016, 3D printing techniques in a medical setting: A systematic literature review. Biomed Eng Online, 15(115). https://doi.org/10.1186/s12938-016-0236-4
6. Mohan P, Marzougui D, Kan CD, 2009, Development and validation of hybrid III crash test dummy. SAE Technical Paper. https://doi.org/10.4271/2009-01-0473
7. Humphrey C, Kumaratilake J, 2016, Ballistics and anatomical modelling—A review. Leg Med, 23(12):21–29. https://doi.org/10.1016/j.legalmed.2016.09.002
8. Trehan K, Kemp CD, Yang SC, 2014, Simulation in cardiothoracic surgical training: Where do we stand? JTCS, 147(1):18–24. https://doi.org/10.1016/j.jtcvs.2013.09.007
9. Kurt E, Yurdakul SE, Atac A, 2013, An overview of the technologies used for anatomy education in terms of medical history. Proc Soc, 103:109–115. https://doi.org/10.1016/j.sbspro.2013.10.314
10. Cooper JB, Taqueti VR, 2004, A brief history of the development of mannequin simulators for clinical education and training. Qual Saf Health Care, 13:563–570. https://doi.org/10.1136/qshc.2004.009886
11. Ngo TD, Kashani A, Imbalzano G, et al., 2018, Additive manufacturing (3D printing): A review of materials, methods, applications and challenges. Compos B Eng, 143: 172-196:172–196. https://doi.org/10.1016/j.compositesb.2018.02.012
12. Nikitichev DI, Patel P, Avery J, et al., 2018, Chapter 6: Patient-specific 3D printed models for education, research and surgical simulation, in 3D Printing, IntechOpen Limited, London, United Kingdom. https://doi.org/10.5772/intechopen.79667
13. Waran V, Narayanan V, Karuppiah R, et al., 2014, Injecting realism in surgical training-initial simulation experience with custom 3D models, J Surg Educ, 71(2):193–197. https://doi.org/10.1016/j.jsurg.2013.08.010
14. Wang K, Wu C, Qian Z, et al., 2016, Dual-material 3D printed metamaterials with tunable mechanical properties for patient-specific tissue-mimicking phantoms. Addit Manuf, 12:31–37. https://doi.org/10.1016/j.addma.2016.06.006
15. Yeo J, Koh J, Wang F, et al., (eds.), 2020, Chapter 9: 3D printing silicone materials and devices, in Silicon Containing Hybrid Copolymers, John Wiley & Sons Inc. https://doi.org/10.1002/9783527823499.ch9
16. Spectroplast AG, https://spectroplast.com/, viewed 04.08.2022
17. Stratasys Ltd, https://stratasys.com/medical/advanced-medical-models, viewed 04.08.2022
18. Deltatower GmbH, https://deltatower.ch/en/home-2/, viewed 04.08.2022
19. CR-3D, www.cr3d.de/3d-drucker/liquid-serie/, viewed 02.11.2022
20. Lynxter SAS, lynxter.fr/en/product/3d-printing-silicone-toolhead-liq21/, viewed 02.11.2022
21. Qiu K, Haghiashtiani G, McAlpine MC, 2018, 3D printed organ models for surgical applications. Annu Rev Anal Chem, 11(1):287–306. https://doi.org/10.1146/annurev-anchem-061417-125935
22. Estermann SJ, Pahr DH, Reisinger A, 2020, Quantifying tactile properties of liver tissue, silicone elastomers, and a 3D printed polymer for manufacturing realistic organ models. Mech Behav Biomed Mater, 104:103630. https://doi.org/10.1016/j.jmbbm.2020.103630
23. Witowski JS, Coles-Black J, Zuzak TZ, et al., 2017, 3D Printing in liver surgery: A systematic review. Telemed e-Health, 23(12):943–947. https://doi.org/10.1089/tmj.2017.0049
24. Ratinam R, Quayle M, Crock J, et al., 2019, Challenges in creating dissectible anatomical 3D prints for surgical teaching. J Anat, 234(4):419–437. https://doi.org/10.1111/joa.12934
25. Truby RL, Lewis JA, 2016, Printing soft matter in three dimensions. Nature, 540(7633):371–378. https://doi.org/10.1038/nature21003
26. Ionita CN, Mokin M, Varble N, et al., 2014, Challenges and limitations of patient-specific vascular phantom fabrication using 3D Polyjet printing. Proc SPIE Int Soc Opt Eng, 9038:90380M. https://doi.org/10.1117/12.2042266
27. Maddox MM, Feibus A, Liu J, et al., 2018, 3D-printed soft-tissue physical models of renal malignancies for individualized surgical simulation: A feasibility study. J Robot Surg, 12(1):27–33. https://doi.org/10.1007/s11701-017-0680-6
28. Saari M, Xia B, Krueger PS, et al., 2015, Additive manufacturing of soft and composite parts from thermoplastic elastomers. Proceedings of the International Solid Freeform Fabrication Symposium, Austin, TX.
29. Bakarich SE, Gorkin R, Panhuis M, et al., 2014, Three-dimensional printing fiber reinforced hydrogel composites. ACS App Mater Interfaces, 6(18):15998–16006. https://doi.org/10.1021/am503878d
30. Hardin JO, Ober TJ, Valentine AD, et al., 2015, Microfluidic printheads for multimaterial 3D printing of viscoelastic inks. Adv Mater, 27(21):3279–3284. https://doi.org/10.1002/adma.201500222
31. Jung JW, Lee JS, Cho DW, 2016, Computer-aided multiple-head 3D printing system for printing of heterogeneous organ/tissue constructs. Sci Rep, 6:21685. https://doi.org/10.1038/srep21685
32. Liu W, Zhang YS, Heinrich MA, et al., 2016, Rapid continuous multimaterial extrusion bioprinting. Adv Mater, 29(3):1604630. https://doi.org/10.1002/adma.201604630
33. Skylar-Scott MA, Mueller J, Visser CW, et al., 2019, Voxelated soft material via multimaterial multinozzle 3D printing. Nature, 575(7782):330–335. https://doi.org/10.1038/s41586-019-1736-8
34. Talalwa L, Natour G, Bauer A, et al., 2020, Radiological characteristics of a new experimental rubber elastomeric polymer used in three-dimensional printing with different infill densities and patterns. J Phys Commun, 4(12): 125006. https://doi.org/10.1088/2399-6528/abd1c3
35. Estermann SJ, Förster-Streffleur S, Hirtler L, et al., 2021, Comparison of thiel preserved, fresh human, and animal liver tissue in terms of mechanical properties. Ann Anat, 236:151717. https://doi.org/10.1016/j.aanat.2021.151717
36. Estermann SJ, Pahr DH, Reisinger A, 2020, Hyperelastic and viscoelastic characterization of hepatic tissue under uniaxial tension in time and frequency domain. Mech Behav Biomed Mater, 112:104038. https://doi.org/10.1016/j.jmbbm.2020.104038
37. Jaksa L, Pahr DH, Kronreif G, et al., 2021, Development of a multi-material 3D printer for functional anatomic models. Int J Bioprint, 7(4). https://doi.org/10.18063/ijb.v7i4.420
38. Jaksa L, Pahr DH, Kronreif G, et al., 2022, Calibration dependencies and accuracy assessment of a silicone rubber 3D printer. Inventions, 7(35). https://doi.org/10.3390/inventions7020035
39. 3D-Slicer, https://www.slicer.org/, viewed 04.08.2022
40. Autodesk Meshmixer, https://www.meshmixer.com/, viewed 04.08.2022
41. Elkem AMSil Silicones, https://www.elkem.com/silicones/brands/amsil/, viewed 04.08.2022
42. Optimal Products GmbH, https://optimal-products.de/silikonoele/, viewed 04.08.2022
43. Smooth-On Silc Pig Paints, https://www.smooth-on.com/products/silc-pig/, viewed 04.08.2022
44. Prusa Research a.s., https://www.prusa3d.com/page/prusaslicer_424/, viewed 04.08.2022
45. ASTM D638-14, viewed August 04, 2022, https://www.astm. org/d0638-14.html https://www.astm.org/d0638-14.html, viewed 04.08.2022
46. Aryeetey OJ, Frank M, Lorenz A, et al., 2021, A parameter reduced adaptive quasi-linear viscoelastic model for soft biological tissue in uniaxial tension. Mech Behav Biomed Mater, 126:104999. https://doi.org/10.1016/j.jmbbm.2021.104999
47. Frank M, Marx D, Nedelkovski V, et al., 2018, Dehydration of individual bovine trabeculae causes transition from ductile to quasi brittle failure mode. Mech Behav Biomed Mater, 87:296–305. https://doi.org/10.1016/j.jmbbm.2018.07.039
48. Aryeetey OJ, Frank M, Lorenz A, et al., 2022, Fracture toughness determination of porcine muscle tissue based on AQLV model derived viscous dissipated energy. Mech Behav Biomed Mater, 153:105429. https://doi.org/10.1016/j.jmbbm.2022.105429
49. Goh GD, Sing SL, Lim YF, et al., 2021, Machine learning for 3D printed multi-materials tissue-mimicking anatomical models. Mater Des, 211:110125. https://doi.org/10.1016/j.matdes.2021.110125
50. Kwon J, Ock J, Kim N, 2020, Mimicking the mechanical properties of aortic tissue with pattern-embedded 3D printing for a realistic phantom. Materials, 13(21): 5042. https://doi.org/10.3390/ma13215042
51. Rietzel E, Schardt D, Haberer T, 2007, Range accuracy in carbon ion treatment planning based on CT-calibration with real tissue samples. Radiat Oncol, 2(14). https://doi.org/10.1186/1748-717X-2-14
52. Toledo JM, Ribeiro TPC, 2009, Radiological evaluation of a liver simulator in comparison to a human real liver. Proceedings of the International Nuclear Atlantic Conference – INAC 2009. ISBN: 978-85-99141-03-8
53. Jaksa L, Hatamikia S, Pahr DH, et al., 2022, 3D-printer enabling customized anatomic models, Poster at the 27th Congress of the European Society of Biomechanics, Porto, Portugal. https://doi.org/10.13140/RG.2.2.28812.39049