3D bioprinting of ultrashort self-assembling peptides to engineer scaffolds with different matrix stiffness for chondrogenesis
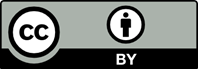
Articular cartilage is a nonvascularized and poorly cellularized tissue with a low selfrepair capacity. Therefore, damage to this tissue due to trauma or degenerative joint diseases such as osteoarthritis needs a high-end medical intervention. However, such interventions are costly, have limited healing capacity, and could impair patients’ quality of life. In this regard, tissue engineering and three-dimensional (3D) bioprinting hold great potential. However, identifying suitable bioinks that are biocompatible, with the desired mechanical stiffness, and can be used under physiological conditions is still a challenge. In this study, we developed two tetrameric self-assembling ultrashort peptide bioinks that are chemically well-defined and can spontaneously form nanofibrous hydrogels under physiological conditions. The printability of the two ultrashort peptides was demonstrated; different shape constructs were printed with high shape fidelity and stability. Furthermore, the developed ultrashort peptide bioinks gave rise to constructs with different mechanical properties that could be used to guide stem cell differentiation toward specific lineages. Both ultrashort peptide bioinks demonstrated high biocompatibility and supported the chondrogenic differentiation of human mesenchymal stem cells. Additionally, the gene expression analysis of differentiated stem cells with the ultrashort peptide bioinks revealed articular cartilage extracellular matrix formation preference. Based on the different mechanical stiffness of the two ultrashort peptide bioinks, they can be used to fabricate cartilage tissue with different cartilaginous zones, including the articular and calcified cartilage zones, which are essential for engineered tissue integration.
1. Seliktar D, 2012, Designing cell-compatible hydrogels for biomedical applications. Science, 336(6085):1124–1128.
2. Fang Y, Eglen RM, 2017, Three-dimensional cell cultures in drug discovery and development. SLAS Discov, 22(5):456–472.
3. Zhao W, Jin X, Cong Y, et al., 2013, Degradable natural polymer hydrogels for articular cartilage tissue engineering. J Chem Technol Biotechnol, 88(3):327–339.
4. Lu B, Yuk H, Lin S, et al., 2019, Pure pedot: Pss hydrogels. Nat Commun, 10(1):1043.
5. Yang J, Bai R, Suo Z, 2018, Topological adhesion of wet materials. Adv Mater, 30(25):1800671.
6. Seow WY, Hauser CA, 2014, Short to ultrashort peptide hydrogels for biomedical uses. Mater Today, 17(8):381–388.
7. Richardson SM, Kalamegam G, Pushparaj PN, et al., 2016, Mesenchymal stem cells in regenerative medicine: Focus on articular cartilage and intervertebral disc regeneration. Methods, 99:69–80.
8. Somoza RA, Welter JF, Correa D, et al., 2014, Chondrogenic differentiation of mesenchymal stem cells: Challenges and unfulfilled expectations. Tissue Eng Part B: Rev, 20(6):596–608.
9. Ng WL, Chua CK, Shen Y-F, 2019, Print me an organ! Why we are not there yet. Progr Polym Sci, 97:101145.
10. Huang J, Xiong J, Wang D, et al., 2021, 3D bioprinting of hydrogels for cartilage tissue engineering. Gels, 7(3):144.
11. Han X, Chang S, Zhang M, 2021, Advances of hydrogel-based bioprinting for cartilage tissue engineering. Front Bioeng Biotechnol, (9):816–829.
12. Huang J, Huang Z, Liang Y, et al., 2021, 3D printed gelatin/ hydroxyapatite scaffolds for stem cell chondrogenic differentiation and articular cartilage repair. Biomater Sci, 9(7):2620–2630.
13. Xiongfa J, Hao Z, Liming Z, et al., 2018, Recent advances in 3D bioprinting for the regeneration of functional cartilage. Regen Med, 13(1):73–87.
14. Ng WL, Lee JM, Zhou M, et al., 2020, Vat polymerization-based bioprinting—Process, materials, applications and regulatory challenges. Biofabrication, 12(2):022001.
15. Jiang T, Munguia-Lopez JG, Flores-Torres S, et al., 2019, Extrusion bioprinting of soft materials: An emerging technique for biological model fabrication. Appl Phys Rev, 6(1):011310.
16. Li X, Liu B, Pei B, et al., 2020, Inkjet bioprinting of biomaterials. Chem Rev, 120(19):10793–10833.
17. Tekin E, Smith PJ, Schubert US, 2008, Inkjet printing as a deposition and patterning tool for polymers and inorganic particles. Soft Matter, 4(4):703–713.
18. Villar G, Graham AD, Bayley H, 2013, A tissue-like printed material. Science, 340(6128):48–52.
19. Xu T, Jin J, Gregory C, et al., 2005, Inkjet printing of viable mammalian cells. Biomaterials, 26(1):93–99.
20. Ng WL, Huang X, Shkolnikov V, et al., 2022, Controlling droplet impact velocity and droplet volume: Key factors to achieving high cell viability in sub-nanoliter droplet-based bioprinting. Int J Bioprint, 8(1): 424–440.
21. Zhang LG, Leong K, Fisher JP, 2022, 3D Bioprinting and Nanotechnology in Tissue Engineering and Regenerative Medicine, Academic Press.
22. Xue K, Zhang X, Gao Z, et al., 2019, Cartilage progenitor cells combined with PHBV in cartilage tissue engineering. J Transl Med, 17(1):1–11.
23. Weissenberger M, Weissenberger MH, Wagenbrenner M, et al., 2020, Different types of cartilage neotissue fabricated from collagen hydrogels and mesenchymal stromal cells via SOX9, TGFB1 or BMP2 gene transfer. PLoS One, 15(8):e0237479.
24. Ibrahim MI, Alsafadi D, Alamry KA, et al., 2021, Properties and applications of poly(3-hydroxybutyrate-co-3-hydroxyvalerate) biocomposites. J Polym Environ, 29:1010–1030.
25. Montalbano G, Borciani G, Pontremoli C, et al., 2019, Development and biocompatibility of collagen-based composites enriched with nanoparticles of strontium containing mesoporous glass. Materials, 12(22):3719.
26. Khan Z, Kahin K, Rauf S, et al., 2019, Optimization of a 3D bioprinting process using ultrashort peptide bioinks. Int J Bioprint, 5(1):173.
27. Di Bella C, Fosang A, Donati DM, et al., 2015, 3D bioprinting of cartilage for orthopedic surgeons: Reading between the lines. Front Surg, 2:39.
28. Wei W, Ma Y, Yao X, et al., 2021, Advanced hydrogels for the repair of cartilage defects and regeneration. Bioact Mater, 6(4):998–1011.
29. Susapto HH, Alhattab D, Abdelrahman S, et al., 2021, Ultrashort peptide bioinks support automated printing of large-scale constructs assuring long-term survival of printed tissue constructs. Nano Lett, 21(7):2719–2729.
30. Kahin K, Khan Z, Albagami M, et al., 2019, Development of a robotic 3D bioprinting and microfluidic pumping system for tissue and organ engineering. Microfluidics, BioMEMS, and Medical Microsystems XVII, SPIE, 130–139.
31. Alhattab D, Jamali F, Ali D, et al., 2019, An insight into the whole transcriptome profile of four tissue-specific human mesenchymal stem cells. Regen Med, 14(9):841–865.
32. Rajbhandary A, Nilsson BL, 2016, Self-assembling hydrogels, In Gels Handbook: Fundamentals, Properties and Application; Volume 1: Fundamentals of Hydrogels, New Jersey, 219–250.
33. Wang X, Horii A, Zhang S, 2008, Designer functionalized self-assembling peptide nanofiber scaffolds for growth, migration, and tubulogenesis of human umbilical vein endothelial cells. Soft Matter, 4(12):2388–2395.
34. Memic A, Alhadrami HA, Hussain MA, et al., 2016, Hydrogels 2.0: Improved properties with nanomaterial composites for biomedical applications. Biomed Mater, 11(1):014104.
35. Huang L-C, Wang H-C, Chen L-H, et al., 2019, Bioinspired self-assembling peptide hydrogel with proteoglycan-assisted growth factor delivery for therapeutic angiogenesis. Theranostics, 9(23):7072–7087.
36. Unagolla JM, Jayasuriya AC, 2020, Hydrogel-based 3D bioprinting: A comprehensive review on cell-laden hydrogels, bioink formulations, and future perspectives. Appl Mater Today, 18:100479.
37. Susapto HH, Alhattab D, Abdelrahman S, et al., 2021, Ultrashort peptide bioinks support automated printing of large-scale constructs assuring long-term survival of printed tissue constructs. Nano Lett, 21(7):2719–2729.
38. Waters ML, Aromatic interactions in peptides: Impact on structure and function. Pept Sci, 76(5):435–445.
39. Greenfield MA, Hoffman JR, de la Cruz MO, et al., 2010, Tunable mechanics of peptide nanofiber gels. Langmuir, 26(5):3641–3647.
40. Loo Y, Lakshmanan A, Ni M, et al., 2015, Peptide bioink: Self-assembling nanofibrous scaffolds for three-dimensional organotypic cultures. Nano Lett, 15(10):6919–6925.
41. Wu L, Magaz A, Wang T, et al., 2018, Stiffness memory of indirectly 3D-printed elastomer nanohybrid regulates chondrogenesis and osteogenesis of human mesenchymal stem cells. Biomaterials, 186:64–79.
42. El-Rashidy AA, El Moshy S, Radwan IA, et al., 2021, Effect of polymeric matrix stiffness on osteogenic differentiation of mesenchymal stem/progenitor cells: Concise review. Polymers, 13(17):2950.
43. Fahy N, Alini M, Stoddart MJ, 2018, Mechanical stimulation of mesenchymal stem cells: Implications for cartilage tissue engineering. J Orthop Res, 36(1):52–63.
44. Hao J, Zhang Y, Jing D, et al., 2015, Mechanobiology of mesenchymal stem cells: Perspective into mechanical induction of MSC fate. Acta Biomater, 20:1–9.
45. Almalki SG, Agrawal DK, 2016, Key transcription factors in the differentiation of mesenchymal stem cells. Differentiation, 92(1–2):41–51.
46. Liu Y, Shah KM, Luo J, 2021, Strategies for articular cartilage repair and regeneration. Front Bioeng Biotechnol, 9:1328.
47. Idaszek J, Costantini M, Karlsen TA, et al., 2019, 3D bioprinting of hydrogel constructs with cell and material gradients for the regeneration of full-thickness chondral defect using a microfluidic printing head. Biofabrication, 11(4):044101.
48. Costantini M, Idaszek J, Szöke K, et al., 2016, 3D bioprinting of BM-MSCs-loaded ECM biomimetic hydrogels for in vitro neocartilage formation. Biofabrication, 8(3):035002.
49. Roughley PJ, Mort JS, 2014, The role of aggrecan in normal and osteoarthritic cartilage. J Exp Orthop, 1(1):1–11.
50. Hollenstein J, Terrier A, Cory E, et al., 2015, Mechanical evaluation of a tissue-engineered zone of calcification in a bone–hydrogel osteochondral construct. Comput Methods Biomech Biomed Eng, 18(3):332–337.
51. Olivares-Navarrete R, Lee EM, Smith K, et al., Substrate stiffness controls osteoblastic and chondrocytic differentiation of mesenchymal stem cells without exogenous stimuli. PLoS One, 12(1):e0170312.
52. Zhou Y, Qiu J, Wan L, et al., 2022, The effect of matrix stiffness on the chondrogenic differentiation of mesenchymal stem cells. J Mol Histol, 53(5):805–816.
53. Mohammed M, Lai T-S, Lin H-C, 2021, Substrate stiffness and sequence dependent bioactive peptide hydrogels influence the chondrogenic differentiation of human mesenchymal stem cells. J Mater Chem B, 9(6):1676–1685.
54. Park JS, Chu JS, Tsou AD, et al., 2011, The effect of matrix stiffness on the differentiation of mesenchymal stem cells in response to TGF-β. Biomaterials, 32(16):3921–3930.