Bioprinted Notch ligand to function as stem cell niche improves muscle regeneration in dystrophic muscle
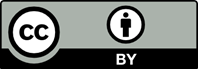
In Duchenne muscular dystrophy, dystrophic muscle phenotypes are closely associated with the exhaustion of muscle stem cells. Transplantation of muscle stem cells has been widely studied for improving muscle regeneration, but poor cell survival and self-renewal, rapid loss of stemness, and limited dispersion of grafted cells following transplantation have collectively hindered the overall success of this strategy. Optimized mechanisms for maintaining and improving stem cell function are naturally present in the microenvironment of the stem cell niche in healthy muscles. Therefore, one logical strategy toward improving stem cell function and efficiency of stem cell transplantation in diseased muscles would be the establishment of a microenvironment mimicking some key aspects of healthy native stem cell niches. Here, we applied inkjet-based bioprinting technology to engineer a mimicked artificial stem cell niche in dystrophic muscle, comprising stem cell niche regulating factors (Notch activator DLL1) bioprinted onto 3D DermaMatrix construct. The recombinant DLL1 protein, DLL1 (mouse): Fc (human) (rec), was applied here as the Notch activator. Bioprinted DermaMatrix construct was seeded with muscle stem cells in vitro, and increased stem cell maintenance and repressed myogenic differentiation process was observed. DLL1 bioprinted DermaMatrix construct was then engrafted into dystrophic muscle of mdx/scid mice, and the improved cell engraftment and progression of muscle regeneration was observed 10 days after engraftment. Our results demonstrated that bioprinting of Notch activator within 3D construct can be applied to serve as muscle stem cell niche and improve the efficacy of muscle stem cell transplantation in diseased muscle.
1.Sacco A, Mourkioti F, Tran R, et al., 2010, Short telomeres and stem cell exhaustion model Duchenne muscular dystrophy in mdx/mTR mice. Cell, 143(7):1059–1071.https://doi.org/10.1016/j.cell.2010.11.039
2. Jang YC, Sinha M, Cerletti M, et al., 2011, Skeletal muscle stem cells: Effects of aging and metabolism on muscle regenerative function. Cold Spring Harb Symp Quant Biol,76:101–111.https://doi.org/10.1101/sqb.2011.76.010652
3. Usas A, Maciulaitis J, Maciulaitis R, et al., 2011, Skeletal muscle-derived stem cells: Implications for cell-mediated therapies. Medicina (Kaunas), 47(9):469–479.
4. Ikezawa M, Cao B, Qu Z, et al., 2003, Dystrophin delivery in dystrophin-deficient DMDmdx skeletal muscle by isogenic muscle-derived stem cell transplantation. Hum Gene Ther,14(16):1535–1546.https://doi.org/10.1089/104303403322495043
5. Winkler T, von Roth P, Matziolis G, et al., 2009, Dose-response relationship of mesenchymal stem cell transplantation and functional regeneration after severe skeletal muscle injury in rats. Tissue Eng Part A, 15(3):487–492.https://doi.org/10.1089/ten.tea.2007.0426
6. Tremblay JP, Malouin F, Roy R, et al., 1993, Results of a triple blind clinical study of myoblast transplantations without immunosuppressive treatment in young boys with Duchenne muscular dystrophy. Cell Transplant, 2(2):99–112.
7. Drowley L, Okada M, Beckman S, et al., 2010, Cellular antioxidant levels influence muscle stem cell therapy. Mol Ther, 18(10):1865–1873.
https://doi.org/10.1038/mt.2010.160
8. Maclean S, Khan WS, Malik AA, et al., 2012, The potential of stem cells in the treatment of skeletal muscle injury and disease. Stem Cells Int, 2012:282348.https://doi.org/10.1155/2012/282348
9. Gussoni E, Blau HM, Kunkel LM, 1997, The fate of individual myoblasts after transplantation into muscles of DMD patients. Nat Med, 3(9):970–977.
10. Karpati G, Holland P, Worton RG, 1992, Myoblast transfer in DMD: Problems in the interpretation of efficiency. Muscle Nerve, 15(10):1209–1210.https://doi.org/10.1002/mus.880151016
11. Mayeuf A, Relaix F, 2011, Notch pathway: From development to regeneration of skeletal muscle. Med Sci (Paris), 27(5):521–526.
https://doi.org/10.1051/medsci/2011275018
12. Carey KA, Farnfield MM, Tarquinio SD, et al., 2007, Impaired expression of Notch signaling genes in aged human skeletal muscle. J Gerontol A Biol Sci Med Sci, 62(1):9–17.https://doi.org/62/1/9
13. Conboy IM, Rando TA, 2002, The regulation of Notch signaling controls satellite cell activation and cell fate determination in postnatal myogenesis. Dev Cell, 3(3):397–409.
14. Acharyya S, Sharma SM, Cheng AS, et al., 2010, TNF inhibits Notch-1 in skeletal muscle cells by Ezh2 and DNA methylation mediated repression: Implications in Duchenne muscular dystrophy. PLoS One, 5(8):e12479.https://doi.org/10.1371/journal.pone.0012479
15. Sweeney C, Morrow D, Birney YA, et al., 2004, Notch 1 and 3 receptor signaling modulates vascular smooth muscle cell growth, apoptosis, and migration via a CBF-1/RBP-Jk dependent pathway. FASEB J, 18(12):1421–1423. https://doi.org/10.1096/fj.04-1700fje04-1700fje
16. Li Y, Hiroi Y, Liao JK, 2010, Notch signaling as an important mediator of cardiac repair and regeneration after myocardial infarction. Trends Cardiovasc Med, 20(7):228–231. https://doi.org/10.1016/j.tcm.2011.11.006
17. Carlson ME, Hsu M, Conboy IM, 2008, Imbalance between pSmad3 and Notch induces CDK inhibitors in old muscle stem cells. Nature, 454(7203):528–532.https://doi.org/10.1038/nature07034
18. Yang K, Proweller A, 2011, Vascular smooth muscle Notch signals regulate endothelial cell sensitivity to angiogenic stimulation. J Biol Chem, 286(15):13741–13753.https://doi.org/10.1074/jbc.M110.181842
19. Phng LK, Gerhardt H, 2009, Angiogenesis: A team effort coordinated by notch. Dev Cell, 16(2):196–208.
https://doi.org/10.1016/j.devcel.2009.01.015
20. Boonen KJ, Post MJ, 2008, The muscle stem cell niche: Regulation of satellite cells during regeneration. Tissue Eng Part B Rev, 14(4):419–431.
https://doi.org/10.1089/ten.teb.2008.0045
21. Kuang S, Kuroda K, Le Grand F, et al., 2007, Asymmetric self-renewal and commitment of satellite stem cells in muscle. Cell, 129(5):999–1010.
https://doi.org/10.1016/j.cell.2007.03.044
22. Chakkalakal JV, Jones KM, Basson MA, et al., 2012, The aged niche disrupts muscle stem cell quiescence. Nature,490(7420):355–360.
https://doi.org/10.1038/nature11438
23. Bjornson CR, Cheung TH, Liu L, et al., 2012, Notch signaling is necessary to maintain quiescence in adult muscle stem cells. Stem Cells, 30(2):232–242.https://doi.org/10.1002/stem.773
24. Mourikis P, Sambasivan R, Castel D, et al., 2012, A critical requirement for notch signaling in maintenance of the quiescent skeletal muscle stem cell state. Stem Cells,30(2):243–252.https://doi.org/10.1002/stem.775
25. Fukada S, Yamaguchi M, Kokubo H, et al., 2011, Hesr1 and Hesr3 are essential to generate undifferentiated quiescent satellite cells and to maintain satellite cell numbers. Development, 138(21):4609–4619.https://doi.org/10.1242/dev.067165
26. Brohl D, Vasyutina E, Czajkowski MT, et al., 2012, Colonization of the satellite cell niche by skeletal muscle progenitor cells depends on Notch signals. Dev Cell,23(3):469–481.https://doi.org/10.1016/j.devcel.2012.07.014
27. Lepper C, Low S, Partridge TA, 2012, The satellite cell builds its nest under Notch’s guidance. Cell Stem Cell, 11(4):443–444.
https://doi.org/10.1016/j.stem.2012.09.010
28. Vieira NM, Elvers I, Alexander MS, et al., 2015, Jagged 1 rescues the Duchenne muscular dystrophy phenotype. Cell,163(5):1204–1213.
https://doi.org/10.1016/j.cell.2015.10.049
29. Gharaibeh B, Lu A, Tebbets J, et al., 2008, Isolation of a slowly adhering cell fraction containing stem cells from murine skeletal muscle by the preplate technique. Nat Protoc, 3(9):1501–1509. https://doi.org/10.1038/nprot.2008.142
30. Ota S, Uehara K, Nozaki M, et al., 2011, Intramuscular transplantation of muscle-derived stem cells accelerates skeletal muscle healing after contusion injury via enhancement of angiogenesis. Am J Sports Med, 39(9):1912–1922.https://doi.org/10.1177/0363546511415239
31. Sekiya N, Tobita K, Beckman S, et al., 2013, Muscle-derived stem cell sheets support pump function and prevent cardiac arrhythmias in a model of chronic myocardial infarction.Mol Ther, 21(3):662–9.https://doi.org/10.1038/mt.2012.266
32. Matai I, Kaur G, Seyedsalehi A, et al., 2020, Progress in 3D bioprinting technology for tissue/organ regenerative engineering. Biomaterials, 226:119536.https://doi.org/10.1016/j.biomaterials.2019.119536
33. Vijayavenkataraman S, Yan WC, Lu WF, et al., 2018, 3D bioprinting of tissues and organs for regenerative medicine.Adv Drug Deliv Rev, 132:296–332.https://doi.org/10.1016/j.addr.2018.07.004
34. Ng WL, Chua CK, Shen Y-F, 2019, Print me an organ! Why we are not there yet. Progr Polym Sci, 97:101145.
https://doi.org/10.1016/j.progpolymsci.2019.101145
35. Ng WL, Lee JM, Zhou M, et al., 2020, Vat polymerizationbased bioprinting-process, materials, applications and regulatory challenges. Biofabrication, 12(2):022001.https://doi.org/10.1088/1758-5090/ab6034
36. Ng WL, Huang X, Shkolnikov V, et al., 2022, Controlling droplet impact velocity and droplet volume: Key factors to achieving high cell viability in sub-nanoliter droplet-based bioprinting. Int J Bioprint, 8(1):424.https://doi.org/10.18063/ijb.v8i1.424
37. Zhou C, Yang Y, Wang J, et al., 2021, Ferromagnetic soft catheter robots for minimally invasive bioprinting. Nat Commun, 12(1):5072.
https://doi.org/10.1038/s41467-021-25386-w
38. Jiang T, Munguia-Lopez GJ, Flores-Torres S, et al., 2019, Extrusion bioprinting of soft materials: An emerging technique for biological model fabrication. Appl Phys Rev,6(1):011310.https://doi.org/10.1063/1.5059393, 10.1063/1.5085013,10.1063/1.5055659, 10.1063/1.5053909
39. Eisenberg MC, Kim Y, Li R, et al., 2011, Mechanistic modeling of the effects of myoferlin on tumor cell invasion.Proc Natl Acad Sci U S A, 108(50):20078–20083.https://doi.org/10.1073/pnas.1116327108
40. Yerneni SS, Whiteside TL, Weiss LE, et al., 2019, Bioprinting exosome-like extracellular vesicle microenvironments. Bioprinting, 13:e00041.
https://doi.org/10.1016/j.bprint.2019.e00041
41. Cooper GM, Miller ED, DeCesare GE, et al., 2010, Inkjetbased biopatterning of bone morphogenetic protein-2 to spatially control calvarial bone formation. Tissue Eng Part A,16:1749–1759.https://doi.org/10.1089/ten.TEA.2009.0650
42. Ker ED, Nain AS, Weiss LE, et al., 2011, Bioprinting ofgrowth factors onto aligned sub-micron fibrous scaffolds for simultaneous control of cell differentiation and alignment.Biomaterials, 32(32):8097–8107.https://doi.org/10.1016/j.biomaterials.2011.07.025
43. Herberg S, Kondrikova G, Periyasamy-Thandavan S, et al., 2014, Inkjet-based biopatterning of SDF-1beta augments BMP-2-induced repair of critical size calvarial bone defects in mice. Bone, 67:95–103.https://doi.org/10.1016/j.bone.2014.07.007
44. Skuk D, Tremblay JP, 2000, Progress in myoblast transplantation: A potential treatment of dystrophies. Microsc Res Tech, 48(3-4):213–222.
https://doi.org/10.1002/(SICI)1097-0029(20000201/15)48:3/4<213::AID-JEMT9>3.0.CO;2-Z
45. Bouchentouf M, Benabdallah BF, Tremblay JP, 2004, Myoblast survival enhancement and transplantation success improvement by heat-shock treatment in mdx mice. Transplantation, 77(9):1349–1356.
46. Skuk D, Caron NJ, Goulet M, et al., 2003, Resetting the problem of cell death following muscle-derived cell transplantation: Detection, dynamics and mechanisms. J Neuropathol Exp Neurol, 62(9):951–967.
47. Qu-Petersen Z, Deasy B, Jankowski R, et al., 2002,Identification of a novel population of muscle stem cells in mice: Potential for muscle regeneration. J Cell Biol,157(5):851–864.https://doi.org/10.1083/jcb.200108150
48. Deasy BM, Jankowski RJ, Huard J, 2001, Muscle-derived stem cells: Characterization and potential for cell-mediated therapy. Blood Cells Mol Dis, 27(5):924–933.https://doi.org/10.1006/bcmd.2001.0463
49. Parker MH, Loretz C, Tyler AE, et al., 2012, Activation of Notch signaling during ex vivo expansion maintains donor muscle cell engraftment. Stem Cells, 30(10):2212–2220.https://doi.org/10.1002/stem.1181