Three-Dimensional Printing Technologies for Drug Delivery Applications: Processes, Materials, and Effects
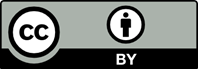
: Since the 1930s, new methods of drug delivery, such as implantable devices with drug release control, have been developed. However, manufacturing techniques require bulk due to high initial production costs. Three-dimensional (3D) printing, also known as additive manufacturing or rapid prototyping, allows the fabrication of personalized drug delivery that uses different materials and complex geometries with multiple release profiles, thereby eradicating high initial costs. Different studies have been developed showing the extensive potential of 3D printing for the pharmaceutical industry, and despite in-depth discussions that have been published, there is no comprehensive review of processes, materials, and effects in drug delivery applications thus far. This review aims to fill this gap by presenting the use of 3D printing technology for drug delivery, exposing the different variations of the technique according to the characteristics, material, and dosage form sought. There are seven main categories of 3D printing according to the standards jointly developed by International Organization for Standardization and American Society for Testing and Materials: material jetting, binder jetting, material extrusion, vat photopolymerization, powder bed fusion, sheet lamination, and directed energy deposition. There are different 3D fabrication processes used for drug delivery applications depending on the dosage form and material applied. In this context, polymers, glasses, and hydrogels represent the most frequent materials used. 3D printing allows different forms of drug dosage. Oral, topical, rectal and vaginal, parental and implantable are discussed in this paper, presenting the identification of the type of 3D printing technology, the active pharmaceutical ingredient, formulation, and pharmaceutical effect. The main aim of this paper is to offer insights to people from academy and industry who are interested in the advancement of drug delivery and in knowing the future directions in the development of 3D printing applications in this area.
1. Florence, A, Attwood, D 2015, Physiochemical Principles of Pharmacy. London: Pharmaceutical Press.
2. Kleiner L, Wright J, Wang Y, 2014, Evolution of Implantable and Insertable Drug Delivery Systems. J Control Release, 181:1–10. https://doi.org/10.1016/j.jconrel.2014.02.006
3. Hoffman A, Hillery A, 2017, Historical Introduction to the Field of Controlled Drug Delivery. Drug Delivery Fundamental Appllications. United States: CRC Press. p1–21.
4. Deanesly R, Parkes A, 1937, Biological Properties of Some New Derivatives of Testosterone. Biochem J, 31:1161–4. https://doi.org/10.1042/bj0311161
5. Schork NJ, 2015, Personalized Medicine: Time for one-person Trials. Nature, 520:609–11. https://doi.org/10.1038/520609a
6. Peak R, 2018, Precision Medicine is not Just Genomics: The right dose for every patient. Ann Rev Pharmacol Toxicol, 58:105–22. https://doi.org/10.1146/annurev-pharmtox-010617-052446
7. Prasad L, Smyth H, 2016, 3D Printing Technologies for Drug Delivery: A Review. Drug Dev Ind Pharm, 42:1019–31. https://doi.org/10.3109/03639045.2015.1120743
8. Syrkel M, 2021, A Brief History of Additive Manufacturing. Arizona: Nexus 3 Manufacturing and Engineering. Available from: https://www.nexus3mfg.com/3d-printing [Last accessed on 2021 Apr 06].
9. International Organization for Standardization/ASTM 52900, 2021, Additive Manufacturing-General Principles-Fundamentals and Vocabulary. Switzerland: International Organization for Standardization. Available from: https://Www.Iso.Org/Obp/Ui/Iso: Std:Iso-Astm:52900:Ed-2:V1:En
10. Jamroz W, Szafraniec J, Kurek M, et al., 2018, 3D Printing in Pharmaceutical and Medical Applications-Recent Achievements and Challenges. Pharm Res, 35:176. https://doi.org/10.1007/s11095-018-2454-x
11. Norman J, Madurawe R, Moore C, et al., 2017, A New Chapter in Pharmaceutical Manufacturing: 3D-printed Drug Products. Adv Drug Deliv Rev, 108:39–50. https://doi.org/10.1016/j.addr.2016.03.001
12. Lim SH, Kathuria H, Yao J, et al., 2018, 3D Printed Drug Delivery and Testing Systems-a Passing Fad or the Future? Adv Drug Deliv Rev, 132:139–68. https://doi.org/10.1016/j.addr.2018.05.006
13. Li J, Rossignol F, Macdonald J, 2015, Inkjet Printing for Biosensor Fabrication: Combining Chemistry and Technology for Advanced Manufacturing. Lab Chip, 15:2538–58. https://doi.org/10.1039/c5lc00235d
14. Redwood B, 2022, Types of 3D Printing. HUBS, Netherlands. Available from: https://www.hubs.com/knowledge-base/types-of-3d-printing [Last accessed on 2022 Apr 03].
15. Loughborough University, 2021, The 7 Categories of Additive Manufacturing. England: Loughborough University. Available from: https://www.lboro.ac.uk/research/amrg/ about/the7categoriesofadditivemanufacturing [Last accessed on 2022 Apr 03].
16. Palo M, Holländer J, Suominen J, et al., 2017, 3D Printed Drug Delivery Devices: Perspectives and Technical Challenges. Expert Rev Med Dev, 14:685–96. https://doi.org/10.1080/17434440.2017.1363647
17. Moulton S, Wallace G, 2014, 3-Dimensional (3D) Fabricated Polymer Based Drug Delivery Systems. J Controlled Release, 193:27–34. https://doi.org/10.1016/j.jconrel.2014.07.005
18. Lee JY, Tan WS, An J, et al., 2016, The Potential to Enhance Membrane Module Design with 3D Printing Technology. J Membr Sci, 499:480–90. https://doi.org/10.1016/j.memsci.2015.11.008
19. Ostendorf A, Chichkov B, 2006, Two-Photon Polymerization: A New Approach to Micromachining. United States: Photonics Spectra. Available from: https://www.photonics. com/Articles/Two-Photon_Polymerization_A_New_ Approach_to/a26907 [Last accessed on 2022 Sep 18].
20. Lee J, Sing S, Zhou M, et al., 2018, 3D Bioprinting Processes: A Perspective on Classification and Terminology. Int J Bioprint, 4:151. https://doi.org/10.18063/IJB.v4i2.151
21. Ge L, Dong L, Wang D, et al., 2018, A Digital Light Processing 3D Printer for Fast and High-Precision Fabrication of Soft Pneumatic Actuators. Sens Actuators A Phys, 273:285–92. https://doi.org/10.1016/j.sna.2018.02.041
22. Kadry H, Wadnap S, Xu C, et al., 2019, Digital Light Processing (DLP) 3D-Printing Technology and Photoreactive Polymers in Fabrication of Modified-Release Tablets. Eur J Pharm Sci, 135:60–7. https://doi.org/10.1016/j.ejps.2019.05.008
23. Carbon 3D, 2022, A Carbon 3D Printer for Every Budget. Available from: https://www.carbon3d.com/products [Last accessed on 2022 Sep 17].
24. James HP, John R, Alex A, et al., 2014 Smart Polymers for the Controlled Delivery of Drugs-a Concise Overview. Acta Pharm Sin B, 4:120–7. https://doi.org/10.1016/j.apsb.2014.02.005
25. Liechty WB, Kryscio DR, Slaughter BV, et al., 2010, Polymers for Drug Delivery Systems. Ann Rev Chem Biomol Eng, 1:149–73. https://doi.org/10.1146/annurev-chembioeng-073009-100847
26. Mahmood M, 2021, 3D Printing in Drug Delivery and Biomedical Applications: A State-of-the-art Review. Compounds, 1:94–115. https://doi.org/10.3390/compounds1030009
27. Zhang J, Zhao S, Zhu Y, et al., 2014, Three-dimensional Printing of Strontium-Containing Mesoporous Bioactive Glass Scaffolds for Bone Regeneration. Acta Biomater, 10:2269–81. https://doi.org/10.1016/j.actbio.2014.01.001
28. Soundrapandian C, Datta S, Kundu B, et al., 2010, Porous Bioactive Glass Scaffolds for Local Drug Delivery in Osteomyelitis: Development and in Vitro Characterization. AAPS PharmaSciTech, 11:1675–83. https://doi.org/10.1208/s12249-010-9550-5
29. Wu C, Chang J, Xiao Y, 2011, Mesoporous Bioactive Glasses as Drug Delivery and Bone Tissue Regeneration Platforms. Ther Deliv, 2:1189–98. https://doi.org/10.4155/tde.11.84
30. Hoare TR, Kohane DS, 2008, Hydrogels in Drug Delivery: Progress and Challenges. Polymer, 49:1993–2007. https://doi.org/10.1016/j.polymer.2008.01.027
31. Santoro M, Tatara AM, Mikos AG, 2014, Gelatin Carriers for Drug and Cell Delivery in Tissue Engineering. J Controlled Release, 190:210–8. https://doi.org/10.1016/j.jconrel.2014.04.014
32. Trenfield S, Awad A, Goyanes A, et al., 2018, 3D Printing Pharmaceuticals: Drug Development to Frontline Care. Trends Pharmacol Sci, 39:440–51. https://doi.org/10.1016/j.tips.2018.02.006
33. Katstra W, Palazzolo R, Rowe C, et al., 2000, Oral Dosage Forms Fabricated by Three Dimensional Printing. J Controlled Release, 66:1–9. https://doi.org/10.1016/S0168-3659(99)00225-4
34. Rowe CW, Katstra WE, Palazzolo RD, et al., 2000, Multimechanism Oral Dosage forms Fabricated by Three Dimensional Printing. J Controlled Release, 66:11–7. https://doi.org/10.1016/S0168-3659(99)00224-2
35. Groll J, Burdick J, Cho D, et al., 2018, A Definition of Bioinks and their Distinction from Biomaterials Inks. Biofabrication, 11:013001.
36. Hsu H, Harris M, Toth S, et al., 2015, Drop Printing of Pharmaceuticals: Effect of Molecular Weight on PEG Coatednaproxen/PEG 3350 Solid Dispersions. AIChE J, 61:4502–8. https://doi.org/10.1002/aic.14979
37. Kyobula M, Adedeji A, Alexander MR, et al., (2017), 3D Inkjet Printing of Tablets Exploiting Bespoke Complex Geometries for Controlled and Tuneable Drug Release. J Controlled Release, 261:207–15. https://doi.org/10.1016/j.jconrel.2017.06.025
38. Alhijjaj M, Belton P, Qi S, 2016, An Investigation into the use of Polymer Blends to Improve the Printability of and Regulate Drug Release from Pharmaceutical Solid Dispersions Prepared Via Fused Deposition Modeling (FDM) 3D Printing. Eur J Pharm Biopharm, 108:111–25. https://doi.org/10.1016/j.ejpb.2016.08.016
39. Arafat B, Wojsz M, Isreb A, et al., 2018, Tablet Fragmentation without a Disintegrant: A Novel Design Approach for Accelerating Disintegration and Drug Release from 3D Printed Cellulosic Tablets. Eur J Pharm Sci, 118:191–9. https://doi.org/10.1016/j.ejps.2018.03.019
40. Sadia M, Arafat B, Ahmed W, et al., 2018, Channelled Tablets: An Innovative Approach to Accelerating Drug Release from 3D Printed Tablets. J Controlled Release, 269:355–63. https://doi.org/10.1016/j.jconrel.2017.11.022
41. Goyanes A, Buanz AB, Hatton GB, et al., 2015, 3D Printing of Modified-release Aminosalicylate (4-ASA and 5-ASA) Tablets. Eur J Pharm Biopharm, 89:157–62. https://doi.org/10.1016/j.ejpb.2014.12.003
42. Goyanes A, Chang H, Sedough D, et al., 2015, Fabrication of Controlled-release Budesonide Tablets via Desktop (FDM) 3D Printing. Int J Pharm, 496:414–20. https://doi.org/10.1016/j.ijpharm.2015.10.039
43. Li Q, Guan X, Cui M, et al., 2018, Preparation and Investigation of Novel Gastro-Floating Tablets with 3D Extrusion-based Printing. Int J Pharm, 535:325–32. https://doi.org/10.1016/j.ijpharm.2017.10.037
44. Chai X, Chai H, Wang X, et al., 2017, Fused Deposition Modeling (FDM) 3D Printed Tablets for Intragastric Floating Delivery of Domperidone. Sci Rep, 7:2829. https://doi.org/10.1038/s41598-017-03097-x
45. Cader HK, Rance GA, Alexander MR, et al., 2019, Waterbased 3D Inkjet Printing of an Oral Pharmaceutical Dosage form. Int J Pharm, 564:359–68. https://doi.org/10.1016/j.ijpharm.2019.04.026
46. Clark E, Alexander M, Irvine D, et al., 2017, 3D Printing of Tablets using Inkjet with UV Photoinitiation. Int J Pharm, 529:523–30. https://doi.org/10.1016/j.ijpharm.2017.06.085
47. Lee K, Kang A, Delfino JJ, et al., 2003, Evaluation of Critical Formulation Factors in the Development of a Rapidly Dispersing Captopril Oral Dosage Form. Drug Dev Ind Pharm, 29:967–79. https://doi.org/10.1081/DDC-120025454
48. Yu D, Shen X, Branford-White C, et al., 2009, Novel Oral Fast-disintegrating Drug Delivery Devices with Predefined Inner Structure Fabricated by Three-dimensional Printing. J Pharm Pharmacol, 61:323–9. https://doi.org/10.1211/jpp.61.03.0006
49. Jacob J, Coyle N, West TG, et al., 2014, Aprecia Pharmaceuticals LLC. Rapid Disperse Dosage form Containing Levetiracetam. US9339489B2, Abstract.
50. Yu DG, Branford-White C, Ma ZH, et al., 2009, Novel Drug Delivery Devices for Providing Linear Release Profiles Fabricated by 3DP. Int J Pharm, 370:160–6. https://doi.org/10.1016/j.ijpharm.2008.12.008
51. Yu DG, Yang X, Huang W, et al., 2007, Tablets with Material Gradients Fabricated by Three-dimensional Printing. J Pharm Sci, 96:2446–56. https://doi.org/10.1002/jps.20864
52. Pietrzak K, Isreb A, Alhnan M, 2015, A Flexible-dose Dispenser for Immediate and Extended Release 3D Printed Tablets. Eur J Pharm Biopharm, 96:380–7. https://doi.org/10.1016/j.ejpb.2015.07.027
53. Skowyra J, Pietrzak K, Alhnan MA, 2015, Fabrication of Extended-release patient-Tailored Prednisolone Tablets via Fused Deposition Modelling (FDM) 3D Printing. Eur J Pharm Sci, 68:11–7. https://doi.org/10.1016/j.ejps.2014.11.009
54. Gioumouxouzis C, Katsamenis O, Bouropoulos N, et al., 2017, 3D Printed Oral Solid Dosage forms Containing Hydrochlorothiazide for Controlled Drug Delivery. J Drug Deliv Sci Technol, 40:164–71. https://doi.org/10.1016/j.jddst.2017.06.008
55. Boetker J, Water JJ, Aho J, et al., 2016, Modifying Release Characteristics from 3D Printed Drug-eluting Products. Eur J Pharm Sci, 90:47–52. https://doi.org/10.1016/j.ejps.2016.03.013
56. Water JJ, Bohr A, Boetker J, et al., 2015, Three-dimensional Printing of Drug-Eluting Implants: Preparation of an Antimicrobial Polylactide Feedstock Material. J Pharm Sci, 104:1099–107. https://doi.org/10.1002/jps.24305
57. Goyanes A, Fina F, Martorana A, et al., 2017, Development of Modified Release 3D Printed Tablets (printlets) with Pharmaceutical Excipients using Additive Manufacturing. Int J Pharm, 527:21–30. https://doi.org/10.1016/j.ijpharm.2017.05.021
58. Zhang J, Feng X, Patil H, et al., 2017, Coupling 3D Printing with Hot-melt Extrusion to Produce Controlled-release Tablets. Int J Pharm, 519:186–97. https://doi.org/10.1016/j.ijpharm.2016.12.049
59. Sadia M, Sośnicka A, Arafat B, et al., 2016, Adaptation of Pharmaceutical Excipients to FDM 3D Printing for the Fabrication of Patient-Tailored Immediate Release Tablets. Int J Pharm, 513:659–68. https://doi.org/10.1016/j.ijpharm.2016.09.050
60. Li Q, Wen H, Jia D, et al., 2017, Preparation and Investigation of Controlled-release Glipizide Novel Oral Device with Three-dimensional Printing. Int J Pharm, 525:5–11. https://doi.org/10.1016/j.ijpharm.2017.03.066
61. Vo AQ, Zhang J, Nyavanandi D, et al., 2020, Hot Melt Extrusion Paired Fused Deposition Modeling 3D Printing to Develop Hydroxypropyl Cellulose Based Floating Tablets of Cinnarizine. Carbohydr Polym, 246:116519. https://doi.org/10.1016/j.carbpol.2020.116519
62. Patel NG, Serajuddin AT, 2021, Development of FDM 3D-Printed Tablets with Rapid Drug Release, High Drugpolymer Miscibility and Reduced Printing Temperature by Applying the Acid-base Supersolubilization (ABS) Principle. Int J Pharm, 600:120524. https://doi.org/10.1016/j.ijpharm.2021.120524
63. Tabriz AG, Nandi U, Hurt AP, et al., 2021, 3D Printed Bilayer Tablet with Dual Controlled Drug Release for Tuberculosis Treatment. Int J Pharm, 593:120147. https://doi.org/10.1016/j.ijpharm.2020.120147
64. Khaled SA, Burley JC, Alexander MR, et al., 2014, Desktop 3D Printing of Controlled Release Pharmaceutical Bilayer Tablets. Int J Pharm, 461:105–11. https://doi.org/10.1016/j.ijpharm.2013.11.021
65. Khaled SA, Burley JC, Alexander MR, et al., 2015, 3D Printing of Tablets Containing Multiple Drugs with Defined Release Profiles. Int J Pharm, 494:643–50. https://doi.org/10.1016/j.ijpharm.2015.07.067
66. Khaled S, Burley J, Alexander M, et al., 2015, 3D Printing of Five-in-one dose Combination Polypill with Defined Immediate and Sustained Release Profiles. J Controlled Release, 217:308–14. https://doi.org/10.1016/j.jconrel.2015.09.028
67. Olmos-Juste R, Guaresti O, Calvo-Correas T, et al., 2021, Design of Drug-loaded 3D Printing Biomaterial Inks and Tailor-made Pharmaceutical forms for Controlled Release. Int J Pharm, 609:121124. https://doi.org/10.1016/j.ijpharm.2021.121124
68. Wang J, Goyanes A, Gaisford S, et al., 2016, Stereolithographic (SLA) 3D Printing of Oral Modified-release Dosage forms. Int J Pharm, 503:207–12. https://doi.org/10.1016/j.ijpharm.2016.03.016
69. Martinez PR, Goyanes A, Basit AW, et al., 2017, Fabrication of Drug-loaded Hydrogels with Stereolithographic 3D Printing. Int J Pharm, 532:313–7. https://doi.org/10.1016/j.ijpharm.2017.09.003
70. Chen K, Zeng J, Lin G, 2022, Fabrication of 5-Fluorouracilloaded Tablets with Hyperbranched Polyester by Digital Light Processing 3D Printing Technology. Eur Polym J, 171:111190.
https://doi.org/10.1016/j.eurpolymj.2022.111190
71. Fina F, Madla C, Goyanes A, et al., 2018, Fabricating 3D Printed Orally Disintegrating Printlets using Selective Laser Sintering. Int J Pharm, 541:101–7. https://doi.org/10.1016/j.ijpharm.2018.02.015
72. Fina F, Goyanes A, Gaisford S, et al., 2017, Selective Laser Sintering (SLS) 3D Printing of Medicines. Int J Pharm, 529:285–93. https://doi.org/10.1016/j.ijpharm.2017.06.082
73. Goyanes A, Kobayashi M, Martínez-Pacheco R, et al., 2016, Fused-filament 3D Printing of Drug Products: Microstructure Analysis and Drug Release Characteristics of PVA-based Caplets. Int J Pharm, 514:290–5. https://doi.org/10.1016/j.ijpharm.2016.06.021
74. Maroni A, Melocchi A, Parietti F, et al., 2017, 3D Printed Multi-compartment Capsular Devices for Two-pulse Oral Drug Delivery. J Controlled Release, 268:10–8. https://doi.org/10.1016/j.jconrel.2017.10.008
75. Genina N, Janßen E, Breitenbach A, et al., 2013, Evaluation of Different Substrates for Inkjet Printing of Rasagiline Mesylate. Eur J Pharm Biopharm, 85:1075–83. https://doi.org/10.1016/j.ejpb.2013.03.017
76. Genina N, Fors D, Palo M, et al., 2013, Behavior of Printable Formulations of Loperamide and Caffeine on Different Substrates-Effect of Print Density in Inkjet Printing. Int J Pharm, 453:488–97. https://doi.org/10.1016/j.ijpharm.2013.06.003
77. Meléndez P, Kane K, Ashvar C, et al., 2008, Thermal Inkjet Application in the Preparation of Oral Dosage Forms: Dispensing of Prednisolone Solutions and Polymorphic Characterization by Solid-State Spectroscopic Techniques. J Pharm Sci, 97:2619–36. https://doi.org/10.1002/jps.21189
78. Takala M, Helkiö H, Sundholm J, et al., 2012, Ink-jet Printing of Pharmaceuticals. In: 8th International DAAAM Baltic Conference. p233–9.
79. Wimmer-Teubenbacher M, Planchette C, Pichler H, et al., 2018, Pharmaceutical-grade Oral Films as Substrates for Printed Medicine. Int J Pharm, 547:169–80. https://doi.org/10.1016/j.ijpharm.2018.05.041
80. Thabet Y, Lunter D, Breitkreutz J, 2018, Continuous Inkjet Printing of Enalapril Maleate onto Orodispersible Film Formulations. Int J Pharm, 546:180–7. https://doi.org/10.1016/j.ijpharm.2018.04.064
81. Boudriau S, Hanzel C, Massicotte J, et al., 2016, Randomized Comparative Bioavailability of a Novel Three-dimensional Printed Fast-Melt Formulation of Levetiracetam Following the Administration of a Single 1000-mg Dose to Healthy Human Volunteers Under Fasting and Fed Conditions. Drugs R D, 16:229–38. https://doi.org/10.1007/s40268-016-0132-1
82. Jamróz W, Kurek M, Łyszczarz E, et al., 2017, 3D Printed Orodispersible Films with Aripiprazole. Int J Pharm, 533:413–20. https://doi.org/10.1016/j.ijpharm.2017.05.052
83. Sjöholm E, Sandler N, 2019, Additive Manufacturing of Personalized Orodispersible Warfarin Films. Int J Pharm, 564:117–23. https://doi.org/10.1016/j.ijpharm.2019.04.018
84. He S, Radeke C, Jacobsen J, et al., 2021, Multi-material 3D Printing of Programmable and Stretchable Oromucosal Patches for Delivery of Saquinavir. Int J Pharm, 610:121236. https://doi.org/10.1016/j.ijpharm.2021.121236
85. Goyanes A, Det-Amornrat U, Wang J, et al., 2016, 3D Scanning and 3D Printing as Innovative Technologies for Fabricating Personalized Topical Drug Delivery Systems. J Controlled Release, 234:41–8. https://doi.org/10.1016/j.jconrel.2016.05.034
86. Muwaffak Z, Goyanes A, Clark V, et al., 2017, Patient-specific 3D Scanned and 3D Printed Antimicrobial Polycaprolactone Wound Dressings. Int J Pharm, 527:161–70. https://doi.org/10.1016/j.ijpharm.2017.04.077
87. Wei Y, Li-Tsang CW, Liu J, et al., 2017, 3D-Printed Transparent Facemasks in the Treatment of Facial Hypertrophic Scars of Young Children with Burns. Burns, 43:e19–26. https://doi.org/10.1016/j.burns.2016.08.034
88. Azizoglu E, Ozer O, 2020, Fabrication of Montelukast Sodium Loaded Filaments and 3D Printing Transdermal Patches onto Packaging Material. Int J Pharm, 587:119588. https://doi.org/10.1016/j.ijpharm.2020.119588
89. Teoh JH, Tay SM, Fuh J, et al., 2022, Fabricating Scalable, Personalized Wound Dressings with Customizable Drug Loadings via 3D Printing. J Controlled Release, 341:80–94. https://doi.org/10.1016/j.jconrel.2021.11.017
90. Sun Y, Ruan X, Li H, et al., 2016, Fabrication of Non dissolving Analgesic Suppositories using 3D Printed Moulds. Int J Pharm, 513:717–24. https://doi.org/10.1016/j.ijpharm.2016.09.073
91. Chatzitaki AT, Tsongas K, Tzimtzimis EK, et al., 2021, 3D Printing of Patient-Tailored SNEDDS-based Suppositories of Lidocaine. J Drug Deliv Sci Technol, 61:102292. https://doi.org/10.1016/j.jddst.2020.102292
92. Holländer J, Genina N, Jukarainen H, et al., 2016, Three- Dimensional Printed PCL-Based Implantable Prototypes of Medical Devices for Controlled Drug Delivery. J Pharm Sci, 05:2665–76. https://doi.org/10.1016/j.xphs.2015.12.012
93. Genina N, Holländer J, Jukarainen H, et al., 2016, Ethylene Vinyl Acetate (EVA) as a New Drug Carrier for 3D Printed Medical Drug Delivery Devices. Eur J Pharm Sci, 90:53–63. https://doi.org/10.1016/j.ejps.2015.11.005
94. Tappa K, Jammalamadaka U, Ballard D, et al., 2017, Medication Eluting Devices for the Field of OBGYN (MEDOBGYN): 3D Printed Biodegradable Hormone Eluting Constructs, a Proof of Concept Study. PLoS One, 12:e0182929. https://doi.org/10.1371/journal.pone.0182929
95. Eder S, Wiltschko L, Koutsamanis I, et al., 2022, Toward a New Generation of Vaginal Pessaries via 3D-printing: Concomitant Mechanical Support and Drug Delivery. Eur J Pharm Biopharm, 174:77–89. https://doi.org/10.1016/j.ejpb.2022.04.001
96. Indurkhya A, Patel M, Sharma P, et al., 2018, Chapter 6 Influence of Drug Properties and Routes of Drug Administration on the Design of Controlled Release System. Adv Pharm Prod Dev Res, i:179–223. https://doi.org/10.1016/B978-0-12-814423-7.00006-X
97. Kim YC, Park JH, Prausnitz MR, 2012, Microneedles for Drug and Vaccine Delivery. Adv Drug Deliv Rev, 64:1547–68. https://doi.org/10.1016/j.addr.2012.04.005
98. Pere CP, Economidou SN, Lall G, et al., 2018, 3D Printed Microneedles for Insulin Skin Delivery. Int J Pharm, 544:425–32. https://doi.org/10.1016/j.ijpharm.2018.03.031
99. Lim S, Ng J, Kang L, 2017, Three-dimensional Printing of a Microneedle Array on Personalized Curved Surfaces for Dual-pronged Treatment of Trigger Finger. Biofabrication, 9:015010. https://doi.org/10.1088/1758-5090/9/1/015010
100. Uddin M, Scoutaris N, Klepetsanis P, et al., 2015, Inkjet Printing of Transdermal Microneedles for the Delivery of Anticancer Agents. Int J Pharm, 494:593–602. https://doi.org/10.1016/j.ijpharm.2015.01.038
101. Gittard S, Ovsianikov A, Akar H, et al., 2010, Two Photon Polymerization-Micromolding of Polyethylene Glycol-Gentamicin Sulfate Microneedles. Adv Eng Mater, 12:B77–82. https://doi.org/10.1002/adem.200980012
102. Gittard S, Miller P, Jin C, et al., 2011, Deposition of Antimicrobial Coatings on Microstereolithographyfabricated Microneedles. JOM, 63:59–68. https://doi.org/10.1007/s11837-011-0093-3
103. Shin D, Hyun J, 2021, Silk Fibroin Microneedles Fabricated by Digital Light Processing 3D Printing. J Ind Eng Chem, 95:126–33. https://doi.org/10.1016/j.jiec.2020.12.011
104. Johnson A, Caudill C, Tumbleston J, et al., 2016, Single-Step Fabrication of Computationally Designed Microneedles by Continuous Liquid Interface Production. PLoS One, 11:e0162518. https://doi.org/10.1371/journal.pone.0162518
105. Wu W, Zheng Q, Guo X, et al., 2009, A programmed Release Multi-drug Implant Fabricated by Three-dimensional Printing Technology for Bone Tuberculosis Therapy. Biomed Mater, 4:065005. https://doi.org/10.1088/1748-6041/4/6/065005
106. Wu W, Zheng Q, Guo X, et al., 2009, The Controlled releasing Drug Implant Based on the Three Dimensional Printing Technology: Fabrication and Properties of Drug Releasing in Vivo. J Wuhan Univ Technol Mater Sci Ed, 24:977–81. https://doi.org/10.1007/s11595-009-6977-1
107. Wu G, Wu W, Zheng Q, et al., 2014, Experimental Study of PLLA/INH Slow Release Implant Fabricated by Three Dimensional Printing Technique and Drug Release Characteristics in vitro. BioMed Eng Online, 13:97. https://doi.org/10.1186/1475-925X-13-97
108. Wu W, Ye C, Zheng Q, et al., 2016, A Therapeutic Delivery System for Chronic Osteomyelitis via a Multi-drug Implant Based on Three-dimensional Printing Technology. J Biomater Appl, 31:250–60. https://doi.org/10.1177/0885328216640660
109. Sandler N, Salmela I, Fallarero A, et al., 2014, Towards Fabrication of 3D Printed Medical Devices to Prevent Biofilm Formation. Int J Pharm, 459:62–4. https://doi.org/10.1016/j.ijpharm.2013.11.001
110. Ballard D, Weisman J, Jammalamadaka U, et al., 2017, Three-dimensional Printing of Bioactive Hernia Meshes: In vitro Proof of Principle. Surgery, 161:1479–81. https://doi.org/10.1016/j.surg.2016.08.033
111. Weisman JA, Jammalamadaka U, Tappa K, et al., 2015, 3D Printing Antibiotic and Chemotherapeutic Eluting Catheters and Constructs. J Vasc Interv Radiol, 26:S12. https://doi.org/10.1016/j.jvir.2014.12.040
112. Misra SK, Ostadhossein F, Babu R, et al., 2017, 3D-Printed Multidrug-Eluting Stent from Graphene-Nanoplatelet-Doped Biodegradable Polymer Composite. Adv Healthc Mater, 6:1700008. https://doi.org/10.1002/adhm.201700008
113. Cui M, Hu N, Fang D, et al., 2022, Fabrication and Evaluation of Customized Implantable Drug Delivery System for Orthopedic Therapy Based on 3D Printing Technologies. Int J Pharm, 618:121679. https://doi.org/10.1016/j.ijpharm.2022.121679
114. Zhu M, Wang H, Liu J, et al., 2011, A Mesoporous Silica Nanoparticulate/β-TCP/BG Composite Drug Delivery System for Osteoarticular Tuberculosis Therapy. Biomaterials, 32 (7), 1986–95. https://doi.org/10.1016/j.biomaterials.2010.11.025
115. Zhang J, Zhao S, Zhu M, et al., 2014, 3D-printed Magnetic Fe3O4/MBG/PCL Composite Scaffolds with Multifunctionality of Bone Regeneration, Local Anticancer Drug Delivery and Hyperthermia. J Mater Chem B, 2:7583–95. https://doi.org/10.1039/C4TB01063A
116. Min Z, Shichang Z, Chen X, et al., 2015, 3D-printed Dimethyloxallyl Glycine Delivery Scaffolds to Improve Angiogenesis and Osteogenesis. Biomater Sci, 3:1236–44. https://doi.org/10.1039/C5BM00132C
117. Ahlfeld T, Akkineni A, Förster Y, et al., 2016, Design and Fabrication of Complex Scaffolds for Bone Defect Healing: Combined 3D Plotting of a Calcium Phosphate Cement and a Growth Factor-Loaded Hydrogel. Ann Biomed Eng, 45:224–36. https://doi.org/10.1007/s10439-016-1685-4
118. Cui M, Pan H, Li L, et al., 2021, Exploration and Preparation of Patient-specific Ciprofloxacin Implants Drug Delivery System Via 3D Printing Technologies. J Pharm Sci, 110:3678–89. https://doi.org/10.1016/j.xphs.2021.08.004
119. Cui M, Pan H, Fang D, et al., 2022, 3D Printed Personalized Amikacin Sulfate Local Drug Delivery System for Bone Defect Therapy. J Drug Deliv Sci Technol, 70:103208. https://doi.org/10.1016/j.jddst.2022.103208
120. Maher S, Kaur G, Lima-Marques L, et al., 2017, Engineering of Micro-to Nanostructured 3D-Printed Drug-Releasing Titanium Implants for Enhanced Osseointegration and Localized Delivery of Anticancer Drugs. ACS Appl Mater Interfaces, 9:29562–70. https://doi.org/10.1021/acsami.7b09916
121. Parry JA, Olthof MG, Shogren KL, et al., 2017, Three- Dimension-Printed Porous Poly (Propylene Fumarate) Scaffolds with Delayed rhBMP-2 Release for Anterior Cruciate Ligament Graft Fixation. Tissue Eng Part A, 23:359–65. https://doi.org/10.1089/ten.tea.2016.0343
122. Huang W, Zheng Q, Sun W, et al., 2007, Levofloxacin Implants with Predefined Microstructure Fabricated by Three-dimensional Printing Technique. Int J Pharm, 339:33–8. https://doi.org/10.1016/j.ijpharm.2007.02.021
123. Xu X, Goyanes A, Trenfield SJ, et al., 2021, Stereolithography (SLA) 3D Printing of a Bladder Device for Intravesical Drug Delivery. Mater Sci Eng C Mater Biol Appl, 120:111773. https://doi.org/10.1016/j.msec.2020.111773
124. Soetedjo AA, Lee JM, Lau HH, et al., 2021, Tissue Engineering and 3D Printing of Bioartificial Pancreas for Regenerative Medicine in Diabetes. Trends Endocrinol Metab, 32:609–22. https://doi.org/10.1016/j.tem.2021.05.007
125. Dhavalikar P, Lan Z, Kar R, et al., 2020, 1.4.8-Biomedical Applications of Additive Manufacturing. Biomater Sci (Fourth Ed), 623–39. https://doi.org/10.1016/B978-0-12-816137-1.00040-4
126. Barua R, Datta S, Roychowdhury A, et al., 2019, “Importance of 3D printing technology in medical fields”. In: Zindani D, Paulo J, Kumar DK, editors. Additive Manufacturing Technologies From an Optimization Perspective. United States: IGI Global. p21–40. https://doi.org/10.4018/978-1-5225-9167-2.ch002
127. Seoane-Viano I, Trenfield SJ, Basit AW, et al., 2021, Translating 3D Printed Pharmaceuticals: From Hype to Real-world Clinical Applications. Adv Drug Deliv Rev, 174:553–75. https://doi.org/10.1016/j.addr.2021.05.003
128. Kalyan BG, Kumar L, 2022, 3D Printing: Applications in Tissue Engineering, Medical Devices, and Drug Delivery. AAPS PharmSciTech, 23:92. https://doi.org/10.1208/s12249-022-02242-8
129. Morrison RJ, Kashlan KN, Flanangan CL, et al., 2015, Regulatory Considerations in the Design and Manufacturing of Implantable 3D-Printed Medical Devices. Clin Transl Sci, 8:594–600. https://doi.org/10.1111/cts.12315
130. Mohapatra S, Kar RK, Biswal PK, et al., 2022, Approaches of 3D Printing in Current Drug Delivery. Sensors Int, 3:100146. https://doi.org/10.1016/j.sintl.2021.100146
131. Varghese R, Sood P, Salvi S, et al., 2022, 3D Printing in the Pharmaceutical Sector: Advances and Evidences. Sensors Int, 3:100177. https://doi.org/10.1016/j.sintl.2022.100177
132. Ng WL, Lee JM, Zhou M, et al., 2020, Vat polymerization Based Bioprinting-process, Materials, Applications and Regulatory Challenges. Biofabrication, 12:022001. https://doi.org/10.1088/1758-5090/ab6034
133. Smith J, Shanler M, 2021, Hype Cycle for Life Science Research and Development, 2021. Stamford: Gartner Research. p1–128.
134. Ong JJ, Muniz B, Gaisford S, et al., 2022, Accelerating 3D Printing of Pharmaceutical Products using Machine Learning. Int J Pharm, 4:100120. https://doi.org/10.1016/j.ijpx.2022.100120
135. Yu C, Jiang J, 2020, A Perspective on using Machine Learning in 3D Bioprinting. Int J Bioprint, 6:253. https://doi.org/10.18063/ijb.v6i1.253
136. Lao W, Li M, Wong TN, et al., 2020, Improving Surface Finish Quality in Extrusion-based 3D Concrete Printing using Machine Learning-based Extrudate Geometry Control. Virtual Phys Prototyp, 15:178–93.
137. Ng WL, Chan A, Ong YS, et al., 2020, Deep Learning for Fabrication and Maturation of 3D Bioprinted Tissues and Organs. Virtual Phys Prototyp, 15:340–58.
138. Sing SL, Kuo CN, Shih CT, et al., 2021, Perspectives of using Machine Learning in Laser Powder Bed Fusion for Metal Additive Manufacturing. Virtual Phys Prototyp, 16:372–86.
139. Lyu J, Manoochehri S, 2021, Online Convolutional Neural Network-based Anomaly Detection and Quality Control for Fused Filament Fabrication Process. Virtual Phys Prototyp, 16:160–77.
140. An J, Chua CK, Mironov V, 2021, Application of Machine Learning in 3D Bioprinting: Focus on Development of Big Data and Digital Twin. Int J Bioprint, 7:342. https://doi.org/10.18063/ijb.v7i1.342
141. Fu Z, Angeline V, Sun W, 2021, Evaluation of Printing Parameters on 3D Extrusion Printing of Pluronic Hydrogels and Machine Learning Guided Parameter Recommendation. Int J Bioprint, 7:434. https://doi.org/10.18063/ijb.v7i4.434
142. Dong G, Yeong WY, 2022, Applications of Machine Learning in 3D Printing. Mater Today Proceed, 54:2214–7853. https://doi.org/10.1016/j.matpr.2022.08.551
143. Rahmani S, Ozcan O, Tasoglu S, 2022, Machine Learning- Enabled Optimization of Extrusion-Based 3D printing. Methods, 206:27–40. https://doi.org/10.1016/j.ymeth.2022.08.002
144. Elbadawi M, McCoubrey LE, Gavins FK, et al., 2021, Disrupting 3D Printing of Medicines with Machine Learning. Trends Pharmacol Sci, 42:745–57. https://doi.org/10.1016/j.tips.2021.06.002
145. Simões MF, Silva G, Pinto AC, et al., 2020, Artificial Neural Networks Applied to Quality-by-Design: From Formulation Development to Clinical Outcome. Eur J Pharm Biopharm, 152:282–95. https://doi.org/10.1016/j.ejpb.2020.05.012
146. Sarabi MR, Alseed MM, Karagoz AA, et al., 2022, Machine Learning-enabled Prediction of 3D-printed Microneedle Features. Biosensors, 12:491. https://doi.org/10.3390/bios12070491
147. Ahmed A, Arya S, Gupta V, et al., 2021, 4D Printing: Fundamentals, Materials, Applications and Challenges. Polymer, 228:123926. https://doi.org/10.1016/j.polymer.2021.123926
148. Chua CK, 2020, Publication Trends in 3D Bioprinting and 3D Food Printing. Int J Bioprint, 6:257. https://doi.org/10.18063/ijb.v6i1.257