Recombinant Human Collagen-Based Bioinks for the 3D Bioprinting of Full-thickness Human Skin Equivalent
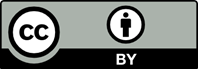
As a major extracellular matrix component within the skin, collagen has been widely used to engineer human skin tissues. However, most collagen is extracted from animals. Here, we introduced recombinant human type III collagen (rhCol3) as a bioactive component to formulate bioinks for the bioprinting of a full-thickness human skin equivalent. Human dermal fibroblasts were encapsulated in the gelatin methacryloyl-rhCol3 composite bioinks and printed on a transwell to form the dermis layer, on which human epidermal keratinocytes were seeded to perform an air-liquid interface culture for 6 weeks. After optimizing the bioink formulation and bioprinting process, we investigated the effect of rhCol3 on skin tissue formation. The results suggest that a higher concentration of rhCol3 would enhance the growth of both cells, resulting in a more confluent (~100%) spreading of the epidermal keratinocytes at an early stage (3 days), compared to the rhCol3-free counterpart. Moreover, in an in vivo experiment, adding rhCol3 in the hydrogel formulation would contribute to the skin wound healing process. Taken together, we conclude that rhCol3 could act as a functional bioink component to promote basic skin cellular processes for skin tissue engineering.
1. Kanitakis J, 2002, Anatomy, histology and immunohistochemistry of normal human skin. Eur J Dermatol, 12:390–9.
2. Gonzales KA, Fuchs E, 2011, Skin and its regenerative powers: An alliance between stem cells and their niche. Dev Cell, 43:387–401. https://doi.org/10.1016/j.devcel.2017.10.001
3. Fuchs E, Raghavan S, 2002, Getting under the skin of epidermal morphogenesis. Nat Rev Genet, 3:199–209. https://doi.org/10.1038/nrg758
4. Watt FM, Huck W, 2013, Role of the extracellular matrix in regulating stem cell fate. Nat Rev Mol Cell Biol, 14:467–73. https://doi.org/10.1038/nrm3620
5. Chung JH, Seo JY, Choi HR, et al., 2001, Modulation of skin collagen metabolism in aged and photoaged human skin in vivo. J Invest Dermatol, 117:1218–24. https://doi.org/10.1046/j.0022-202x.2001.01544.x
6. D’Hondt S, Guillemyn B, Syx D, et al., 2018, Type III collagen affects dermal and vascular collagen fibrillogenesis and tissue integrity in a mutant Col3a1 transgenic mouse model. Matrix Biol, 70:72–83. https://doi.org/10.1016/j.matbio.2018.03.008
7. Guo Y, Bian Z, Xu Q, et al., 2021, Novel tissue-engineered skin equivalent from recombinant human collagen hydrogel and fibroblasts facilitated full-thickness skin defect repair in a mouse model. Mater Sci Eng C Mater Biol Appl, 130:112469. https://doi.org/10.1016/j.msec.2021.112469
8. Zoppi N, Gardella R, De Paepe A, et al., 2004, Human Fibroblasts with Mutations in COL5A1 and COL3A1 Genes Do Not Organize Collagens and Fibronectin in the Extracellular Matrix, Down-regulate α2β1 Integrin, and Recruit αvβ3 Instead of α5β1 Integrin. J Biol Chem, 279:18157–68. https://doi.org/10.1074/jbc.M312609200
9. Epstein EH, 1974, [α1(III)]3 Human Skin Collagen: Release by pepsin digestion and preponderance in fetal life. J Biol Chem, 249:3225–31. https://doi.org/10.1016/S0021-9258(19)42661-6
10. Gref R, Deloménie C, Maksimenko A, et al., 2020, Vitamin C-squalene bioconjugate promotes epidermal thickening and collagen production in human skin. Sci Rep, 10:16883. https://doi.org/10.1038/s41598-020-72704-1
11. Lovell CR, Smolenski KA, Duance VC, et al., 2010, Type I and III collagen content and fibre distribution in normal human skin during ageing. Br J Dermatol, 117:419–28. https://doi.org/10.1111/j.1365-2133.1987.tb04921.x
12. Riekki R, Parikka M, Jukkola A, et al., 2002, Increased expression of collagen Types I and III in human skin as a consequence of radiotherapy. Arch Dermatol Res, 294:178–84. https://doi.org/10.1007/s00403-002-0306-2
13. Cheng W, Yan-Hua R, Fang-Gang N, et al., 2011, The content and ratio of type I and III collagen in skin differ with age and injury. Afr J Biotechnol, 10:2524–9. https://doi.org/10.5897/AJB10.1999
14. Fuchs E, Blau HM, 2020, Tissue stem cells: Architects of their niches. Cell Stem Cell, 27:532–56. https://doi.org/10.1016/j.stem.2020.09.011
15. Yu W, Wang G, Luo X, et al., 2012, Substrate stiffness regulates the proliferation, migration, and differentiation of epidermal cells. Burns, 38:414–20. https://doi.org/10.1016/j.burns.2011.09.002
16. Ng MR, Besser A, Danuser G, et al., 2012, Substrate stiffness regulates cadherin-dependent collective migration through myosin-II contractility. J Cell Biol, 199:545–63. https://doi.org/10.1083/jcb.201207148
17. Gangatirkar P, Paquet-Fifield S, Li A, et al., 2007, Establishment of 3D organotypic cultures using human neonatal epidermal cells. Nat Protoc, 2:178–86. https://doi.org/10.1038/nprot.2006.448
18. Mathes SH, Ruffner H, Graf-Hausner U, 2014, The use of skin models in drug development. Adv Drug Deliv Rev, 69-70:81–102. https://doi.org/10.1016/j.addr.2013.12.006
19. Kim BS, Kwon YW, Kong JS, et al., 2018, 3D cell printing of in vitro stabilized skin model and in vivo pre-vascularized skin patch using tissue-specific extracellular matrix bioink: A step towards advanced skin tissue engineering. Biomaterials, 168:38–53. https://doi.org/10.1016/j.biomaterials.2018.03.040
20. Lee V, Singh G, Trasatti JP, et al., 2014, Design and fabrication of human skin by three-dimensional bioprinting. Tissue Eng Part C Methods, 20:473–84. https://doi.org/10.1089/ ten.TEC.2013.0335
21. Koch L, Deiwick A, Schlie S, et al., 2012, Skin tissue generation by laser cell printing. Biotechnol Bioeng, 109:1855–63. https://doi.org/10.1002/bit.24455
22. Kim BS, Lee JS, Gao G, et al., 2017, Direct 3D cell-printing of human skin with functional transwell system. Biofabrication, 9:025034. https://doi.org/10.1088/1758-5090/aa71c8
23. Fertala A, 2020, Three Decades of Research on Recombinant Collagens: Reinventing the Wheel or Developing New Biomedical Products? Bioengineering (Basel), 7:155. https://doi.org/10.3390/bioengineering7040155
24. Yang L, Wu H, Lu L, et al., 2021, A tailored extracellular matrix (ECM) - Mimetic coating for cardiovascular stents by stepwise assembly of hyaluronic acid and recombinant human Type III collagen. Biomaterials, 276:121055. https://doi.org/10.1016/j.biomaterials.2021.121055
25. Fushimi H, Hiratsuka T, Okamura A, et al., 2020, Recombinant collagen polypeptide as a versatile bone graft biomaterial. Commun Mater, 1:87. https://doi.org/10.1038/s43246-020-00089-9
26. Huang J, Lei X, Huang Z, et al., 2022, Bioprinted gelatin recombinant Type III collagen hydrogel promotes wound healing. Int J Bioprint, 8:517. https://doi.org/10.18063/ijb.v8i2.517
27. Gelse K, Pöschl E, Aigner T, 2003, Collagens–structure, function, and biosynthesis. Adv Drug Deliv Rev, 55:1531–46. https://doi.org/10.1016/j.addr.2003.08.002
28. Zhou F, Hong Y, Liang R, et al., 2020, Rapid printing of bio-inspired 3D tissue constructs for skin regeneration. Biomaterials, 258:120287. https://doi.org/10.1016/j.biomaterials.2020.120287
29. Nielsen MJ, Karsdal MA, 2016, Type III collagen. In: Karsdal MA, editor. Biochemistry of Collagens, Laminins and Elastin. Ch. 3. Massachusetts, United States: Academic Press. p21-30.
30. Ribeiro A, Blokzijl MM, Levato R, et al., 2017, Assessing bioink shape fidelity to aid material development in 3D bioprinting. Biofabrication, 10:014102. https://doi.org/10.1088/1758-5090/aa90e2
31. Ouyang L, Yao R, Zhao Y, et al., 2016, Effect of bioink properties on printability and cell viability for 3D bioplotting of embryonic stem cells. Biofabrication, 8:035020. https://doi.org/10.1088/1758-5090/8/3/035020
32. Bonnans C, Chou J, Werb Z, 2014, Remodelling the extracellular matrix in development and disease. Nat Rev Mol Cell Biol, 15:786–801. https://doi.org/10.1038/nrm3904
33. Reis LA, Chiu L, Yan L, et al., 2012, A peptide-modified chitosan–collagen hydrogel for cardiac cell culture and delivery. Acta Biomater, 8:1022–36. https://doi.org/10.1016/j.actbio.2011.11.030
34. Ouyang L, Wojciechowski JP, 2022, Tunable Microgel-Templated Porogel (MTP) Bioink for 3D Bioprinting Applications. Adv Healthc Mater, 11:e2200027. https://doi.org/10.1002/adhm.202200027
35. Ouyang L, Armstrong JP, Lin Y, et al., 2020, Expanding and optimizing 3D bioprinting capabilities using complementary network bioinks. Sci Adv, 6:eabc5529. https://doi.org/10.1126/sciadv.abc5529
36. Ouyang L, 2022, Pushing the rheological and mechanical boundaries of extrusion-based 3D bioprinting. Trends Biotechnol. 40:891–902. https://doi.org/10.1016/j.tibtech.2022.01.001
37. Barros NR, Kim HJ, Gouidie MJ, et al., 2021, Biofabrication of endothelial cell, dermal fibroblast, and multi-layered keratinocyte layers for skin tissue engineering. Biofabrication, 13:035030. https://doi.org/10.1088/1758-5090/aba503
38. Shie MY, Lee JJ, Ho CC, et al., 2020, Effects of Gelatin Methacrylate Bio-ink Concentration on Mechano-Physical Properties and Human Dermal Fibroblast Behavior. Polymers (Basel), 12:1930. https://doi.org/10.3390/polym12091930
39. Martin P, 1997, Wound healing - Aiming for perfect skin regeneration. Science, 276:75–81. https://doi.org/10.1126/science.276.5309.75
40. Fang C, Yue S, Mohanasundaram P, et al., 2016, Vimentin coordinates fibroblast proliferation and keratinocyte differentiation in wound healing via TGF-β–Slug signaling. Proc Natl Acad Sci U S A, 113:E4320–7. https://doi.org/10.1073/pnas.1519197113
41. Kwon EJ, Park EJ, Yu H, et al., 2018, SIRT-1 regulates TGF-β-induced dermal fibroblast migration via modulation of Cyr61 expression. Connect Tissue Res, 59:245–54. https://doi.org/10.1080/03008207.2017.1360293
42. Baranyi U, Winter B, Gugerell A, et al., 2019, Primary Human Fibroblasts in Culture Switch to a Myofibroblast-Like Phenotype Independently of TGF Beta. Cells, 8:721. https://doi.org/10.3390/cells8070721
43. Ibañez RI, do Amaral RJ, Reis RL, et al., 2021, 3D-Printed Gelatin Methacrylate Scaffolds with Controlled Architecture and Stiffness Modulate the Fibroblast Phenotype towards Dermal Regeneration. Polymers (Basel), 13:2510. https://doi.org/10.3390/polym13152510
44. Chester D, Lee V, Wagner P, et al., 2022, Elucidating the combinatorial effect of substrate stiffness and surface viscoelasticity on cellular phenotype. J Biomed Mater Res A, 110:1224–37. https://doi.org/10.1002/jbm.a.37367
45. Aldana AA, Valente F, Dilley R, et al., 2021, Development of 3D bioprinted GelMA-alginate hydrogels with tunable mechanical properties. Bioprinting, 21:e00105. https://doi.org/10.1016/j.bprint.2020.e00105
46. Ha JH, Lim JH, Kim JW, et al., 2021, Conductive GelMA–Collagen–AgNW Blended Hydrogel for Smart Actuator. Polymers (Basel), 13:1217. https://doi.org/10.3390/polym13081217
47. Zhao X, Lang Q, Yildirimer L, et al., 2016, Photocrosslinkable Gelatin Hydrogel for Epidermal Tissue Engineering. Adv Healthc Mater, 5:108–18. https://doi.org/10.1002/adhm.201500005
48. Koster MI, Roop DR, 2004, The role of p63 in development and differentiation of the epidermis. J Dermatol Sci, 34:3–9. https://doi.org/10.1016/j.jdermsci.2003.10.003
49. Proksch E, Brandner JM, Jensen JM, 2008, The skin: An indispensable barrier. Exp Dermatol, 17:1063–72. https://doi.org/10.1111/j.1600-0625.2008.00786.x
50. Braun S, Hanselmann C, Gassmann MG, et al., 2002, Nrf2 transcription factor, a novel target of keratinocyte growth factor action which regulates gene expression and inflammation in the healing skin wound. Mol Cell Biol, 22:5492–505. https://doi.org/10.1128/MCB.22.15.5492-5505.2002
51. Yin Y, Peng H, Shao J, et al., 2021, NRF2 deficiency sensitizes human keratinocytes to zinc oxide nanoparticles-induced autophagy and cytotoxicity. Environ Toxicol Pharmacol, 87:103721. https://doi.org/10.1016/j.etap.2021.103721