Bioprinting and its Use in Tumor-On-A-Chip Technology for Cancer Drug Screening: A Review
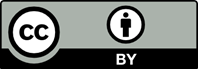
The rising global incidence of cancer and high attrition rates of anticancer drugs make it imperative to design novel screening platforms to increase the success rate of chemotherapeutic agents. Advances in cell culture models from two-dimensional to three-dimensional platforms, along with microfluidics, have resulted in the creation of tumor-on-a-chip technology, which enables high-throughput molecular screening and helps to simulate the dynamic tumor microenvironment. Furthermore, advancements in bioprinting have allowed the structural and physiological aspects of the tumor to be recreated accurately and help to mimic cell-cell interactions and cell-extracellular matrix. This paper provides a comprehensive review of three-dimensional bioprinting to fabricate a tumor-on-a-chip platform to advance the discovery and screening of anticancer agents and provides a perspective on the challenges and future directions associated with the adoption of this technology to advance cancer research.
1. Bray F, Ferlay J, Soerjomataram I, et al., 2018, Global Cancer Statistics 2018: GLOBOCAN Estimates of Incidence and Mortality Worldwide for 36 Cancers in 185 Countries. CA Cancer J Clin, 68:394–424. https://doi.org/10.3322/caac.21492
2. Sung H, Ferlay J, Siegel RL, et al., 2021, Global Cancer Statistics 2020: GLOBACAN Estimates of Incidence and Mortality Worldwide for 36 Cancers in 185 Countries. CA Cancer J Clin, 71:209–49. https://doi.org/10.3322/caac.21660
3. Ferlay J, Colombet M, Soerjomataram I, et al., 2018, Cancer Incidence and Mortality Patterns in Europe: Estimates for 40 Countries and 25 Major Cancers in 2018. Eur J Cancer, 103:356–87. https://doi.org/10.1016/j.ejca.2018.07.005
4. Stewart B, Wild CP, 2014, World Cancer Report 2014. IARC Publication.
5. Knowlton S, Onal S, Yu CH, et al., 2015, Bioprinting for Cancer Research. Trends Biote, 33:504–13.
6. Hay M, Thomas DW, Craighead JL, et al., 2014, Clinical Development Success Rates for Investigational Drugs. Nat Biotechnol, 32:40–51. https://doi.org/10.1038/nbt.2786
7. Smietana K, Siatkowski M, Møller M, 2016, Trends in Clinical Success Rates. Nat Rev Drug Discov, 15:379. https://doi.org/10.1038/nrd.2016.85
8. Heylman C, Sobrino A, Shirure VS, et al., 2014, A strategy for integrating essential three-dimensional microphysiological systems of human organs for realistic anticancer drug screening. Exp Biol Med (Maywood), 239:1240–54. https://doi.org/10.1177/1535370214525295
9. Imamura Y, Mukohara T, Shimono Y, et al., 2015, Comparison of 2D- and 3D-Culture Models as Drug-Testing Platforms in Breast Cancer. Oncol Rep, 33:1837–43. https://doi.org/10.3892/or.2015.3767
10. Chi CW, Lao YH, Ahmed R, et al., 2020, High-Throughput Tumor-on-a-chip Platform to Study Tumor-Stroma Interactions and Drug Pharmacokinetics. Adv Healthc Mater, 9:2000880. https://doi.org/10.1002/adhm.202000880
11. He Y, Nie J, Xie M, et al., 2020, Why Choose 3D Printing? Part III: Printing in 3D Models for Drug Screening. Bio Des Manufac, 3:160–3. https://doi.org/10.1007/s42242-020-00067-7
12. Samadian H, Jafari S, Sepand MR, et al., 2021, 3D Bioprinting Technology to Mimic the Tumor Microenvironment: Tumoron-a-chip Concept. Mat Today Adv, 12:100160. https://doi.org/10.1016/j.mtadv.2021.100160
13. Cao X, Ashfaq R, Cheng F, et al., 2019, A Tumor-on-a-chip System with Bioprinted Blood AND Lymphatic Vessel Pair. Adv Funct Mater, 29:1807173. https://doi.org/10.1002/adfm.201807173
14. Radhakrishnan J, Varadaraj S, Dash SK, et al., 2020, Organotypic Cancer Tissue Models for Drug Screening: 3D Constructs, Bioprinting and Microfluidic Chips. Drug Discov Today, 25:879–90. https://doi.org/10.1016/j.drudis.2020.03.002
15. Mak IW, Evaniew N, Ghert M, 2014, Lost in Translation: Animal Models and Clinical Trials in Cancer Treatment. Am J Transl Res, 6:114–8.
16. Kronemberger GS, Miranda GA, Tavares RS, et al., 2021, Recapitulating Tumorigenesis In Vitro: Opportunities and Challenges of 3D Bioprinting. Front Bioeng Biotechnol, 9:682498. https://doi.org/10.3389/fbioe.2021.682498
17. Nath S, Devi GR, 2016, Three-Dimensional Culture Systems in Cancer Research: Focus on Tumor Spheroid Model. Pharmacol Therap, 163:94–108. https://doi.org/10.1016/j.pharmthera.2016.03.013
18. Zaeri A, Cao K, Zhang F, et al., 2022, A Review of the Structural and Physical Properties that Govern Cell Interactions with Structured Biomaterials Enabled by Additive Manufacturing. Bioprinting, 26:e00201. https://doi.org/10.1016/j.bprint.2022.e00201
19. Augustine R, Kalva SN, Ahmad R, et al., 2021, 3D Bioprinted Cancer Models: Revolutionizing Personalized Cancer Therapy. Trans Oncol, 14:101015. https://doi.org/10.1016/j.tranon.2021.101015
20. Lopatina T, Gai C, Deregibus MC, et al., 2016, Cross Talk Between Cancer and Mesenchymal Stem Cells Through Extracellular Vesicles Carrying Nucleic Acids. Front Oncol, 6:239. https://doi.org/10.3389/fonc.2016.00125
21. Datta P, Dey M, Ataie Z, et al., 2020, 3D Bioprinting for Reconstituting the Cancer Microenvironment. Precision Oncol, 4:18. https://doi.org/10.1038/s41698-020-0121-2
22. Joyce JA, Pollard JW, 2009, Microenvironmental Regulation of Metastasis. Nat Rev Cancer, 9:239.
23. Turley SJ, Cremasco V, Astarita JL, 2015, Immunological Hallmarks of Stromal Cells in the Tumour Microenvironment. Nat Rev Immunol, 15:669. https://doi.org/10.1038/nri3902
24. Cao Y, 2005, Tumor Angiogenesis and Therapy. Biomed Pharmacother, 59:S340–3.
25. Trujillo-de Santiago G, Flores-Garza BG, Tavares-Negrete JA, et al., 2019, The Tumor-on-chip: Recent Advances in the Development of Microfluidic Systems to Recapitulate the Physiology of Solid Tumors. Materials, 12:2945. https://doi.org/10.3390/ma12182945
26. Van den Berg A, Mummery CL, Passier R, et al., 2019, Personalised Organs-on-chips: Functional Testing for Precision Medicine. Lab Chip, 19:198–205. https://doi.org/10.1039/c8lc00827b
27. Han B, Qu C, Park K, et al., 2016, Recapitulation of Complex Transport and Action of Drugs at the Tumor Microenvironment Using Tumor-Microenvironment-on chip. Cancer Lett, 380:319–29. https://doi.org/10.1016/j.canlet.2015.12.003
28. McMaster R, Hoefner C, Hrynevich A, et al., 2019, Tailored Melt Electrowritten Scaffolds for the Generation of Sheetlike Tissue Constructs from Multicellular Spheroids. Adv Healthc Mater, 8:e1801326. https://doi.org/10.1002/adhm.201801326
29. Chiantore MV, Mangino G, Zangrillo MS, et al., 2015, Role of the Microenvironment in Tumourigenesis: Focus on Virus induced Tumors. Curr Med Chem, 22:958–74. https://doi.org/10.2174/0929867322666141212121751
30. Peppicelli S, Andreucci E, Ruzzolini J, et al., 2017, The Acidic Microenvironment as a Possible Niche of Dormant Tumor Cells. Cell Mol Life Sci, 74:2761–71. https://doi.org/10.1007/s00018-017-2496-y
31. Butturini E, de Prati AC, Boriero D, et al., 2019, Tumor Dormancy and Interplay with Hypoxic Tumor Microenvironment. Int J Mol Sci, 20:4305. https://doi.org/10.3390/ijms20174305
32. Wu T, Dai Y, 2017, Tumor Microenvironment and Therapeutic Response. Cancer Lett, 387:61–8.
33. O’Loghlen A, 2018, Role for Extracellular Vesicles in the Tumour Microenvironment. Philos Trans R Soc B Biol Sci, 373:20160488.
34. Erdogan B, Ao M, White LM, et al., 2017, Cancer-Associated Fibroblasts Promote Directional Cancer Cell Migration by Aligning Fibronectin. J Cell Biol, 216:3799–816. https://doi.org/10.1083/jcb.201704053
35. Naumov GN, Folkman J, Straume O, et al., 2008, Tumor-Vascular Interactions and Tumor Dormancy. APMIS, 116:569–85. https://doi.org/10.1111/j.1600-0463.2008.01213.x
36. Quail DF, Joyce JA, 2013, Microenvironmental Regulation of Tumor Progression and Metastasis. Nat Med, 19:1423–37. https://doi.org/10.1038/nm.3394
37. Arneth B, 2019, Tumor Microenvironment. Medicina, 56:15.
38. Hida K, Akiyama K, Ohga N, et al., 2013, Tumour Endothelial Cells Acquire Drug Resistance in a Tumour Microenvironment. J Biochem, 153:243–9. https://doi.org/10.1093/jb/mvs152
39. Sahai E, Astsaturov I, Cukierman E, et al., 2020, A Framework of Advancing Our Understanding of Cancer-associated Fibroblasts. Nat Rev Cancer, 20:174–86. https://doi.org/10.1002/adma.201601504
40. Hanahan D, Coussens LM, 2012, Accessories to the Crime: Functions of Cells Recruited to the Tumor Microenvironment. Cancer Cell, 21:309–22. https://doi.org/10.1016/j.ccr.2012.02.022
41. Sun Z, Wang S, Zhao RC, 2014, The Roles of Mesenchymal Stem Cells in Tumor Inflammatory Microenvironment. J Hematol Oncol, 7:14. https://doi.org/10.1186/1756-8722-7-14
42. Hanahan D, Coussens LM, 2012, Accessories to the Crime: Functions of Cells Recruited to the Tumor Microenvironment. Cancer Cell, 21:309–22. https://doi.org/10.1016/j.ccr.2012.02.022
43. Bi J, Tian Z, 2017, NK Cell Exhaustion. Front Immunol, 8:760.
44. Zhang C, Hu Y, Shi C, 2020, Targeting Natural Killer Cells for Tumor Immunotherapy. Front Immunol, 11:60. https://doi.org/10.3389/fimmu.2020.00060
45. Payne KK, Bear HD, Manjili MH, 2014, Adoptive Cellular Therapy of Cancer: Exploring Innate and Adaptive Cellular Crosstalk to Improve Anti-tumor Efficacy. Future Oncol, 10:1779–94. https://doi.org/10.2217/fon.14.97
46. Nassar D, Blanpain C, 2016, Cancer Stem Cells: Basic Concepts and Therapeutic Implications. Annu Rev Pathol, 11:47–76. https://doi.org/10.1146/annurev-pathol-012615-044438
47. Albini A, Bruno A, Gallo C, et al., 2015, Cancer Stem Cells and the Tumor Microenvironment: Interplay in Tumor Heterogeneity. Connect Tissue Res, 56:414–25. https://doi.org/10.3109/03008207.2015.1066780
48. de Lope LR, Alcíbar OL, López AA, et al., 2018, Tumour adipose Tissue Crosstalk: Fuelling Tumour Metastasis by Extracellular Vesicles. Philos Trans R Soc London Ser B Biol Sci, 373:20160485. https://doi.org/10.1098/rstb.2016.0485
49. Ribeiro AL, Okamoto OK, 2015, Combined Effects of Pericytes in the Tumor Microenvironment. Stem Cells Int, 2015:868475. https://doi.org/10.1155/2015/868475
50. Barrow AD, Colonna M, 2019, Exploiting NK Cell Surveillance Pathways for Cancer Therapy. Cancers, 11:55. https://doi.org/10.3390/cancers11010055
51. Mravic M, Asatrian G, Soo C, et al., 2014, From Pericytes to Perivascular Tumours: Correlation Between Pathology, Stem Cell Biology, and Tissue Engineering. Int Orthop, 38:1819–24. https://doi.org/10.1007/s00264-014-2295-0
52. Insua-Rodriguez J, Oskarsson T, 2016, The Extracellular Matrix in Breast Cancer. Adv Drug Deliv Rev, 97:41–55. https://doi.org/10.1016/j.addr.2015.12.017
53. Cheng YQ, Wang SB, Liu JH, et al., 2020, Modifying the Tumour Microenvironment and Reverting Tumour Cells: New Strategies for Treating Malignant Tumours. Cell Prolif, 53:e12865. https://doi.org/10.1111/cpr.12865
54. Trivanovic D, Krstic J, Djordjevic IO, et al., 2016, The Roles of Mesenchymal Stromal/Stem Cells in Tumor Microenvironment Associated with Inflammation. Mediators Inflamm, 2016:7314016.
55. Sangaletti S, Chiodoni C, Tripodo C, et al., 2017, Common Extracellular Matrix Regulation of Myeloid Cell Activity in the Bone Marrow and Tumor Microenvironments. Cancer Immunol Immunother, 66:1059–67. https://doi.org/10.1007/s00262-017-2014-y
56. Mohammadi H, Sahai E, 2018, Mechanisms and Impact of Altered Tumour Mechanics. Nat Cell Bio, 20:766–74. https://doi.org/10.1038/s41556-018-0131-2
57. Levental KR, Yu H, Kass L, et al., 2009, Matrix Crosslinking Forces Tumor Progression by Enhancing Integrin Signaling. Cell, 139:891-906. https://doi.org/10.1016/j.cell.2009.10.027
58. Masola V, Bellin G, Gambaro G, et al., 2018, Heparanase: A Multitasking Protein Involved in Extracellular Matrix (ECM) Remodeling and Intracellular Events. Cell, 7:236. https://doi.org/10.3390/cells7120236
59. Nallanthighal S, Heiserman JP, Cheon DJ, 2019, The Role of the Extracellular Matrix in Cancer Stemness. Front Cell Dev Biol, 7:86. https://doi.org/10.3389/fcell.2019.00086
60. Tsai HF, Trubelja A, Shen AQ, 2017, Tumour-On-A-Chip: Microfluidic Models of Tumour Morphology, Growth and Microenvironment. J R Soc Interface, 14:20170137. https://doi.org/10.1098/rsif.2017.0137
61. Shoemaker RH, 2016, The NCI60 Human Tumour Cell Line Anticancer Drug Screen. Nat Rev Cancer, 6:813–23. https://doi.org/10.1038/nrc1951
62. Liu X, Fang J, Huang S, et al., 2021, Tumor-on-a-chip: From Bioinspired Design to Biomedical Application. Microsyst Nanoeng, 7:50. https://doi.org/10.1038/s41378-021-00277-8
63. Kapalczynska M, Kolenda T, Przybyla W, et al., 2018, 2D and 3D Cell Cultures-A Comparison of Different Types of Cancer Cell Cultures. Arch Med Sci, 14:910.
64. Ryan SL, Baird AM, Vaz G, et al., 2016, Drug Discovery Approaches Utilizing Three-Dimensional Cell Culture. Assay Drug Dev Technol, 14:19–28. https://doi.org/10.1089/adt.2015.670
65. Brancato V, Oliviera JM, Correlo VM, et al., 2020, Could 3D Models of Cancer Enhance Drug Screening? Biomaterials, 232:119744. https://doi.org/10.1016/j.biomaterials.2019.119744
66. Skardal A, Devarasetty M, Forsythe S, et al., 2016, A Reductionist Metastasis-on-a-chip Platform for In Vitro Tumor Progression Modeling and Drug Screening. Biotechnol Bioeng, 113:2020–32. https://doi.org/10.1002/bit.25950
67. Saglam-Metiner P, Gulce-Iz S, Biray-Avci C, 2019, Bioengineering Inspired Three-Dimensional Culture Systems: Organoids to Create Tumor Microenvironment. Gene, 686:203–12. https://doi.org/10.1016/j.gene.2018.11.058
68. Carvalho V, Maia I, Souza A, et al., 2020, In Vitro Stenotic Arteries to Perform Blood Analogues Flow Visualizations and Measurements: A Review. Open Biomed Eng J, 14:87–102. https://doi.org/10.2174/1874120702014010087
69. Friedrich J, Seidel C, Ebner R, et al., 2009, Spheroid-Based Drug Screen: Considerations and Practical Approach. Nat Protoc, 4:309–24. https://doi.org/10.1038/nprot.2008.226
70. Costa EC, Moreira AF, de Melo-Diogo D, et al., 2016, 3D Tumor Spheroids: An Overview on the Tools and Techniques Used for Their Analysis. Biotechnol Adv, 34:1427–41. https://doi.org/10.1016/j.biotechadv.2016.11.002
71. Hoffmann OI, Ilmberger C, Magosch S, et al., 2015, Impact of the Spheroid Model Complexity on Drug Response. J Biotechnol, 205:14–23. https://doi.org/10.1016/j.jbiotec.2015.02.029
72. Nunes AS, Barros AS, Costa EC, et al., 2019, 3D Tumor Spheroids as In Vitro Models to Mimic In Vivo Human Solid Tumors Resistance to Therapeutic Drugs. Biotechnol Bioeng, 116:206–26. https://doi.org/10.1002/bit.26845
73 Fang Y, Eglen RM, 2017, Three-Dimensional Cell Cultures in Drug Discovery and Development. SLAS Discov, 22:456–72. https://doi.org/10.1177/1087057117696795
74. Kitaeva KV, Rutland CS, Rizvanov AA, et al., 2020, Cell Culture Based In Vitro Test Systems for Anticancer Drug Screening. Front Bioeng Biotechnol, 8:322. https://doi.org/10.3389/fbioe.2020.00322
75. Clevers H, 2016, Modeling Development and Disease with Organoids. Cell, 165:1586–97.
76. Velasco V, Shariati SA, Esfandyarpour R, 2020, Microtechnology-Based Methods for Organoid Models. Microsyst Nanoeng, 6:76. https://doi.org/10.1038/s41378-020-00185-3
77. Lutolf MP, Lauer-Fields JL, Schmoekel HG, et al., 2003, Synthetic Matrix Metalloproteinase-Sensitive Hydrogels for the Conduction of Tissue Regeneration: Engineering Cell Invasion Characteristics. Proc Natl Acad Sci U S A, 100:5413–8. https://doi.org/10.1073/pnas.0737381100
78. Nguyen KT, West JL, 2002, Photopolymerizable Hydrogels for Tissue Engineering Applications. Biomaterials, 23:4307–14. https://doi.org/10.1016/s0142-9612(02)00175-8
79. Charbe N, McCarron PA, Tambuwala MM, 2017, Three-Dimensional Bio-Printing: A New Frontier in Oncology Research. World J Clin Oncol, 8:21. https://doi.org/10.5306/wjco.v8.i1.21
80. Zaeri A, Zgeib R, Cao K, et al., 2022, Numerical Analysis on the Effects of Microfluidic-based Bioprinting Parameters on the Microfiber Geometrical Outcomes. Sci Rep, 12:3364. https://doi.org/10.1038/s41598-022-07392-0
81. Colosi C, Shin SR, Manoharan V, et al., 2016, Microfluidic Bioprinting of Heterogenous 3D Tissue Constructs Using Low-viscosity Bioink. Adv Mater, 28:677–84. https://doi.org/10.1002/adma.201503310
82. Yu Y, Wei W, Wang Y, et al., 2016, Simple Spinning of Heterogeneous Hollow Microfibers on Chip. Adv Mater, 28:6649–55.
83. Murphy SV, Atala A, 2014, 3D Bioprinting of Tissues and Organs. Nat Biotechnol, 32:773–85. https://doi.org/10.1038/nbt.2958
84. Ingber DE, Mow VC, Butler D, et al., 2006, Tissue Engineering and Developmental Biology: Going Biomimetic. Tissue Eng, 12:3265–83. https://doi.org/10.1089/ten.2006.12.3265
85. Mironov V, Visconti RP, Kasyanov V, et al., 2009, Organ Printing: Tissue Spheroids as Building Blocks. Biomaterials, 30:2164–74. https://doi.org/10.1089/ten.2006.12.3265
86. Kelm JM, Lorber V, Snedeker JG, et al., 2010, A Novel Concept for Scaffold-Free Vessel Tissue Engineering: Self-assembly of Microtissue Building Blocks. J Biotechnol, 148:46–55. https://doi.org/10.1016/j.jbiotec.2010.03.002
87. Tiwari AP, Thorat ND, Pricl S, et al., 2021, Bioink: A 3D-Bioprinting Tool for Anticancer Drug Discovery and Cancer Management. Drug Discov Today, 26:1574–90. https://doi.org/10.1016/j.drudis.2021.03.010
88. Hospodiuk M, Dey M, Sosnoski D, et al., 2017, The Bioink: A Comprehensive Review on Bioprintable Materials. Biotechnol Adv, 35:217–39. https://doi.org/10.1016/j.biotechadv.2016.12.006
89. Kleinman HK, Martin GR, 2005, Matrigel: Basement Membrane Matrix with Biological Activity. Semin Cancer Biol, 15:378–86. https://doi.org/10.1016/j.semcancer.2005.05.004
90. Yin Z, Dong C, Jiang K, et al., 2019, Heterogeneity of Cancer-Associated Fibroblasts and Roles in the Progression, Prognosis, and Therapy of Hepatocellular Carcinoma. J Hematol Oncol, 12;101. https://doi.org/10.1186/s13045-019-0782-x
91. Belgodere JA, King CT, Bursavich JB, et al., 2018, Engineering Breast Cancer Microenvironments and 3D Bioprinting. Front Bioeng Biotechnol, 6:66. https://doi.org/10.3389/fbioe.2018.00066
92. Foyt DA, Norma MA, Yu TT, et al., 2018, Exploiting Advanced Hydrogel Technologies to Address Key Challenges in Regenerative Medicine. Adv Healthcare Mat, 7:1700939. https://doi.org/10.1002/adhm.201700939
93. Markstedt K, Mantas A, Tournier I, et al., 2015, 3D Bioprinting Human Chondrocytes with Nanocellulose-Alginate Bioink for Cartilage Tissue Engineering Applications. Biomacromol, 16:1489–96. https://doi.org/10.1021/acs.biomac.5b00188
94. Duan B, Hockaday LA, Hang KH, et al., 2013, 3D Bioprinting of Heterogeneous Aortic Valve Conduits with Alginate/Gelatin Hydrogels. J Biomed Mater Res A, 101:1255–64. https://doi.org/10.1002/jbm.a.34420
95. Norotte C, Marga FS, Niklason LE, et al., 2009, Scaffold-Free Vascular Tissue Engineering Using Bioprinting. Biomaterials, 30:5910–7. https://doi.org/10.1016/j.biomaterials.2009.06.034
96. Rhee S, Puetzer JL, Mason BN, et al., 2016, 3D Bioprinting of Spatially Heterogeneous Collagen Constructs for Cartilage Tissue Engineering. ACS Biomater Sci Eng, 2:1800–5. https://doi.org/10.1021/acsbiomaterials.6b00288
97. Kingsley DM, Roberge CL, Rudkouskaya A, et al., 2019, Laser-Based 3D Bioprinting for Spatial and Size Control of Tumor Spheroids and Embryoid Bodies. Acta Biomater, 95:357–70. https://doi.org/10.1016/j.actbio.2019.02.014
98. Lim KS, Galarraga JH, Cui X, et al., 2020, Fundamentals and Applications of Photo-Cross-Linking in Bioprinting. Chem Rev, 120:10662–94. https://doi.org/10.1021/acs.chemrev.9b00812
99. Shin JY, Yeo YH, Jeong JE, et al., 2020, Dual-Crosslinked Methylcellulose Hydrogels for 3D Bioprinting Applications. Carbohydr Polym, 238:116192. https://doi.org/10.1016/j.carbpol.2020.116192
100. Konig G, McAllister TN, Dusserre N, et al., 2009, Mechanical Properties of Completely Autologous Human Tissue Engineered Blood Vessels Compared to Human Saphenous Vein and Mammary Artery. Biomaterials, 30:1542–50. https://doi.org/10.1016/j.biomaterials.2008.11.011
101. Rago AP, Dean DM, Morgan JP, 2009, Controlling Cell Position in Complex Heterotypic 3D Microtissues by Tissue Fusion. Biotechnol Bioeng, 102:1231–41. https://doi.org/10.1002/bit.22162
102. Xu Q, Norma JT, Shrivastav J, et al., 2007, In Vitro Models of TGF-β-induced Fibrosis Suitable for High-throughput Screening of Antifibrotic Agents. Am J Physiol, 293:F631–40. https://doi.org/10.1152/ajprenal.00379.2006
103. Wang Y, Shi W, Kuss M, et al., 2018, 3D Bioprinting of Breast Cancer Models for Drug Resistance Study. ACS Biomater Sci Eng, 4:4401–11. https://doi.org/10.1021/acsbiomaterials.8b01277
104. Murphy SV, Atala A, 2014, 3D Bioprinting of Tissues and Organs. Nat Biotechnol, 32:773–85. https://doi.org/10.1038/nbt.2958
105. Knowlton S, Joshi A, Yenilmez B, et al., 2016, Advancing Cancer Research Using Bioprinting for Tumour-on-chip Platforms. Int J Bioprint, 2:3–8. https://doi.org/10.18063/ijb.2016.02.003
106. Huh D, Hamilton GA, Ingber DE, 2011, From 3D Cell Culture to Organs-on-chips. Trends Cell Biol, 21:745–54. https://doi.org/10.1016/j.tcb.2011.09.005
107. Chang SF, Chang CA, Lee DY, et al., 2008, Tumour Cell Cycle Arrest Induced by Shear Stress: Roles of Integrins and Smad. Proc Nat Acad Sci, 105:3927–32. https://doi.org/10.1073/pnas.0712353105
108. Skardal A, Aleman J, Forsythe S, et al., 2020, Drug Compound Screening in Single and Integrated Multi-Organoid Body-ona-chip Systems, Biofabrication, 12:025017. https://doi.org/10.1088/1758-5090/ab6d36
109. Bhatia SN, Ingber DE, 2014, Microfluidic Organs-on-chips. Nat Biotechnol, 32:760–72. https://doi.org/10.1038/nbt.2989
110. Yang Q, Lian Q, Xu F, 2017, Perspective: Fabrication of Integrated Organ-On-Achip Via Bioprinting. Biomicrofluidics, 11:031301. https://doi.org/10.1063/1.4982945
111. Asano H, Shiraishi Y, 2015, Development of Paper-Based Microfluidic Analytical Device for Iron Assay Using Photomask Printed with 3D Printer for Fabrication of Hydrophilic and Hydrophobic Zones on Paper by Photolithography. Anal Chim Acta, 883:55–60. https://doi.org/10.1016/j.aca.2015.04.014
112. Carvalho V, Gonçalves I, Lage T, et al., 2021, 3D Printing Techniques and Their Applications to Organ-on-a-chip Platforms: A Systematic Review. Sensors, 21:33014. https://doi.org/10.3390/s21093304
113. Qin D, Xia Y, Whitesides GM, 2010, Soft Lithography for Micro-and Nanoscale Patterning. Nat Protoc, 5, 491–502. https://doi.org/10.1038/nprot.2009.234
114. McDonald JC, Whitesides GM, 2002, Poly (Dimethylsiloxane) as a Material for Fabricating Microfluidic Devices. Acc Chem Res, 35:491–9. https://doi.org/10.1021/ar010110q
115. Thakare K, Jerpseth L, Pei Z, et al., 2021, Bioprinting of Organ-on-chip Systems: A Literature Review from a Manufacturing Perspective. J Manuf Mater Process, 5:91. https://doi.org/10.3390/jmmp5030091
116. Bernard A, Renault JP, Michel B, et al., 2000, Microcontact Printing of Proteins. Adv Mater, 12:1067–70. https://doi.org/10.1002/1521-4095(200007)12:14<1067:aidadma1067> 3.0.co;2-m
117. Auner AW, Tasneem KM, Markov DA, et al., 2019, Chemical-PDMS Binding Kinetics and Implications for Bioavailability in Microfluidic Devices. Lab Chip, 19:864–74. https://doi.org/10.1039/c8lc00796a
118. Nguyen T, et al., 2019, Robust chemical bonding of PMMA microfluidic devices to porous PETE membranes for reliable cytotoxicity testing of drugs. Lab Chip, 19:3706–13. https://doi.org/10.1039/c9lc00338j
119. Dababneh AB, Ozbolat IT, 2014, Bioprinting Technology: A Current State-of-the-art Review. J Manuf Sci Eng, 136:061016.
120. Xu F, Celli J, Rizvi I, et al., 2011, A Three-Dimensional In Vitro Ovarian Cancer Coculture Model Using a High-Throughput Cell Patterning Platform. Biotechnol J, 6:204–12. https://doi.org/10.1002/biot.201000340
121. Keenan TM, Folch A, 2008, Biomolecular Gradients in Cell Culture Systems. Lab Chip, 8:34–57. https://doi.org/10.1039/b711887b
122. Monteiro MV, Zhang YS, Gaspar VM, et al., 2021, 3D-Bioprinted Cancer-on-a-chip: Level-Up Organotypic In Vitro Models. Trends Biotechnol, 40:432–47. https://doi.org/10.1016/j.tibtech.2021.08.007
123. Hamid Q, Wang C, Zhao Y, et al., 2014, A Three-dimensional Cell-laden Microfluidic Chip for In Vitro Drug Metabolism Detection. Biofabrication, 6:025008. https://doi.org/10.1088/1758-5082/6/2/025008
124. Hamid Q, Wang C, Snyder J, et al., 2015, Maskless Fabrication of Cell-laden Microfluidic Chips with Localized Surface Functionalization for the Co-culture of Cancer Cells. Biofabrication, 7:015012. https://doi.org/10.1088/1758-5090/7/1/015012
125. Zhang J, Chen F, He Z, et al., 2016, A Novel Approach for Precisely Controlled Multiple Cell Patterning in Microfluidic Chip by Inkjet Printing and Detection of Drug Metabolism and Diffusion. Analyst, 141:395. https://doi.org/10.1088/1758-5090/7/1/015012
126. Cheng F, Cao X, Li H, et al., 2019, Generation of Cost effective Paper-based Tissue Models Through Matrix-assisted Sacrificial 3D Printing. Nano Lett, 19:3603–11. https://doi.org/10.1021/acs.nanolett.9b00583.s001
127. Li Y, Zhang T, Pang Y, et al., 2019, 3D Bioprinting of Hepatoma Cells and Application with Microfluidics for Pharmacodynamic Test of Metuzumab. Biofabrication, 11:034102. https://doi.org/10.1088/1758-5090/ab256c
128. Mi S, Yang S, Liu T, et al., 2019, A Novel Controllable Cell Array Printing Technique on Microfluidic Chips. Trans Biomed Eng, 66:2512–20. https://doi.org/10.1109/tbme.2019.2891016
129. Yi HG, Jeong YH, Kim Y, et al., 2019, A Bioprinted Glioblastoma-on-a-chip for the Identification of Patient-Specific Responses to Chemoradiotherapy. Nat Biomed Eng, 3:509–519. https://doi.org/10.1038/s41551-019-0363-x
130. Xie M, Gao Q, Fu J, et al., 2020, Bioprinting of Novel 3D Tumor Array Chip for Drug Screening. Biodes Manuf, 3:175–88. https://doi.org/10.1007/s42242-020-00078-4
131. Komen J, van Neerven SM, van den Berg A, et al., 2021, Mimicking and Surpassing the Xenograft Model with Canceron-chip Technology. EBioMedicine, 66:103303. https://doi.org/10.1016/j.ebiom.2021.103303
132. Maulana TI, Kromidas E, Wallstabe L, et al., 2021, Immunocompetent Cancer-on-chip Models to Assess Immuno-oncology Therapy. Adv Drug Del Rev, 173:281–305. https://doi.org/10.1016/j.addr.2021.03.015
133. Krishnamoorthy M, Gerhardt L, Vareki SM, 2021, Immunosuppressive Effects of Myeloid-Derived Suppressor Cells in Cancer and Immunotherapy. Cells, 10:1170. https://doi.org/10.3390/cells10051170
134. Kumar SA, Delgado M, Mendez VE, et al., 2019, Applications of Stem Cells and Bioprinting for Potential Treatment of Diabetes. World J Stem Cells, 11:13–32. https://doi.org/10.4252/wjsc.v11.i1.13
135. Jovic TH, Combellack EJ, Jessop ZM, et al., 2020, 3D Bioprinting and the Future of Surgery. Front Surg, 7:609836. https://doi.org/10.3389/fsurg.2020.609836
136. Izumchenko E, Paz K, Ciznadija D, et al., 2017, Patient derived xenografts effectively capture responses to oncology therapy in a heterogeneous cohort of patients with solid tumors. Ann Oncol, 28:2595–605. https://doi.org/10.1093/annonc/mdx416
137. Zhang N, Yin Y, Xu SJ, et al., 2008, 5-Fluorouracil: Mechanisms of resistance and reversal strategies. Molecules, 13:1551–69. https://doi.org/10.3390/molecules13081551
138. Boso D, Maghin E, Carraro E, et al., 2020, Extracellular matrix-derived hydrogels as biomaterial for different skeletal muscle tissue replacements. Materials (Basel), 13: 2483 https://doi.org/10.3390/ma13112483
139. Weissleder R, Pittet MJ, 2008, Imaging in the Era of Molecular Oncology. Nature, 452:580–9. https://doi.org/10.1038/nature06917