Ecologically Friendly Biofunctional Ink for Reconstruction of Rigid Living Systems Under Wet Conditions
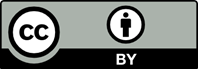
The development of three-dimensional (3D)-printable inks is essential for several applications, from industrial manufacturing to novel applications for biomedical engineering. Remarkably, biomaterials for tissue engineering applications can be expanded to other new horizons; for instance, restoration of rigid living systems as coral reefs is an emergent need derived from recent issues from climate change. The coral reefs have been endangered, which can be observed in the increasing bleaching around the world. Very few studies report eco-friendly inks for matter since most conventional approaches require synthetic polymer, which at some point could be a pollutant depending on the material. Therefore, there is an unmet need for cost-effective formulations from eco-friendly materials for 3D manufacturing to develop carbonate-based inks for coral reef restoration. Our value proposition derives from technologies developed for regenerative medicine, commonly applied for human tissues like bone and cartilage. In our case, we created a novel biomaterial formulation from biopolymers such as gelatin methacrylate, poly (ethylene glycol diacrylate), alginate, and gelatin as scaffold and binder for the calcium carbonate and hydroxyapatite bioceramics needed to mimic the structure of rigid structures. This project presents evidence from 2D/3D manufacturing, chemical, mechanical, and biological characterization, which supports the hypothesis of its utility to aid in the fight to counteract the coral bleaching that affects all the marine ecosystem, primarily when this is supported by solid research in biomaterials science used for living systems, it can extend tissue engineering into new approaches in different domains such as environmental or marine sciences.
1. Chesterman J, Zhang Z, Ortiz O, et al., 2020, Biodegradable Polymers. In: Principles of Tissue Engineering. 5th ed., Ch. 18. Academic Press, United States, p317-342. https://doi.org/10.1016/j.matpr.2020.01.437
2. Biswal T, BadJena SK, Pradhan D, 2020, Sustainable Biomaterials and their Applications: A Short Review. Mater Today, 30:274–82.
3. Simionescu BC, Ivanov D, 2015, Natural and Synthetic Polymers for Designing Composite Materials. In: Handbook of Bioceramics and Biocomposites. Ch. 11-1. Wiley-VCH: Weinheim, Germany, p1–54. https://doi.org/10.1007/978-3-319-09230-0_11-1
4. Lee M, Rizzo R, Surman F, Zenobi-Wong M, 2020, Guiding Lights: Tissue Bioprinting Using Photoactivated Materials. Chem Rev, 120:10950–1027. https://doi.org/10.1021/acs.chemrev.0c00077
5. Chimene D, Miller L, Cross LM, et al., 2020, Nanoengineered Osteoinductive Bioink for 3D Bioprinting Bone Tissue. ACS Appl Mater Interfaces, 12:15976–88. https://doi.org/10.1021/acsami.9b19037
6. Zhou H, Lee J, 2011, Nanoscale Hydroxyapatite Particles for Bone Tissue Engineering. Acta Biomater, 7:2769–81.
7. Kelly BE, Bhattacharya I, Heidari H, et al, 2019, Volumetric Additive Manufacturing Via Tomographic Reconstruction. Science, 363:1075–9. https://doi.org/10.1126/science.aau7114
8. Leggat WP, Camp EF, Suggett DJ, et al., 2019, Rapid Coral Decay is Associated with Marine Heatwave Mortality Events on Reefs. Curr Biol, 29:2723–30.e4. https://doi.org/10.1016/j.cub.2019.06.077
9. Mass T, et al., 2017, Amorphous Calcium Carbonate Particles form Coral Skeletons. Proc Natl Acad Sci, 114:E7670–8. https://doi.org/10.1073/pnas.1707890114
10. Wangpraseurt D, You S, Azam F, et al., 2020, Bionic 3D Printed Corals. Nat Commun, 11:1748.
11. Yu AC, Reinhart M, Hunter R, et al., 2021, Seasonal Impact of Phosphate-Based Fire Retardants on Soil Chemistry Following the Prophylactic Treatment of Vegetation. Environ Sci Technol, 55:2316–23. https://doi.org/10.1021/acs.est.0c05472.s001
12. Loessner D, Meinert C, Kaemmerer E, et al., Functionalization, Preparation and use of Cell-laden Gelatin Methacryloyl based Hydrogels as Modular Tissue Culture Platforms. Nat Protoc, 11:727–46. https://doi.org/10.1038/nprot.2016.037
13. Khan Z, Kahin K, Rauf S, et al., 2019, Optimization of a 3D Bioprinting Process Using Ultrashort Peptide Bioinks. Int J Bioprint, 5:173. https://doi.org/10.18063/ijb.v5i1.173
14. Gong J, Schuurmans CC, van Genderen AM, et al., 2020, Complexation-induced Resolution Enhancement of 3D-Printed Hydrogel Constructs. Nat Commun, 11:1267.
15. Gonzalez-Rios JA, Valle-Pérez AU, Amaya-Delgado L, et al., 2021, A Quick Fed-batch Saccharification Strategy of Wheat Straw at High Solid Loadings Improving Lignocellulosic Ethanol Productivity. Biomass Conversion Biorefinery. https://doi.org/10.1007/s13399-021-01580-0
16. Valle-Pérez AU, Flores-Cosío G, Amaya-Delgado L, 2021, Bioconversion of Agave Bagasse to Produce Cellulases and Xylanases by Penicillium citrinum and Aspergillus fumigatus in Solid-State Fermentation. Waste Biomass Valorization. https://doi.org/10.1007/s12649-021-01397-y
17. Susapto HH, Alhattab D, Abdelrahman S, et al., 2021, Ultrashort Peptide Bioinks Support Automated Printing of Large-Scale Constructs Assuring Long-Term Survival of Printed Tissue Constructs. Nano Lett, 21:2719–29. https://doi.org/10.1021/acs.nanolett.0c04426.s010
18. Theus AS, Ning L, Hwang B, et al., 2020, Bioprintability: Physiomechanical and Biological Requirements of Materials for 3D Bioprinting Processes. Polymers (Basel), 12:2262. https://doi.org/10.3390/polym12102262
19. Doostmohammadi A, Monshi A, Salehi R, et al., 2011, Cytotoxicity Evaluation of 63s Bioactive Glass and Bone-Derived Hydroxyapatite Particles Using Human Bone marrow Stem Cells. Biomed Pap Med Fac Univ Palacky Olomouc Czech Repub, 155:323–6. https://doi.org/10.5507/bp.2011.028
20. Parvez S, Rahman MM, Khan MA, et al., 2012, Preparation and Characterization of Artificial Skin Using Chitosan and Gelatin Composites for Potential Biomedical Application. Polym Bull, 69:715–31. https://doi.org/10.1007/s00289-012-0761-7
21. Guo X, Liu L, Wang W, et al., 2011, Controlled Crystallization of Hierarchical and Porous Calcium Carbonate Crystals Using Polypeptide Type Block Copolymer as Crystal Growth Modifier in a Mixed Solution. Cryst Eng Commun, 13:2011. https://doi.org/10.1039/c0ce00202j
22. Saarai A, Kasparkova V, Sedlacek T, et al., 2013, On the Development and Characterisation of Crosslinked Sodium Alginate/Gelatine Hydrogels. J Mech Behav Biomed Mater, 18:152–66. https://doi.org/10.1016/j.jmbbm.2012.11.010
23. Aldana AA, Malatto L, Rehman MA, et al., 2019, Fabrication of Gelatin Methacrylate (GelMA) Scaffolds with Nano- and Micro-Topographical and Morphological Features. Nanomaterials, 9:120. https://doi.org/10.3390/nano9010120
24. Kontoyannis CG, Vagenas NV, 2000, Calcium Carbonate Phase Analysis Using XRD and FT-Raman Spectroscopy. Analyst, 125:251–5. https://doi.org/10.1039/a908609i
25. Manafi SA, Yazdani B, Rahimiopour MR, et al., 2008, Synthesis of Nano-hydroxyapatite under a Sonochemical/Hydrothermal Condition. Biomed Mater, 3:025002. https://doi.org/10.1088/1748-6041/3/2/025002
26. Duan P, Li X, Wang T, et al., 2018, The Chemical Structure of Carbon Nanothreads Analyzed by Advanced Solid-State NMR. J Am Chem Soc, 140:7658–66. https://doi.org/10.1021/jacs.8b03733
27. Zhu M, Wang Y, Ferracci G, et al., 2019, Gelatin Methacryloyl and its Hydrogels with an Exceptional Degree of Controllability and Batch-to-Batch Consistency. Sci Rep, 9:6863. https://doi.org/10.1038/s41598-019-42186-x
28. Raveendran NT, Vaquette C, Meinert C, et al., 2019, Optimization of 3D Bioprinting of Periodontal Ligament Cells. Dent Mater, 35:1683–94. https://doi.org/10.1016/j.dental.2019.08.114
29. Tan F, Xu X, Deng T, et al., 2012, Fabrication of Positively Charged Poly(Ethylene Glycol)-Diacrylate Hydrogel as a Bone Tissue Engineering Scaffold. Biomed. Mater, 7:055009. https://doi.org/10.1088/1748-6041/7/5/055009
30. Gill P, Moghadam TT, Ranjbar B, 2010, Differential Scanning Calorimetry Techniques: Applications in Biology and Nanoscience. J. Biomol. Technol., 21:167–193.