3D Bioprinting Photo-Crosslinkable Hydrogels for Bone and Cartilage Repair
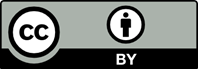
Three-dimensional (3D) bioprinting has become a promising strategy for bone manufacturing, with excellent control over geometry and microarchitectures of the scaffolds. The bioprinting ink for bone and cartilage engineering has thus become the key to developing 3D constructs for bone and cartilage defect repair. Maintaining the balance of cellular viability, drugs or cytokines’ function, and mechanical integrity is critical for constructing 3D bone and/or cartilage scaffolds. Photo-crosslinkable hydrogel is one of the most promising materials in tissue engineering; it can respond to light and induce structural or morphological transition. The biocompatibility, easy fabrication, as well as controllable mechanical and degradation properties of photo-crosslinkable hydrogel can meet various requirements of the bone and cartilage scaffolds, which enable it to serve as an effective bio-ink for 3D bioprinting. Here, in this review, we first introduce commonly used photo-crosslinkable hydrogel materials and additives (such as nanomaterials, functional cells, and drugs/cytokine), and then discuss the applications of the 3D bioprinted photo-crosslinkable hydrogel scaffolds for bone and cartilage engineering. Finally, we conclude the review with future perspectives about the development of 3D bioprinting photo-crosslinkable hydrogels in bone and cartilage engineering.
1. Midha S, Dalela M, Sybil D, et al., 2019, Advances in Three dimensional Bioprinting of Bone: Progress and Challenges. J Tissue Eng Regen Med, 13:925–45. https://doi.org/10.1002/term.2847
2. Liu Y, Luo D, Wang T, 2016, Hierarchical Structures of Bone and Bioinspired Bone Tissue Engineering. Small, 12:4611–32. https://doi.org/10.1002/smll.201600626
3. Bai X, Gao M, Syed S, et al., 2018, Bioactive Hydrogels For Bone Regeneration. Bioact Mater, 3:401–17.
4. Mandrycky C, Wang Z, Kim K, et al., 2016, 3D Bioprinting for Engineering Complex Tissues. Biotechnol Adv, 34:422–34. https://doi.org/10.1016/j.biotechadv.2015.12.011
5. Allen MR, Burr DB, 2014, Bone Modeling and Remodeling. In: Basic and Applied Bone Biology. Amsterdam, Netherlands: Elsevier. p75-90. https://doi.org/10.1016/b978-0-12-416015-6.00004-6
6. Byambaa B, Annabi N, Yue K, et al., 2017, Bioprinted Osteogenic and Vasculogenic Patterns for Engineering 3D Bone Tissue. Adv Healthc Mater, 6:1700015. https://doi.org/10.1002/adhm.201700015
7. Groll J, Boland T, Blunk T, et al., 2016, Biofabrication: Reappraising the Definition of an Evolving Field. Biofabrication, 8:013001. https://doi.org/10.1088/1758-5090/8/1/013001
8. Ashammakhi N, Hasan A, Kaarela O, et al., 2019, Advancing Frontiers in Bone Bioprinting. Adv Healthc Mater, 8:1801048. https://doi.org/10.1002/adhm.201801048
9. Keriquel V, Oliveira H, Rémy M, et al., 2017, In Situ Printing of Mesenchymal Stromal Cells, by Laser-assisted Bioprinting, for In Vivo Bone Regeneration Applications. Sci Rep, 7:1–10. https://doi.org/10.1038/s41598-017-01914-x
10. Yang Y, Zhang Q, Xu T, et al., 2020, Photo crosslinkable Nanocomposite Ink for Printing Strong, Biodegradable and Bioactive Bone Graft. Biomaterials, 263:120378. https://doi.org/10.1016/j.biomaterials.2020.120378
11. Nguyen QV, Park JH, Lee DS, 2015, Injectable Polymeric Hydrogels for the Delivery of Therapeutic Agents: A Review. Eur Polym J, 72:602–619. https://doi.org/10.1016/j.eurpolymj.2015.03.016
12. Yue K, Trujillo-de Santiago G, Alvarez MM, et al., 2015, Synthesis, Properties, and Biomedical Applications of Gelatin Methacryloyl (GelMA) Hydrogels. Biomaterials, 73:254–271. https://doi.org/10.1016/j.biomaterials.2015.08.045
13. Vijayavenkataraman S, Yan WC, Lu WF, et al., 2018, 3D Bioprinting of Tissues and Organs for Regenerative Medicine. Adv Drug Deliv Rev, 132:296–332. https://doi.org/10.1016/j.addr.2018.07.004
14. Li X, Chen S, Li J, et al., 2016, 3D Culture of Chondrocytes in Gelatin Hydrogels with Different Stiffness. Polymers (Basel), 8:269. https://doi.org/10.3390/polym8080269
15. Bryant SJ, Chowdhury TT, Lee DA, et al., 2004, Crosslinking Density Influences Chondrocyte Metabolism in Dynamically Loaded Photocrosslinked Poly (Ethylene Glycol) Hydrogels. Ann Biomed Eng, 32:407–17. https://doi.org/10.1023/b: abme.0000017535.00602.ca
16. Marklein RA, Burdick JA, 2010, Spatially Controlled Hydrogel Mechanics to Modulate Stem Cell Interactions. Soft Matter, 6:136–43. https://doi.org/10.1039/b916933d
17. Bian L, Hou C, Tous E, et al., 2013, The Influence of Hyaluronic Acid Hydrogel Crosslinking Density and Macromolecular Diffusivity on Human MSC Chondrogenesis and Hypertrophy. Biomaterials, 34:413–21. https://doi.org/10.1016/j.biomaterials.2012.09.052
18. Nguyen KT, West JL, 2002, Photopolymerizable Hydrogels for Tissue Engineering Applications. Biomaterials, 23: 4307–14. https://doi.org/10.1016/s0142-9612(02)00175-8
19. Annabi N, Tamayol A, Uquillas JA, et al., 2014, 25th Anniversary Article: Rational Design and Applications of Hydrogels in Regenerative Medicine. Adv Mater, 26:85–124. https://doi.org/10.1002/adma.201303233
20. Jiang Y, Chen J, Deng C, et al., 2014, Click Hydrogels, Microgels and Nanogels: Emerging Platforms for Drug Delivery and Tissue Engineering. Biomaterials, 35:4969–85. https://doi.org/10.1016/j.biomaterials.2014.03.001
21. Van Vlierberghe S, Dubruel P, Schacht EJ, 2011, Biopolymer based Hydrogels as Scaffolds for Tissue Engineering Applications: A Review. Biomacromolecules, 12:1387–408. https://doi.org/10.1021/bm200083n
22. Collins MN, Birkinshaw CJ, 2013, Hyaluronic Acid Based Scaffolds for Tissue Engineering a review. Rom J Morphol Embryol, 92:1262–79. https://doi.org/10.1016/j.carbpol.2012.10.028
23. Sherman L, Sleeman J, Herrlich P, et al., 1994, Hyaluronate Receptors: Key Players in Growth, Differentiation, Migration and Tumor Progression. Curr Opin Cell Biol, 6:726–33. https://doi.org/10.1016/0955-0674(94)90100-7
24. Prestwich GD, 2011, Hyaluronic Acid-based Clinical Biomaterials Derived for Cell and Molecule Delivery in Regenerative Medicine. J Control Release, 155:193–9. https://doi.org/10.1016/j.jconrel.2011.04.007
25. Gramlich WM, Kim IL, Burdick JA, 2013, Synthesis and Orthogonal Photopatterning of Hyaluronic Acid Hydrogels with Thiol-Norbornene Chemistry. Biomaterials, 34:9803–11. https://doi.org/10.1016/j.biomaterials.2013.08.089
26. Oudshoorn MH, Rissmann R, Bouwstra JA, et al., 2007, Synthesis of Methacrylated Hyaluronic Acid with Tailored Degree of Substitution. Polymer, 48:1915–20. https://doi.org/10.1016/j.polymer.2007.01.068
27. Poldervaart MT, Goversen B, De Ruijter M, et al., 2017, 3D Bioprinting of Methacrylated Hyaluronic Acid (MeHA) Hydrogel with Intrinsic Osteogenicity. PLoS One, 12:e0177628. https://doi.org/10.1371/journal.pone.0177628
28. Liu Y, Chan-Park MB, 2010, A Biomimetic Hydrogel Based on Methacrylated Dextran-graft-lysine and Gelatin for 3D Smooth Muscle Cell Culture. Biomaterials, 31:1158–70. https://doi.org/10.1016/j.biomaterials.2009.10.040
29. Lai JY, Li YT, 2010, Functional Assessment of Cross-linked Porous Gelatin Hydrogels for Bioengineered Cell Sheet Carriers. Biomacromolecules, 11:1387–97. https://doi.org/10.1021/bm100213f
30. Schuurman W, Levett PA, Pot MW, et al., 2013, Gelatin methacrylamide Hydrogels as Potential Biomaterials for Fabrication of Tissue-engineered Cartilage Constructs. Macromol Biosci, 13:551–61. https://doi.org/10.1002/mabi.201200471
31. Mũnoz Z, Shih H, Lin CC, 2014, Gelatin Hydrogels Formed by Orthogonal Thiol-Norbornene Photochemistry for Cell Encapsulation. Biomater Sci, 2:1063–72. https://doi.org/10.1039/c4bm00070f
32. Chen YC, Lin RZ, Qi H, et al., 2012, Functional Human Vascular Network Generated in Photocrosslinkable Gelatin Methacrylate Hydrogels. Adv Funct Mater, 22:2027–39. https://doi.org/10.1002/adfm.201101662
33. Sun X, Lang Q, Zhang H, et al., 2017, Electrospun Photocrosslinkable Hydrogel Fibrous Scaffolds for Rapid In Vivo Vascularized Skin Flap Regeneration. Adv Funct Mater, 27:1604617. https://doi.org/10.1002/adfm.201604617
34. Zhao X, Lang Q, Yildirimer L, et al., 2016, Photocrosslinkable Gelatin Hydrogel for Epidermal Tissue Engineering. PLoS One, 5:108–18.
35. Zhao X, Sun X, Yildirimer L, et al., 2017, Cell Infiltrative Hydrogel Fibrous Scaffolds for Accelerated Wound Healing. Acta Biomater, 49:66–77. https://doi.org/10.1016/j.actbio.2016.11.017
36. Pellá MC, Lima-Tenório MK, Tenório-Neto ET, et al., 2018, Chitosan-based Hydrogels: From Preparation to Biomedical Applications. Carbohydr Polym, 196:233–45. https://doi.org/10.1016/j.carbpol.2018.05.033
37. He M, Han B, Jiang Z, et al., 2017, Synthesis of a Chitosan based Photo-Sensitive Hydrogel and its Biocompatibility and Biodegradability. Carbohydr Polym, 166:228–35. https://doi.org/10.1016/j.carbpol.2017.02.072
38. Pei M, Mao J, Xu W, et al., 2019, Photocrosslinkable Chitosan Hydrogels and their Biomedical Applications. J Polym Sci, 57:1862–71.
39. Elizalde-Peña E, Flores-Ramirez N, Luna-Barcenas G, et al., 2007, Synthesis and Characterization of Chitosan-g-glycidyl Methacrylate with Methyl Methacrylate. Eur Polym J, 43:3963–9. https://doi.org/10.1016/j.eurpolymj.2007.06.004
40. Lin CC, Raza A, Shih HJ, 2011, PEG Hydrogels Formed by Thiol-ene Photo-Click Chemistry and their Effect on the Formation and Recovery of Insulin-Secreting Cell Spheroids. Biomaterials, 32:9685–95. https://doi.org/10.1016/j.biomaterials.2011.08.083
41. Hockaday L, Kang K, Colangelo N, et al., 2012, Rapid 3D Printing of Anatomically Accurate and Mechanically Heterogeneous Aortic Valve Hydrogel Scaffolds. Biofabricatio, 4:035005. https://doi.org/10.1088/1758-5082/4/3/035005
42. Lim WS, Chen K, Chong TW, et al., 2018, A Bilayer Swellable Drug-eluting Ureteric Stent: Localized Drug Delivery to Treat Urothelial Diseases. Biomaterials, 165:25–38. https://doi.org/10.1016/j.biomaterials.2018.02.035
43. Shin H, Olsen BD, Khademhosseini A, 2012, The Mechanical Properties and Cytotoxicity of Cell-laden Double-network Hydrogels Based on Photocrosslinkable Gelatin and Gellan Gum Biomacromolecules. Biomaterials, 33:3143–52. https://doi.org/10.1016/j.biomaterials.2011.12.050
44. Kaur M, Srivastava A, 2002, Photopolymerization: A review. J Macromol Sci Part C Polym Rev, 42:481–512.
45. Yan Q, Dong H, Su J, et al., 2018, A Review of 3D Printing Technology for Medical Applications. Engineering, 4:729–42.
46. Colosi C, Shin SR, Manoharan V, et al., 2016, Microfluidic Bioprinting of Heterogeneous 3D Tissue Constructs Using Low-viscosity Bioink. Adv Mater, 28:677–84. https://doi.org/10.1002/adma.201503310
47. Wadnap S, Krishnamoorthy S, Zhang Z, et al., 2019, Biofabrication of 3D Cell-encapsulated Tubular Constructs Using Dynamic Optical Projection Stereolithography. J Mater Sci Mater Med, 30:1–10. https://doi.org/10.1007/s10856-019-6239-5
48. Xu H, Liu Q, Casillas J, et al., 2020, Prediction of Cell Viability in Dynamic Optical Projection Stereolithography based Bioprinting Using Machine Learning. J Intell Manuf, 2020:1–11. https://doi.org/10.1007/s10845-020-01708-5
49. Zhang K, Feng Q, Xu J, et al., 2017, Self-assembled Injectable Nanocomposite Hydrogels Stabilized by Bisphosphonate-magnesium (Mg2+) Coordination Regulates the Differentiation of Encapsulated Stem Cells Via Dual Crosslinking. Adv Funct Mater, 27:1701642. https://doi.org/10.1002/adfm.201701642
50. Zuo Y, Liu X, Wei D, et al., 2015, Photo-cross-linkable Methacrylated Gelatin and Hydroxyapatite Hybrid Hydrogel for Modularly Engineering Biomimetic Osteon. 7:10386–94. https://doi.org/10.1021/acsami.5b01433
51. Shi D, Xu X, Ye Y, et al., 2016, Photo-cross-linked Scaffold with Kartogenin-encapsulated Nanoparticles for Cartilage Regeneration. ACS Nano, 10:1292–9. https://doi.org/10.1021/acsnano.5b06663.s001
52. de Windt TS, Vonk LA, Slaper-Cortenbach IC, et al., 2017, Allogeneic Mesenchymal Stem Cells Stimulate Cartilage Regeneration and are Safe for Single-stage Cartilage Repair in Humans Upon Mixture with Recycled Autologous Chondrons. Stem Cells, 35:256–64. https://doi.org/10.1002/stem.2475
53. Levato R, Webb WR, Otto IA, et al., 2017, The Bio in the Ink: Cartilage Regeneration with Bioprintable Hydrogels and Articular Cartilage-derived Progenitor Cells. Acta Biomater, 61:41–53. https://doi.org/10.1016/j.actbio.2017.08.005
54. Yan S, Wang T, Feng L, et al., 2014, Injectable In Situ Self-cross-linking Hydrogels Based on Poly (L-glutamic Acid) and Alginate for Cartilage Tissue Engineering. Biomacromolecules, 15:4495–508. https://doi.org/10.1021/bm501313t
55. Gao G, Schilling AF, Hubbell K, et al., 2015, Improved Properties of Bone and Cartilage Tissue from 3D Inkjet bioprinted Human Mesenchymal Stem Cells by Simultaneous Deposition and Photocrosslinking in PEG-GelMA. 37:2349–55. https://doi.org/10.1007/s10529-015-1921-2
56. Rao RR, Stegemann JP, 2013, Cell-based Approaches to the Engineering of Vascularized Bone Tissue. Cytotherapy, 15:1309–22. https://doi.org/10.1016/j.jcyt.2013.06.005
57. Peters EB, 2018, Endothelial Progenitor Cells for the Vascularization of Engineered Tissues. Tissue Eng Part B Rev, 24:1–24.
58. Zhai X, Ruan C, Ma Y, et al., 2018, 3D-bioprinted Osteoblast laden Nanocomposite Hydrogel Constructs with Induced Microenvironments Promote Cell Viability, Differentiation, and Osteogenesis Both In Vitro and In Vivo. Adv Sci (Weinh), 5:1700550. https://doi.org/10.1002/advs.201700550
59. Wenz A, Tjoeng I, Schneider I, et al., 2018, Improved Vasculogenesis and Bone Matrix Formation through Coculture of Endothelial Cells and Stem Cells in Tissue specific Methacryloyl Gelatin-Based Hydrogels. Biotechnol Bioeng, 115:2643–53. https://doi.org/10.1002/bit.26792
60. Doschak MR, Kucharski CM, Wright JE, et al., 2009, Improved Bone Delivery of Osteoprotegerin by Bisphosphonate Conjugation in a Rat Model of Osteoarthritis. Mol Pharm, 6:634–40. https://doi.org/10.1021/mp8002368
61. Park D, Park CW, Choi Y, et al., 2016, A Novel Small molecule PPI Inhibitor Targeting Integrin αvβ3-osteopontin Interface Blocks Bone Resorption In Vitro and Prevents Bone Loss in Mice. Biomaterials, 98:131–42. https://doi.org/10.1016/j.biomaterials.2016.05.007
62. Teno N, Masuya K, Ehara T, et al., 2008, Effect of Cathepsin K Inhibitors on Bone Resorption. J Med Chem, 51:5459–62.
63. Arrighi I, Mark S, Alvisi M, et al., 2009, Bone Healing Induced by Local Delivery of an Engineered Parathyroid Hormone Prodrug. Biomaterials, 30:1763–71. https://doi.org/10.1016/j.biomaterials.2008.12.023
64. Rodan GA, Martin TJ, 2000, Therapeutic Approaches to Bone Diseases. Science, 289:1508–14.
65. Zhang Gand Suggs L J J A d d r, 2007, Matrices and scaffolds for drug delivery in vascular tissue engineering. Adv Drug Deliv Rev, 59:360–73. https://doi.org/10.1016/j.addr.2009.08.002
66. Nomi M, Atala A, De Coppi P, et al., 2002, Principals of Neovascularization for Tissue Engineering. Mol Aspects Med, 23:463–83. https://doi.org/10.1016/s0098-2997(02)00008-0
67. An Y, Hubbell JA, 2000, Intraarterial Protein Delivery Via Intimally-adherent Bilayer Hydrogels. J Control Release, 64:205–15. https://doi.org/10.1016/s0168-3659(99)00143-1
68. Kim HD, Lee EA, An YH, et al., 2017, Chondroitin Sulfate based Biomineralizing Surface Hydrogels for Bone Tissue Engineering. ACS Appl Mater Interfaces, 9:21639–50. https://doi.org/10.1021/acsami.7b04114
69. Xin T, Mao J, Liu L, et al., 2020, Programmed Sustained Release of Recombinant Human Bone Morphogenetic Protein-2 and Inorganic ion Composite Hydrogel as Artificial Periosteum. ACS Appl Mater Interfaces, 12:6840–51. https://doi.org/10.1021/acsami.9b18496
70. Cui H, Zhu W, Nowicki M, et al., 2016, Hierarchical Fabrication of Engineered Vascularized Bone Biphasic Constructs Via Dual 3D Bioprinting: Integrating Regional Bioactive Factors into Architectural Design. Adv Healthc Mater, 5:2174–81. https://doi.org/10.1002/adhm.201600505
71. Boere KW, Visser J, Seyednejad H, et al., 2014, Covalent Attachment of a Three-dimensionally Printed Thermoplast to a Gelatin Hydrogel for Mechanically Enhanced Cartilage Constructs. Acta Biomater, 10:2602–11. https://doi.org/10.1016/j.actbio.2014.02.041
72. Dhawan A, Kennedy PM, Rizk EB, et al., 2019, Three dimensional Bioprinting for Bone and Cartilage Restoration in Orthopaedic Surgery. J Am Acad Orthop Surg, 27:e215–26. https://doi.org/10.5435/jaaos-d-17-00632
73. Duchi S, Onofrillo C, O’Connell CD, et al., 2017, Handheld Co-axial Bioprinting: Application to In Situ Surgical Cartilage Repair. Scientific Reports, 7:1–12. https://doi.org/10.1038/s41598-017-05699-x
74. Galarraga JH, Kwon MY, Burdick JA, 2019, 3D Bioprinting Via an In Situ Crosslinking Technique towards Engineering Cartilage Tissue. Sci Rep, 9:1–12. https://doi.org/10.1038/s41598-019-56117-3
75. Lam T, Dehne T, Krüger JP, et al., 2019, Photopolymerizable Gelatin and Hyaluronic Acid for Stereolithographic 3D Bioprinting of Tissue-engineered Cartilage. J Biomed Mater Res, 107:2649–57. https://doi.org/10.1002/jbm.b.34354
76. Abdollahiyan P, Oroojalian F, Mokhtarzadeh A, et al., 2020, Hydrogel-Based 3D Bioprinting for Bone and Cartilage Tissue Engineering. 15:2000095. https://doi.org/10.1002/biot.202000095
77. Askari M, Naniz MA, Kouhi M, et al., 2021, Recent Progress in Extrusion 3D Bioprinting of Hydrogel Biomaterials for Tissue Regeneration: A Comprehensive Review with Focus on Advanced Fabrication Techniques. Biomater Sci, 9:535–73. https://doi.org/10.1039/d0bm00973c
78. Cui X, Breitenkamp K, Finn M, et al., 2012, Direct Human Cartilage Repair Using Three-dimensional Bioprinting Technology. Tissue Eng Part A, 18:1304–12. https://doi.org/10.1089/ten.tea.2011.0543
79. Gao F, Xu Z, Liang Q, et al., 2019, Osteochondral Regeneration with 3D-Printed Biodegradable High-strength Supramolecular Polymer Reinforced-gelatin Hydrogel Scaffolds. Adv Sci., 6:1900867. https://doi.org/10.1002/advs.201900867
80. Genova T, Roato I, Carossa M, et al., 2020, Advances on Bone Substitutes Through 3D Bioprinting. Int J Mol Sci, 21:7012. https://doi.org/10.3390/ijms21197012
81. Qiao H., Tang TJ, 2018, Engineering 3D Approaches to Model the Dynamic Microenvironments of Cancer Bone Metastasis. Bone Res, 6:1–12. https://doi.org/10.1038/s41413-018-0008-9
82. Ozbolat IT, Peng W, Ozbolat VJ, 2016, Application Areas of 3D Bioprinting. Drug Discov Today, 21:1257–71. https://doi.org/10.1016/j.drudis.2016.04.006
83. Ozbolat IT, Hospodiuk MJ, 2016, Current Advances and Future Perspectives in Extrusion-based Bioprinting. Biomaterials, 76:321–43. https://doi.org/10.1016/j.biomaterials.2015.10.076
84. Miri AK, Nieto D, Iglesias L, et al., 2018, Microfluidics-Enabled Multimaterial Maskless Stereolithographic Bioprinting. Adv Mater, 30:e1800242. https://doi.org/10.1002/adma.201870201
85. Zhu W, Holmes B, Glazer RI, et al., 2016, 3D Printed Nanocomposite Matrix for the Study of Breast Cancer Bone Metastasis. Nanomedicine, 12:69–79. https://doi.org/10.1016/j.nano.2015.09.010
86. Zhou X, Zhu W, Nowicki M, et al., 2016, 3D Bioprinting a Cell-laden Bone Matrix for Breast Cancer Metastasis Study. ACS Appl Mater Interfaces, 8:30017–26. https://doi.org/10.1021/acsami.6b10673
87. Stichler S, Jungst T, Schamel M, et al., 2017, Thiol-ene Clickable Poly(glycidol) Hydrogels for Biofabrication. Ann Biomed Eng, 45:273–85. https://doi.org/10.1007/s10439-016-1633-3
88. Murphy SV, Atala A, 2014, 3D Bioprinting of Tissues and Organs. Nat Biotechnol, 32:773–85. https://doi.org/10.1038/nbt.2958
89. Baxter FR, Bowen CR, Turner IG, et al., 2010, Electrically Active Bioceramics: A Review of Interfacial Responses. Ann Biomed Eng, 38:2079–92. https://doi.org/10.1007/s10439-010-9977-6
90. Jiang L, Wang Y, Liu Z, et al., 2019, Three-dimensional Printing and Injectable Conductive Hydrogels for Tissue Engineering Application. Tissue Eng Part B Rev, 25:398–411. https://doi.org/10.1089/ten.teb.2019.0100
91. Wei K, Zhu M, Sun Y, et al., 2016, Robust Biopolymeric Supramolecular “Host Guest Macromer” Hydrogels Reinforced by In Situ Formed Multivalent Nanoclusters for Cartilage Regeneration. Macromolecules, 49:866–75. https://doi.org/10.1021/acs.macromol.5b02527.s001
92. Salzlechner C, Haghighi T, Huebscher I, et al., 2020, Adhesive Hydrogels for Maxillofacial Tissue Regeneration Using Minimally Invasive Procedures. Adv Healthc Mater, 9:1901134. https://doi.org/10.1002/adhm.201901134
93. Khare D, Basu B, Dubey AK, 2002, Electrical Stimulation and Piezoelectric Biomaterials for Bone Tissue Engineering Applications. Biomaterials, 258:120280.
94. Wang Q, Chen X, Zhu J, et al., 2008, Porous Li-Na-K Niobate Bone-substitute Ceramics: Microstructure and Piezoelectric Properties. Mater Lettt, 62:3506–8. https://doi.org/10.1016/j.matlet.2008.03.024
95. Zhang X, Zhang C, Lin Y, et al., 2016, Nanocomposite Membranes Enhance Bone Regeneration Through Restoring Physiological Electric Microenvironment. ACS Nano, 10:7279–86. https://doi.org/10.1021/acsnano.6b02247.s001
96. Mestres G, Perez RA, D’Elía NL, et al., 2019, Advantages of Microfluidic Systems for Studying Cell-biomaterial Interactions Focus on Bone Regeneration Applications. Biomed Phys Eng Express, 5:032001. https://doi.org/10.1088/2057-1976/ab1033
97. Kirillova A, Maxson R, Stoychev G, et al., 2017, 4D Biofabrication Using Shape-morphing Hydrogels. Adv Mater, 29:1703443. https://doi.org/10.1002/adma.201703443