3D Printed Zn-doped Mesoporous Silica-incorporated Poly-L-lactic Acid Scaffolds for Bone Repair
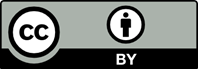
Poly-L-lactic acid (PLLA) lacks osteogenic activity, which limits its application in bone repair. Zinc (Zn) is widely applied to strengthen the biological properties of polymers due to its excellent osteogenic activity. In the present study, Zndoped mesoporous silica (Zn-MS) particles were synthesized by one-pot hydrothermal method. Then, the particles were induced into PLLA scaffolds prepared by selective laser sintering technique, aiming to improve their osteogenic activity. Our results showed that the synthesized particles possessed rosette-like morphology and uniform mesoporous structure, and the composite scaffold displayed the sustained release of Zn ion in a low concentration range, which was attributed to the shield effect of the PLLA matrix and the strong bonding interaction of Si-O-Zn. The scaffold could evidently promote osteogenesis differentiation of mouse bone marrow mesenchymal stem cells by upregulating their osteogenesis-related gene expression. Besides, Zn-MS particles could significantly increase the compressive strength of the PLLA scaffold because of their rosettelike morphology and mesoporous structure, which can form micromechanical interlocking with the PLLA matrix. The Zn-MS particles possess great potential to improve various polymer scaffold properties due to their advantageous morphology and physicochemical properties.
1. Wang G, Qian G, Zan J, et al., 2020, A Co-dispersion Nanosystem of Graphene Oxide@ Silicon-doped Hydroxyapatite to Improve Scaffold Properties. Mater Design, 2020:109399. https://doi.org/10.1016/j.matdes.2020.109399
2. Shuai C, Yu L, Feng P, et al., 2020, Organic Montmorillonite Produced an Interlayer Locking Effect in a Polymer Scaffold to Enhance Interfacial Bonding. Mater Chem Front, 4:2398–408. https://doi.org/10.1039/d0qm00254b
3. Shuai C, Yang W, Feng P, et al., 2020, Accelerated Degradation of HAP/PLLA Bone Scaffold by PGA Blending Facilitates Bioactivity and Osteoconductivity. Bioact Mater, 6:490–502. https://doi.org/10.1016/j.bioactmat.2020.09.001
4. Qi F, Wang C, Peng S, et al., 2021, A Co-dispersed Nanosystem from Strontium-Anchored Reduced Graphene Oxide to Enhance Bioactivity and Mechanical Property in Polymer Scaffolds. Mater Chem Front, 2021:958. https://doi.org/10.1039/d0qm00958j
5. King JC, Shames DM, Woodhouse LR, 2020, Zinc Homeostasis in Humans. J Nutr, 130:1360S–6S.
6. Hadley KB, Newman SM, Hunt JR, 2010, Dietary Zinc Reduces Osteoclast Resorption Activities and Increases Markers of Osteoblast Differentiation, Matrix Maturation, and Mineralization in the Long Bones of Growing Rats. J Nutr Biochem, 21:297–303. https://doi.org/10.1016/j.jnutbio.2009.01.002
7. Vojtěch D, Kubásek J, Šerák J, et al., 2011, Mechanical and Corrosion Properties of Newly Developed Biodegradable Zn based Alloys for Bone Fixation. Acta Biomater, 7:3515–22. https://doi.org/10.1016/j.actbio.2011.05.008
8. Bejarano J, Boccaccini AR, Covarrubias C, et al., 2020, Effect of Cu-and Zn-Doped Bioactive Glasses on the In Vitro Bioactivity, Mechanical and Degradation Behavior of Biodegradable PDLLA Scaffolds. Materials, 13:2908. https://doi.org/10.3390/ma13132908
9. Harikrishnan P, Sivasamy A, 2020, Preparation, Characterization of Electrospun Polycaprolactone-nano Zinc Oxide Composite Scaffolds for Osteogenic Applications. Nanostruct Nanoobjects, 23:100518. https://doi.org/10.1016/j.nanoso.2020.100518
10. Shuai C, Wang C, Qi F, et al., 2020, Enhanced Crystallinity and Antibacterial of PHBV Scaffolds Incorporated with Zinc Oxide. J Nanomater, 2020:6014816.
11. Xia Y, Fan X, Yang H, et al., 2020, ZnO/Nanocarbons modified Fibrous Scaffolds for Stem Cell-based Osteogenic Differentiation. Small, 16:2003010. https://doi.org/10.1002/smll.202003010
12. Wu J, Wang L, He J, et al., 2012, In Vitro Cytotoxicity of Cu2+, Zn2+, Ag+ and their Mixtures on Primary Human Endometrial Epithelial Cells. Contraception, 85:509–18. https://doi.org/10.1016/j.contraception.2011.09.016
13. Bai X, Lin C, Wang Y, et al., 2020, Preparation of Zn Doped Mesoporous Silica Nanoparticles (Zn-MSNs) for the Improvement of Mechanical and Antibacterial Properties of Dental Resin Composites. Dent Mater, 36:794–807. https://doi.org/10.1016/j.dental.2020.03.026
14. Szewczyk A, Skwira A, Ginter M, et al., 2021, Microwave assisted Fabrication of Mesoporous Silica-calcium Phosphate Composites for Dental Application. Polymers, 13:53. https://doi.org/10.3390/polym13010053
15. Li X, Wang X, Qian G, et al., 2019, Synergistical Chemotherapy and Cancer Immunotherapy Using Dual Drug-delivering and Immunopotentiating Mesoporous Silica. Appl Mater Today, 16:102–11. https://doi.org/10.1016/j.apmt.2019.05.006
16. Li X, Shenashen MA, Wang X, et al., 2018, Mesoporous Caged-γ-AlOOH-Double-stranded RNA Analog Complexes for Cancer Immunotherapy. Adv Biosyst, 2:1700114. https://doi.org/10.1002/adbi.201700114
17. Qian G, Wang X, Li X, et al., 2019, An Immuno-potentiating Vehicle Made of Mesoporous Silica-zinc Oxide Microrosettes with Enhanced Doxorubicin Loading for Combined Chemoimmunotherapy. Chem Commun, 55:961–4. https://doi.org/10.1039/c8cc09044k
18. Wang X, Li X, Ito A, et al., 2017, Biodegradable Metal Ion doped Mesoporous Silica Nanospheres Stimulate Anticancer Th1 Immune Response In Vivo. ACS Appl Mater Interf, 9:43538–44. https://doi.org/10.1021/acsami.7b16118.s001
19. Shuai C, Li S, Peng S, et al., 2020, Hydrolytic expansion induces corrosion propagation for increased fe biodegradation. Int J Bioprint, 6:248. https://doi.org/10.18063/ijb.v6i1.248
20. Qian G, Fan P, He F, et al., 2019, Novel Strategy to Accelerate Bone Regeneration of Calcium Phosphate Cement by Incorporating 3D Plotted Poly (Lactic-co-glycolic Acid) Network and Bioactive Wollastonite. Adv Healthc Mater, 8:18013. https://doi.org/10.1002/adhm.201801325
21. Qian G, Lu T, Zhang J, et al., 2020, Promoting Bone Regeneration of Calcium Phosphate Cement by Addition of PLGA Microspheres and Zinc Silicate Via Synergistic Effect of In-Situ Pore Generation, Bioactive ion Stimulation and Macrophage Immunomodulation. Appl Mater Today, 19:100615. https://doi.org/10.1016/j.apmt.2020.100615
22. Yuan S, Chua CK, Zhou K, 2019, 3D-printed Mechanical Metamaterials with High Energy Absorption. Adv Mater Technol, 4:1800419. https://doi.org/10.1002/admt.201800419
23. Ng WL, Lee J M, Zhou M, et al., 2020, Vat Polymerization based Bioprinting-Process, Materials, Applications and Regulatory Challenges. Biofabrication, 12:022001. https://doi.org/10.1088/1758-5090/ab6034
24. Ng WL, Chua CK, Shen YF, 2019, Print me an Organ! Why we are not there yet. Prog Polym Sci, 97:101145. https://doi.org/10.1016/j.progpolymsci.2019.101145
25. Alabort E, Barba D, Reed RC, 2019, Design of Metallic Bone by Additive Manufacturing. Script Mater, 164:110–14. https://doi.org/10.1016/j.scriptamat.2019.01.022
26. Shuai C, Li Y, Yang W, et al., 2020, Graphene Oxide Induces Ester Bonds Hydrolysis of Poly-l-lactic Acid Scaffold to Accelerate Degradation. Int J Bioprint, 6:249. https://doi.org/10.18063/ijb.v6i1.249
27. Liu J, Wei X, Wang X, et al., 2011, High-yield Synthesis of Ultrathin Silica-Based Nanosheets and their Superior Catalytic Activity in H2O2 Decomposition. Chem Commun, 47:6135–7. https://doi.org/10.1039/c1cc10280j
28. Hallab NJ, Bundy J, O’Connor K, et al., 2001, Evaluation of Metallic and Polymeric Biomaterial Surface Energy and Surface Roughness Characteristics for Directed Cell Adhesion. Tissue Eng, 7:55–71. https://doi.org/10.1089/107632700300003297
29. Ranjbar HA, Nourany M, Mollavali M, et al., 2020, Stimuli-responsive Polyurethane Bionanocomposites of Poly (ethylene glycol)/poly (ε-caprolactone) and [poly (ε-caprolactone)-grafted-] Cellulose Nanocrystals. Polym Adv Technol, 32:5062. https://doi.org/10.1002/pat.5062
30. Alnoor O, Laoui T, Ibrahim A, et al., 2020, Graphene Oxide based Membranes for Water Purification Applications: Effect of Plasma Treatment on the Adhesion and Stability of the Synthesized Membranes. Membranes, 10:292. https://doi.org/10.3390/membranes10100292
31. Xin S, Gregory CA, Alge DL, 2020, Interplay between Degradability and Integrin Signaling on Mesenchymal Stem Cell Function within Poly (Ethylene Glycol) Based Microporous Annealed Particle Hydrogels. Acta Biomater, 101:227–36. https://doi.org/10.1016/j.actbio.2019.11.009
32. Yang L, Jiang Z, Zhou L, et al., 2017, Hydrophilic Cell derived Extracellular Matrix as a Niche to Promote Adhesion and Differentiation of Neural Progenitor Cells. RSC Adv, 7:45587–94. https://doi.org/10.1039/c7ra08273h
33. Prochor P, Frossard L, Sajewicz E, 2020, Effect of the Material’s Stiffness on Stress-shielding in Osseointegrated Implants for Bone-anchored Prostheses: A Numerical Analysis and Initial Benchmark Data. Acta Bioeng Biomech, 22:1–24. https://doi.org/10.37190/abb-01543-2020-02
34. Shuai C, Wang B, Bin S, et al., 2020, TiO2-induced In Situ Reaction in Graphene Oxide-reinforced AZ61 Biocomposites to Enhance the Interfacial Bonding. ACS Appl Mater Interf, 12:23464–73. https://doi.org/10.1021/acsami.0c04020
35. Shuai C, Liu G, Yang Y, et al., 2020, A Strawberry-like Ag-decorated Barium Titanate Enhances Piezoelectric and Antibacterial Activities of Polymer Scaffold. Nano Energy, 2020:104825. https://doi.org/10.1016/j.nanoen.2020.104825
36. Murugan R, Ramakrishna S, 2005, Development of Nanocomposites for Bone Grafting. Compos Sci Technol, 65:2385–406.
37. Samuel SP, Li S, Mukherjee I, et al., 2009, Mechanical Properties of Experimental Dental Composites Containing a Combination of Mesoporous and Nonporous Spherical Silica as Fillers. Dent Mater, 25:296–301. https://doi.org/10.1016/j.dental.2008.07.012
38. Shuai C, Li S, Yang W, et al., 2020, MnO2 Catalysis of Oxygen Reduction to Accelerate the Degradation of Fe-C Composites for Biomedical Applications. Corros Sci, 2020:108679. https://doi.org/10.1016/j.corsci.2020.108679
39. Shuai C, He C, Qian G, et al., 2020, Mechanically Driving Supersaturated Fe-Mg Solid Solution for Bone Implant: Preparation, Solubility and Degradation. Compos Part B Eng, 2020:108564. https://doi.org/10.1016/j.compositesb.2020.108564
40. Zou Z, Liu W, Cao L, et al., 2020, Advances in the Occurrence and Biotherapy of Osteoporosis. Biochem Soc Trans, 48:1623–36.
41. Heras C, Sanchez-Salcedo S, Lozano D, et al., 2019, Osteostatin Potentiates the Bioactivity of Mesoporous Glass Scaffolds Containing Zn2+ Ions in Human Mesenchymal Stem Cells. Acta Biomater, 89:359–371. https://doi.org/10.1016/j.actbio.2019.03.033
42. Wang B, Yang M, Liu L, et al., 2019. Osteogenic Potential of Zn2+-passivated Carbon dots for bone Regeneration In Vivo. Biomater Sci, 7:5414–23. https://doi.org/10.1039/c9bm01181a
43. Kao YY, Chen YC, Cheng TJ, et al., 2012, Zinc Oxide Nanoparticles Interfere with Zinc Ion Homeostasis to Cause Cytotoxicity. Toxicol Sci, 125:462–72. https://doi.org/10.1093/toxsci/kfr319
44. Jin Z, Wu R, Shen J, et al., 2018, Nonstoichiometric Wollastonite Bioceramic Scaffolds with Core-shell Pore Struts and Adjustable Mechanical and Biodegradable Properties. J Mech Behav Biomed Mater, 88:140–9. https://doi.org/10.1016/j.jmbbm.2018.08.018
45. Zeng J, Xu L, Luo X, et al., 2021, A Novel Design of SiH/CeO2 (111) van der Waals Type-II Heterojunction for Water Splitting. Phys Chem Chem Phys, 23:2812–8. https://doi.org/10.1039/d0cp05238h
46. Yu Y, Liu K, Wen Z, et al., 2020, Double-edged Effects and Mechanisms of Zn2+ Microenvironments on Osteogenic Activity of BMSCs: Osteogenic Differentiation or Apoptosis. RSC Adv, 10:14915–27. https://doi.org/10.1039/d0ra01465f
47. Zou A, Liang H, Jiao C, et al., 2020, Fabrication and Properties of CaSiO3/Sr3(PO4)2 Composite Scaffold Based on Extrusion Deposition. Ceram Int, 47:4783–92. https://doi.org/10.1016/j.ceramint.2020.10.048
48. Han P, Wu C, Xiao Y, 2013, The Effect of Silicate Ions on Proliferation, Osteogenic Differentiation and Cell Signalling Pathways (WNT and SHH) of Bone Marrow Stromal Cells. Biomater Sci, 1:379–92. https://doi.org/10.1039/c2bm00108j.