Using Plant Proteins to Develop Composite Scaffolds for Cell Culture Applications
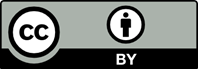
Electrohydrodynamic printing (EHDP) is capable of fabricating scaffolds that consist of micro/nano scale orientated fibers for three-dimensional (3D) cell culture models and drug screening applications. One of the major hurdles that limit the widespread application of EHDP is the lack of diverse biomaterial inks with appropriate printability and desired mechanical and biological properties. In this work, we blended plant proteins with synthetic biopolymer poly(ε-caprolactone) (PCL) to develop composite biomaterial inks, such as PCL/gliadin and PCL/zein for scaffold fabrication through EHDP. The tensile test results showed that the composite materials with a relatively small amount of plant protein portions, such as PCL/ gliadin-10 and PCL/zein-10, can significantly improve tensile properties of the fabricated scaffolds such as Young’s modulus and yield stress. These scaffolds were further evaluated by culturing mouse embryonic fibroblasts (NIH/3T3) cells and proven to enhance cell adhesion and proliferation, apart from temporary inhibition effects for PCL/gliadin-20 scaffold at the initial growth stage. After these plant protein nanoparticles were gradually released into culture medium, the generated nanoporous structures on the scaffold fiber surfaces became favorable for cellular attachment, migration, and proliferation. As competent candidates that regulate cell behaviors in 3D microenvironment, such composite scaffolds manifest a great potential in drug screening and 3D in vitro model development.
1. Pampaloni F, Reynaud E Gand Stelzer E H, 2007, The third dimension bridges the gap between cell culture and live tissue. Nature reviews Molecular cell biology, 8(10): 839-845.
2. Walpita Dand Hay E, 2002, Studying actin-dependent processes in tissue culture. Nature reviews Molecular cell biology, 3(2): 137-141.
3. Gudjonsson T, Rønnov-Jessen L, Villadsen R, et al., 2002, Normal and tumor-derived myoepithelial cells differ in their ability to interact with luminal breast epithelial cells for polarity and basement membrane deposition. Journal of cell science, 115(1): 39-50.
4. Dhiman H K, Ray A Rand Panda A K, 2005, Three-dimensional chitosan scaffold-based MCF-7 cell culture for the determination of the cytotoxicity of tamoxifen. Biomaterials, 26(9): 979-986. 10.1016/j.biomaterials.2004.04.012
5. Chia H Nand Wu B M, 2015, Recent advances in 3D printing of biomaterials. J Biol Eng, 9: 4. 10.1186/s13036-015-0001-4
6. Zhang B, He J, Li X, et al., 2016, Micro/nanoscale electrohydrodynamic printing: from 2D to 3D. Nanoscale, 8(34): 15376-15388. 10.1039/c6nr04106j
7. Siqueira G, Kokkinis D, Libanori R, et al., 2017, Cellulose Nanocrystal Inks for 3D Printing of Textured Cellular Architectures. Advanced Functional Materials, 27(12). 10.1002/adfm.201604619
8. Chung J H Y, Naficy S, Yue Z, et al., 2013, Bio-ink properties and printability for extrusion printing living cells. Biomater Sci, 1(7): 763-773. 10.1039/c3bm00012e
9. Sun J, Vijayavenkataraman Sand Liu H, 2017, An Overview of Scaffold Design and Fabrication Technology for Engineered Knee Meniscus. Materials (Basel), 10(1). 10.3390/ma10010029
10. Onses M S, Sutanto E, Ferreira P M, et al., 2015, Mechanisms, Capabilities, and Applications of High-Resolution Electrohydrodynamic Jet Printing. Small, 11(34): 4237-4266. 10.1002/smll.201500593
11. Jing L, Wang X, Liu H, et al., 2018, Zein Increases the Cytoaffinity and Biodegradability of Scaffolds 3D-Printed with Zein and Poly(epsilon-caprolactone) Composite Ink. ACS Appl Mater Interfaces, 10(22): 18551-18559. 10.1021/acsami.8b04344
12. Woodruff M Aand Hutmacher D W, 2010, The return of a forgotten polymer—Polycaprolactone in the 21st century. Progress in Polymer Science, 35(10): 1217-1256. 10.1016/j.progpolymsci.2010.04.002
13. Reddy Nand Yang Y, 2011, Potential of plant proteins for medical applications. Trends Biotechnol, 29(10): 490-498. 10.1016/j.tibtech.2011.05.003
14. Paliwal Rand Palakurthi S, 2014, Zein in controlled drug delivery and tissue engineering. J Control Release, 189: 108-122. 10.1016/j.jconrel.2014.06.036
15. Wang H J, Gong S J, Lin Z X, et al., 2007, In vivo biocompatibility and mechanical properties of porous zein scaffolds. Biomaterials, 28(27): 3952-3964. 10.1016/j.biomaterials.2007.05.017
16. He J, Xu F, Cao Y, et al., 2016, Towards microscale electrohydrodynamic three-dimensional printing. Journal of Physics D: Applied Physics, 49(5). 10.1088/0022-3727/49/5/055504
17. Wan Z L, Guo Jand Yang X Q, 2015, Plant protein-based delivery systems for bioactive ingredients in foods. Food Funct, 6(9): 2876-2889. 10.1039/c5fo00050e
18. Koning F, 2015, Adverse Effects of Wheat Gluten. Ann Nutr Metab, 67 Suppl 2: 8-14. 10.1159/000440989
19. Liu H, Vijayavenkataraman S, Wang D, et al., 2017, Influence of electrohydrodynamic jetting parameters on the morphology of PCL scaffolds. International Journal of Bioprinting, 3(1). 10.18063/ijb.2017.01.009
20. Jie S, Hong G S, Rahman M, et al., Feature extraction and selection in tool condition monitoring system, Australian Joint Conference on Artificial Intelligence, 2002. Springer, 487-497.
21. Sun J, Hong G S, Rahman M, et al., 2005, Improved performance evaluation of tool condition identification by manufacturing loss consideration. International Journal of Production Research, 43(6): 1185-1204. 10.1080/00207540412331299701
22. Urade R, Sato Nand Sugiyama M, 2018, Gliadins from wheat grain: an overview, from primary structure to nanostructures of aggregates. Biophys Rev, 10(2): 435-443. 10.1007/s12551-017-0367-2
23. Castro A G B, Diba M, Kersten M, et al., 2018, Development of a PCL-silica nanoparticles composite membrane for Guided Bone Regeneration. Mater Sci Eng C Mater Biol Appl, 85: 154-161. 10.1016/j.msec.2017.12.023
24. Meshel A S, Wei Q, Adelstein R S, et al., 2005, Basic mechanism of three-dimensional collagen fibre transport by fibroblasts. Nat Cell Biol, 7(2): 157-164. 10.1038/ncb1216