Rational Design of a Triple-Layered Coaxial Extruder System: in silico and in vitro Evaluations Directed Toward Optimizing Cell Viability
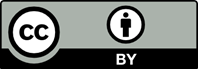
Biofabrication is a rapidly evolving field whose main goal is the manufacturing of three-dimensional (3D) cell-laden constructs that closely mimic tissues and organs. Despite recent advances on materials and techniques directed toward the achievement of this goal, several aspects such as tissue vascularization and prolonged cell functionality are limiting bench-tobedside translation. Extrusion-based 3D bioprinting has been devised as a promising biofabrication technology to overcome these limitations, due to its versatility and wide availability. Here, we report the development of a triple-layered coaxial nozzle for use in the biomanufacturing of vascular networks and vessels. The design of the coaxial nozzle was first optimized toward guaranteeing high cell viability upon extrusion. This was done with the aid of in silico evaluations and their subsequent experimental validation by investigating the bioprinting of an alginate-based bioink. Results confirmed that the values for pressure distribution predicted by in silico experiments resulted in cell viabilities above 70% and further demonstrated the effect of layer thickness and extrusion pressure on cell viability. Our work paves the way for the rational design of multi-layered coaxial extrusion systems to be used in biofabrication approaches to replicate the very complex structures found in native organs and tissues.
1. Murphy SV, Atala A, 2014, 3D Bioprinting of Tissues and Organs. Nat Biotechnol, 32:773–85.
2. Pati F, Gantelius J, Svahn HA, 2016, 3D Bioprinting of Tissue/Organ Models. Angew Chem, 55:4650–65.
3. Heinrich MA, Liu W, Jimenez A, et al., 2019, 3D Bioprinting: From Benches to Translational Applications. Small, 15:1–47.
4. Jiang T, Munguia-Lopez JG, Flores-Torres S, et al., 2019, Extrusion Bioprinting of Soft Materials: An Emerging Technique for Biological Model Fabrication. Appl Phys Rev, 6:11310.
5. Paxton N, Smolan W, Böck T, et al., 2017, Proposal to Assess Printability of Bioinks for Extrusion-based Bioprinting and Evaluation of Rheological Properties Governing Bioprintability. Biofabrication, 9:4.
6. Ozbolat IT, Hospodiuk M, 2016, Current Advances and Future Perspectives in Extrusion-based Bioprinting. Biomaterials, 76:321–43.
7. Hölzl K, Lin S, Tytgat L, et al., 2016, Bioink Properties before, during and after 3D Bioprinting. Biofabrication, 8:32002.
8. Jungst T, Smolan W, Schacht K, et al., 2016, Strategies and Molecular Design Criteria for 3D Printable Hydrogels. Chem Rev, 116:1496–539.
9. Williams D, Thayer P, Martinez H, et al., 2018, A Perspective on the Physical, Mechanical and Biological Specifications of Bioinks and the Development of Functional Tissues in 3D Bioprinting. Bioprinting, 9:19–36.
10. Hospodiuk M, Dey M, Sosnoski D, et al., 2017, The Bioink: A Comprehensive Review on Bioprintable Materials. Biotechnol Adv, 35:217–39.
11. Hinton TJ, Jallerat Q, Palchesko RN, et al., 2015, Three-dimensional Printing of Complex Biological Structures by Freeform Reversible Embedding of Suspended Hydrogels. Sci Adv, 1:9.
12. Kelly BE, Bhattacharya I, Heidari H, et al., 2019, Volumetric Additive Manufacturing via Tomographic Reconstruction. Science, 363:1075–9.
13. Li J, Wu C, Chu PK, Gelinsky M, 2020, 3D Printing of Hydrogels: Rational Design Strategies and Emerging Biomedical Applications. Mater Sci Eng R Rep, 140:100543.
14. Lee A, Hudson AR, Shiwarski DJ, et al., 2019, 3D Bioprinting of Collagen to Rebuild Components of the Human Heart.Science, 365:482–7.
15. Bernal PN, Delrot P, Loterie D, et al., 2019, Volumetric Bioprinting of Complex Living-Tissue Constructs within Seconds. Adv Mater, 31:42.
16. Levato R, Jungst T, Scheuring RG, et al., 2020, From Shape to Function: The Next Step in Bioprinting. Adv Mater, 2020:1906423.
17. Moroni L, Burdick JA, Highley C, et al., 2018, Biofabrication Strategies for 3D In Vitro Models and Regenerative Medicine. Nat Rev Mater, 3:21–37.
18. Ravnic DJ, Leberfinger AN, Koduru SV, et al., 2017, Transplantation of Bioprinted Tissues and Organs: Technical and Clinical Challenges and Future Perspectives. Ann Surg, 266:48–58.
19. Ke D, Murphy SV, 2019, Current Challenges of Bioprinted Tissues Toward Clinical Translation. Tissue Eng Part B Rev, 25:1–13.
20. Kang HW, Lee SJ, Ko IK, et al., 2016, A 3D Bioprinting System to Produce Human-scale Tissue Constructs with Structural Integrity. Nat Biotechnol, 34:312–9.
21. Distler T, Ruther F, Boccaccini AR, et al., 2019, Development of 3D Biofabricated Cell Laden Hydrogel Vessels and a Low-Cost Desktop Printed Perfusion Chamber for In Vitro Vessel Maturation. Macromol Biosci, 19:9.
22. Jia W, Gungor-Ozkerim PS, Zhang YS, et al., 2016, Direct 3D Bioprinting of Perfusable Vascular Constructs Using a Blend Bioink. Biomaterials, 106:58–68.
23. Gao G, Park JY, Kim BS, et al., 2018, Coaxial Cell Printing of Freestanding, Perfusable, and Functional In Vitro Vascular Models for Recapitulation of Native Vascular Endothelium Pathophysiology. Adv Healthc Mater, 7:1–12.
24. Dranseikiene D, Schrüfer S, Schubert DW, et al., 2020, Cell laden Alginate Dialdehyde Gelatin Hydrogels Formed in 3D Printed Sacrificial Gel. J Mater Sci Mater Med, 31:3–7.
25. Blaeser A, Campos DF, Puster U, et al., 2016, Controlling Shear Stress in 3D Bioprinting is a Key Factor to Balance Printing Resolution and Stem Cell Integrity. Adv Healthc Mater, 5:326–33.
26. Nair K, Gandhi M, Khalil S, et al., 2009, Characterization of Cell Viability during Bioprinting Processes. Biotechnol J, 4:1168–77.
27. Yu Y, Zhang Y, Martin JA, et al., 2013, Evaluation of Cell Viability and Functionality in Vessel-like Bioprintable Cellladen Tubular Channels. J Biomech Eng, 135:1–9.
28. Widmaier EP, Raaff H, Strang KT, 2014, Vander’s Human Physiology. 13th ed. McGraw Hill, New York.
29. Curley CJ, Dolan EB, Otten M, et al., 2019, An Injectable Alginate/Extra Cellular Matrix (ECM) Hydrogel Towards Acellular Treatment of Heart Failure. Drug Deliv Transl Res, 9:1–13.
30. Wei LN, Chee KC, Yu FS, 2019, Print Me an Organ! Why We Are Not There Yet. Prog Polym Sci, 97:1–45.
31. Schoneberg J, De Lorenzi F, Theek B, et al., 2018, Engineering Biofunctional In Vitro Vessel Models Using a Multilayer Bioprinting Technique. Sci Rep, 8:1–13.