Investigating the Effect of Carbon Nanomaterials Reinforcing Poly(ε-Caprolactone) Printed Scaffolds for Bone Repair Applications
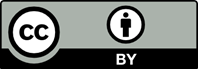
Scaffolds, three-dimensional (3D) substrates providing appropriate mechanical support and biological environments for new tissue formation, are the most common approaches in tissue engineering. To improve scaffold properties such as mechanical properties, surface characteristics, biocompatibility and biodegradability, different types of fillers have been used reinforcing biocompatible and biodegradable polymers. This paper investigates and compares the mechanical and biological behaviors of 3D printed poly(ε-caprolactone) scaffolds reinforced with graphene (G) and graphene oxide (GO) at different concentrations. Results show that contrary to G which improves mechanical properties and enhances cell attachment and proliferation, GO seems to show some cytotoxicity, particular at high contents.
1. Langer R, Vacanti JP, 1993, Tissue Engineering. Science, 260:920–6.
2. Bártolo PJ, Almeida HA, Rezende RA, et al., 2008, Advanced Processes to Fabricate Scaffolds for Tissue Engineering. In: Virtual Prototyping and Bio Manufacturing in Medical Applications. Springer, Berlin, pp. 149–70. DOI: 10.1007/978-0-387-68831-2_8.
3. Feng P, Jia J, Peng S, et al., 2020, Graphene Oxide-driven Interfacial Coupling in Laser 3D Printed PEEK/PVA Scaffolds for Bone Regeneration. Virtual Phys Prototyp, 2020:1–16. DOI: 10.1080/17452759.2020.1719457.
4. Lee JM, Ng WL, Yeong WY. 2019, Resolution and Shape in Bioprinting: Strategizing Towards Complex Tissue and Organ Printing. Appl Phys Rev, 6:011307. DOI: 10.1063/1.5053909.
5. Shuai C, Yang W, Peng S, et al., 2018, Physical Stimulations and Their Osteogenesis-Inducing Mechanisms. 2018:1–4. DOI: 10.18063/ijb.v4i2.138.
6. Lai Y, Li Y, Cao H, et al., 2019, Osteogenic Magnesium Incorporated into PLGA/TCP Porous Scaffold by 3D Printing for Repairing Challenging Bone Defect. Biomaterials, 197:207–19. DOI: 10.1016/j.biomaterials.2019.01.013.
7. Paris JL, Lafuente-Gómez N, Cabañas MV, et al., 2019, Fabrication of a Nanoparticle-containing 3D Porous Bone Scaffold with Proangiogenic and Antibacterial Properties. Acta Biomater, 86:441–9. DOI: 10.1016/j.actbio.2019.01.013.
8. Liu D, Nie W, Li D, et al., 2019, 3D Printed PCL/SrHA Scaffold for Enhanced Bone Regeneration. Chem Eng J, 362:269–79. DOI: 10.1016/j.cej.2019.01.015.
9. Harun WS, Kamariah MS, Muhamad N, et al., 2018, A Review of Powder Additive Manufacturing Processes for Metallic Biomaterials. Powder Technol, 327:128–51. DOI: 10.1016/j.powtec.2017.12.058.
10. Wu H, Fahy WP, Kim S, et al., 2020, Recent Developments in Polymers/polymer Nanocomposites for Additive Manufacturing. Prog Mater Sci, 111:100638. DOI: 10.1016/j.pmatsci.2020.100638.
11. An J, Teoh JE, Suntornnond R, et al., 2015, Design and 3D Printing of Scaffolds and Tissues. Eng Proc, 1:261–8. DOI:10.15302/j-eng-2015061.
12. Domingos M, Chiellini F, Cometa S, et al., 2020, Evaluation of in vitro Degradation of PCL Scaffolds Fabricated via BioExtrusion. Part 1: Influence of the Degradation Environment. Virtual Phys Prototyp, 5:65–73. DOI:10.1080/17452751003769440.
13. Domingos M, Chiellini F, Cometa S, et al., 2011, Evaluation of in vitro Degradation of PCL Scaffolds Fabricated via BioExtrusion Part 2: Influence of Pore Size and Geometry. Virtual Phys Prototyp, 6, 157-165. DOI:10.1080/17452751003769440.
14. Liu F, Vyas C, Poologasundarampillai G, et al., 2018, Structural Evolution of PCL during Melt Extrusion 3D Printing. Macromol Mater Eng, 303:1700494. DOI: 10.1002/mame.201700494.
15. Liu F, Vyas C, Poologasundarampillai G, et al., 2018, Process-Driven Microstructure Control in Melt-Extrusion-Based 3D Printing for Tailorable Mechanical Properties in a Polycaprolactone Filament. Macromol Mater Eng, 303:1800173. DOI: 10.1002/mame.201800173.
16. Liu F, Wang W, Mirihanage W, et al. 2018, A Plasma assisted Bioextrusion System for Tissue Engineering. Cirp Ann Manufact Technol, 67:229–32. DOI: 10.1016/j.cirp.2018.04.077.
17. Wang WG, Caetano G, Ambler WS, et al., 2016, Enhancing the Hydrophilicity and Cell Attachment of 3D Printed PCL/Graphene Scaffolds for Bone Tissue Engineering. Materials, 9(12):992. DOI: 10.3390/ma9120992.
18. Wibowo A, Vyas C, Cooper G, et al., 2020, 3D Printing of Polycaprolactone Polyaniline Electroactive Scaffolds for Bone Tissue Engineering. Materials, 13:512. DOI: 10.3390/ma13030512.
19. Wang W, Junior JR, Nalesso PR, et al., 2019, Engineered 3D Printed poly(ɛcaprolactone)/Graphene Scaffolds for Bone Tissue Engineering. Mater Sci Eng C, 100:759–70. DOI: 10.1016/j.msec.2019.03.047.
20. Wang WG, Huang BY, Byun JJ, et al., 2019, Assessment of PCL/Carbon Material Scaffolds for Bone Regeneration. J Mech Behav Biomed Mater, 93:52–60. DOI: 10.1016/j.jmbbm.2019.01.020.
21. Cooper DR, D’Anjou B, Ghattamaneni N, et al., 2012, Experimental Review of Graphene. ISRN Condensed Matter Phys, 2012:56. DOI: 10.5402/2012/501686.
22. Wang W, Caetano GF, Chiang WH, et al., 2016, Morphological, Mechanical and Biological Assessment of PCL/Pristine Graphene Scaffolds for Bone Regeneration. Int J Bioprint, 2:95–104. DOI: 10.18063/ijb.2016.02.009.
23. Caetano GF, Wang W, Chiang WH, et al., 2018, 3D-Printed Poly(ɛ-caprolactone)/Graphene Scaffolds Activated with P1- Latex Protein for Bone Regeneration. 3d Print Addit Manuf, 5:127–37. DOI: 10.1089/3dp.2018.0012.correx.
24. Huang B, Vyas C, Roberts I, et al., 2019, Fabrication and Characterisation of 3D Printed MWCNT Composite Porous Scaffolds for Bone Regeneration. Mater Sci Eng C, 98:266–78. DOI: 10.1016/j.msec.2018.12.100.
25. Huang B, Vyas C, Byun JJ, et al., 2020, Aligned Multiwalled Carbon Nanotubes with Nanohydroxyapatite in a 3D Printed Polycaprolactone Scaffold Stimulates Osteogenic Differentiation. Mater Sci Eng C, 108:110374. DOI: 10.1016/j.msec.2019.110374.
26. Dreyer DR, Park S, Bielawski CW, et al., 2010, The Chemistry of Graphene Oxide. Chem Soc Rev, 39:228–40.
27. Sivashankari PR, Prabaharan M, 2020, Three-dimensional Porous Scaffolds based on Agarose/chitosan/graphene Oxide Composite for Tissue Engineering. Int J Biol Macromol, 146:222–31. DOI: 10.1016/j.ijbiomac.2019.12.219.
28. Saravanan S, Vimalraj S, Anuradha D, 2018, Chitosan Based Thermoresponsive Hydrogel Containing Graphene Oxide for Bone Tissue Repair. Biomed Pharmacother, 107:908–17. DOI: 10.1016/j.biopha.2018.08.072.
29. Zhang J, Zhu S, Song K, et al., 2020, 3D Reduced Graphene Oxide Hybrid Nano-copper Scaffolds with a High Antibacterial Performance. Mater Lett, 267:127527. DOI: 10.1016/j.matlet.2020.127527.
30. Min Lj, Leong SS, Miaomiao Z, Yee WY, 2018, 3D Bioprinting Processes: A Perspective on Classification and Terminology. Int J Bioprint, 4:151.
31. ASTM International, 2016, Standard Test Method for Compressive Properties of Rigid Cellular Plastics. ASTM International, West Conshohocken, PA.
32. ASTM International, 2015, Standard Test Method for Compressive Properties of Rigid Plastics. ASTM International, West Conshohocken, PA.
33. Ahmed SA, Gogal RM Jr., Walsh JE, 1994, A New Rapid and Simple Non-radioactive Assay to Monitor and Determine the Proliferation of Lymphocytes: An Alternative to [3H] thymidine Incorporation Assay. J Immunol Methods, 170:211–24. DOI: 10.1016/0022-1759(94)90396-4.
34. Ramazani S, Karimi M. 2015, Aligned Poly(ε-caprolactone)/Graphene Oxide and Reduced Graphene Oxide Nanocomposite Nanofibers: Morphological, Mechanical and Structural Properties. Mater Sci Eng C, 56:325–34. DOI: 10.1016/j.msec.2015.06.045.
35. Thomson RC, Yaszemski MJ, Powers JM, et al., 1996, Fabrication of Biodegradable Polymer Scaffolds to Engineer Trabecular Bone. J Biomater Sci Polym Ed, 7:23–38. DOI: 10.1163/156856295x00805.
36. Williams JM, Adewunmi A, Schek RM, et al., 2005, Bone Tissue Engineering Using Polycaprolactone Scaffolds Fabricated via Selective Laser Sintering. Biomaterials, 26:4817–27. DOI: 10.1016/j.biomaterials.2004.11.057.
37. Porter BD, Oldham JB, He LS, et al., 2000, Mechanical Properties of a Biodegradable Bone Regeneration Scaffold. J Biomech Eng, 122:286–8. DOI: 10.1115/1.429659.
38. Lotz JC, Gerhart TN, Hayes WC, 1990, Mechanical Properties of Trabecular Bone from the Proximal Femur: A Quantitative CT Study. J Comput Assist Tomogr, 14:107–14. DOI: 10.1097/00004728-199001000-00020.