The future of skin toxicology testing – Three-dimensional bioprinting meets microfluidics
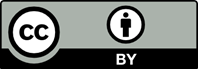
Over the years, the field of toxicology testing has evolved tremendously from the use of animal models to the adaptation of in vitro testing models. In this perspective article, we aim to bridge the gap between the regulatory authorities who performed the testing and approval of new chemicals and the scientists who designed and fabricated these in vitro testing models. An in-depth discussion of existing toxicology testing guidelines for skin tissue models (definition, testing models, principle, and limitations) is first presented to have a good understanding of the stringent requirements that are necessary during the testing process. Next, the ideal requirements of toxicology testing platform (in terms of fabrication, testing, and screening process) are then discussed. We envisioned that the integration of three-dimensional bioprinting within miniaturized microfluidics platform would bring about a paradigm shift in the field of toxicology testing; providing standardization in the fabrication process, accurate, and rapid deposition of test chemicals, real-time monitoring, and high throughput screening for more efficient skin toxicology testing.
1. Hartung T, 2009, Toxicology for The Twenty-first Century. Nature, 460(7252):208-12. DOI 10.1038/460208a.
2. Markets and Markets, 2016, Global in vitro Toxicology Testing Market by Product, Type (ADME). Toxicity Endpoints and Tests (Carcinogenicity, Dermal Toxicity), Technology (Genomics, Transcriptomics), Method (Cellular Assays), Industry (Pharmaceutical) Forecast to 2021, Report.
3. Lilienblum W, Dekant W, Foth H, et al., 2008, Alternative Methods to Safety Studies in Experimental Animals: Role in the Risk Assessment of Chemicals Under the New European Chemicals Legislation (REACH). Arch Toxicol, 82(4):211- 36. DOI 10.1007/s00204-008-0279-9.
4. Burden N, Sewell F, Chapman K, 2015, Testing Chemical Safety: What is Needed to Ensure the Widespread Application of Non-animal Approaches? PLoS Biol, 13(5):e1002156. DOI 10.1371/journal.pbio.1002156.
5. Hartung T, 2009, A Toxicology for the 21st Century Mapping the Road Ahead. Toxicol Sci, 109(1):18-23.
6. Ng WL, Chua CK, Shen YF, 2019, Print Me An Organ! Why We Are Not There Yet. Prog Polym Sci, 97:101145.
7. Ng WL, Yeong WY, Naing MW, 2014, Potential of Bioprinted Films for Skin Tissue Engineering. Proceedings of the 1st International Conference on Progress in Additive Manufacturing, p441-446. DOI 10.3850/978-981-09-0446-3_065.
8. Kathawala MH, Ng WL, Liu D, et al., 2019, Healing of Chronic Wounds An Update of Recent Developments and Future Possibilities. Tissue Eng Part B: Rev. DOI 10.1089/ ten.TEB.2019.0019.
9. Vijayavenkataraman S, Lu W, Fuh J, 2016, 3D Bioprinting of Skin: A State-of-the-art Review on Modelling, Materials, and Processes. Biofabrication, 8(3):32001. DOI 10.1088/1758- 5090/8/3/032001.
10. van den Broek LJ, Bergers LI, Reijnders CM, et al., 2017, Progress and Future Prospectives in Skin-on-chip Development with Emphasis on the Use of Different Cell Types and Technical Challenges. Stem Cell Rev Rep, 13(3):418-29. DOI 10.1007/s12015-017-9737-1.
11. Du G, Fang Q, den Toonder JM, 2016, Microfluidics for Cell-based High Throughput Screening Platforms A Review. Anal Chim Acta, 903:36-50. DOI 10.1016/j.aca.2015.11.023.
12. Barata D, van Blitterswijk C, Habibovic P, 2016, High-throughput Screening Approaches and Combinatorial Development of Biomaterials Using Microfluidics. Acta Biomater, 34:1-20.
13. MacNeil S, 2007, Progress and Opportunities for Tissue-engineered Skin. Nature, 445(7130):874-80. DOI 10.1016/j. actbio.2015.09.009. DOI 10.1038/nature05664.
14. Mathes SH, Ruffner H, Graf-Hausner U, 2014, The Use of Skin Models in Drug Development. Adv Drug Del Rev, 69:81-102. DOI 10.1016/j.addr.2013.12.006.
15. Ng WL, Goh MH, Yeong WY, et al., 2018, Applying Macromolecular Crowding to 3D Bioprinting: Fabrication of 3D Hierarchical Porous Collagen-based Hydrogel Constructs. Biomater Sci, 6(3):562-74. DOI 10.1039/c7bm01015j.
16. Ng WL, Wang S, Yeong WY, et al., Skin Bioprinting: Impending Reality or Fantasy? Trends Biotechnol, 34(9):689- 99. DOI 10.1016/j.tibtech.2016.08.009.
17. OECD, 2004, Skin Absorption: In Vitro Method. France: OECD.
18. OECD, 2014, In Vitro Skin Corrosion: Reconstructed Human Epidermis (RHE) Test Method. France: OECD. DOI 10.1787/9789264224193-en.
19. OECD, 2013, In Vitro Skin Irritation: Reconstructed Human Epidermis Test Method. France: OECD. DOI 10.1787/9789264203884-en.
20. OECD, 2015, In Vitro Skin Sensitization: ARE-Nrf2 Luciferase Test Method. France: OECD.
21. Sun T, Jackson S, Haycock JW, et al., 2006, Culture of Skin Cells in 3D Rather than 2D Improves their Ability to Survive Exposure to Cytotoxic Agents. J Biotechnol, 122(3):372-81. DOI 10.1016/j.jbiotec.2005.12.021.
22. Ackermann K, Borgia SL, Korting HC, et al., 2010, The Phenion Full-thickness Skin Model for Percutaneous Absorption Testing. Skin Pharmacol Physiol, 23(2):105-12. DOI 10.1159/000265681.
23. Horváth L, Umehara Y, Jud C, et al., 2015, Engineering an in vitro Air-blood Barrier by 3D Bioprinting. Sci Rep, 5:7974. DOI 10.1038/srep07974.
24. Lee JM, Ng WL, Yeong WY, 2019, Resolution and Shape in Bioprinting: Strategizing Towards Complex Tissue and Organ Printing. Appl Phys Rev, 6(1):11307. DOI 10.1063/1.5053909.
25. Boland T, Ovsianikov A, Chickov BN, et al., 2007, Rapid Prototyping of Artificial Tissues and Medical Devices. Adv Mater Process, 165(4):51-3.
26. Yeong WY, Chua CK, Leong KF, et al., 2005, Development of Scaffolds for Tissue Engineering Using a 3D Inkjet. Virtual Modelling and Rapid Manufacturing: Advanced Research in Virtual and Rapid Prototyping Proceeding 2nd International Conference on Advanced Research in Virtual and Rapid Prototyping, 28 Sep-1 Oct 2005, Leiria, Portugal: CRC Press, p115. DOI 10.1201/b15961-59.
27. Chua CK, Yeong WY, Leong KF, 2005, Rapid Prototyping in Tissue Engineering: A State-of-the-Art Report. Proceeding 2nd International Conference on Advanced Research in Virtual and Rapid Prototyping, p19-27.
28. Groll J, Burdick JA, Cho DW, et al., 2018, A Definition of Bioinks and Their Distinction from Biomaterial Inks. Biofabrication, 11(1):13001. DOI 10.1088/1758-5090/ aaec52.
29. Pourchet LJ, Thepot A, Albouy M, et al., 2017, Human Skin 3D Bioprinting Using Scaffold-free Approach. Adv Healthc Mater, 6(4):1601101. DOI 10.1002/adhm.201601101.
30. Cubo N, Garcia M, del Cañizo JF, et al., 2016, 3D Bioprinting of Functional Human Skin: Production and in vivo Analysis. Biofabrication, 9(1):15006. DOI 10.1088/1758- 5090/9/1/015006.
31. Ng WL, Yeong WY, Naing MW, 2016, Polyelectrolyte Gelatin-chitosan Hydrogel Optimized for 3D Bioprinting in Skin Tissue Engineering. Int J Bioprint, 2(1):53-62. DOI 10.18063/ijb.2016.01.009.
32. Ng WL, Yeong WY, Naing MW, 2016, Development of Polyelectrolyte Chitosan-gelatin Hydrogels for Skin Bioprinting. Procedia CIRP, 49:105-12. DOI 10.1016/j. procir.2015.09.002.
33. Koch L, Deiwick A, Schlie S, et al., 2012, Skin Tissue Generation by Laser Cell Printing. Biotechnol Bioeng, 109(7):1855-63. DOI 10.1002/bit.24455.
34. Michael S, Sorg H, Peck CT, et al., 2013, Tissue Engineered Skin Substitutes Created by Laser-Assisted Bioprinting Form Skin-Like Structures in the Dorsal Skin Fold Chamber in Mice. PloS One, 8(3):e57741. DOI 10.1371/journal. pone.0057741.
35. Koch L, Deiwick A, Franke A, et al., 2018, Laser Bioprinting of Human Induced Pluripotent Stem Cells the Effect of Printing and Biomaterials on Cell Survival, Pluripotency, and Differentiation. Biofabrication, 10(3):35005. DOI 10.1088/1758-5090/aab981.
36. Ng WL, Lee JM, Yeong WY, et al., 2017, Microvalve-based Bioprinting Process, Bio-inks and Applications. Biomater Sci, 5(4):632-47. DOI 10.1039/c6bm00861e.
37. Lee V, Singh G, Trasatti JP, et al., 2013, Design and Fabrication of Human Skin by Three-Dimensional Bioprinting. Tissue Eng Part C: Methods, 20(6):473-84. DOI 10.1089/ten. tec.2013.0335.
38. Ng WL, Yeong WY, Naing MW, 2016, Microvalve Bioprinting of Cellular Droplets with High Resolution and Consistency. Proceedings of the International Conference on Progress in Additive Manufacturing, p397-402.
39. Kim BS, Lee JS, Gao G, et al., 2017, Direct 3D Cell-printing of Human Skin with Functional Transwell System. Biofabrication, 9(2):25034. DOI 10.1088/1758-5090/aa71c8.
40. Derr K, Zou J, Luo K, et al., 2019, Fully Three-Dimensional Bioprinted Skin Equivalent Constructs with Validated Morphology and Barrier Function. Tissue Eng Part C: Methods, 25(6):334-43. DOI 10.1089/ten.tec.2018.0318.
41. Lee W, Debasitis JC, Lee VK, et al., 2009, Multi-layered Culture of Human Skin Fibroblasts and Keratinocytes Through Three-dimensional Freeform Fabrication. Biomaterials, 30(8):1587-1595. DOI 10.1016/j.biomaterials.2008.12.009.
42. Lee W, Lee V, Polio S, et al., 2010, On-demand Three-dimensional Freeform Fabrication of Multi-layered Hydrogel Scaffold with Fluidic Channels. Biotechnol Bioeng, 105(6):1178-86. DOI 10.1002/bit.22613.
43. Skardal A, Mack D, Kapetanovic E, et al., 2012, Bioprinted Amniotic Fluid-Derived Stem Cells Accelerate Healing of Large Skin Wounds. Stem Cells Trans Med, 1(11):792-802. DOI 10.5966/SCTM.2012-0088.
44. Albanna M, Binder KW, Murphy SV, et al., 2019, In Situ Bioprinting of Autologous Skin Cells Accelerates Wound Healing of Extensive Excisional Full-Thickness Wounds. Sci Rep, 9(1):1856. DOI 10.1038/S41598-018-38366-W.
45. Ng WL, Tan ZQ, Yeong WY, et al., 2018, Proof-of-concept: 3D Bioprinting of Pigmented Human Skin Constructs. Biofabrication, 10(2):25005. DOI 10.1088/1758-5090/ aa9e1e.
46. Min D, Lee W, Bae IH, et al., 2018, Bioprinting of Biomimetic Skin Containing Melanocytes. Exp Dermatol, 27(5):453-9. DOI 10.1111/exd.13376.
47. Yucha SEV, Tamamoto KA, Nguyen H, et al., 2019, Human Skin Equivalents Demonstrate Need for Neuro-Immuno- Cutaneous System. Adv Biosyst, 3(1):1800283. DOI 10.1002/ adbi.201800283.
48. Kim BS, Gao G, Kim JY, et al., 2019, 3D Cell Printing of Perfusable Vascularized Human Skin Equivalent Composed of Epidermis, Dermis, and Hypodermis for Better Structural Recapitulation of Native Skin. Adv Healthc Mater, 8(7):1801019. DOI 10.1002/adhm.201801019.
49. Pepper ME, Seshadri V, Burg TC, et al., 2012, Characterizing the Effects of Cell Settling on Bioprinter Output. Biofabrication, 4(1):11001. DOI 10.1088/1758- 5082/4/1/011001.
50. Jia J, Richards DJ, Pollard S, et al., 2014, Engineering Alginate as Bioink for Bioprinting. Acta Biomater, 10(10):4323-31.
51. Zhuang P, Ng WL, An J, et al., 2019, Layer-by-layer Ultraviolet Assisted Extrusion-based (UAE) Bioprinting of Hydrogel Constructs with High Aspect Ratio for Soft Tissue Engineering Applications. PLoS One, 14(6):e216776. DOI 10.1371/journal.pone.0216776.
52. Ng WL, Yeong WY, Naing MW, 2017, Polyvinylpyrrolidone- Based Bio-Ink Improves Cell Viability and Homogeneity During Drop-On-Demand Printing. Materials, 10(2):190. DOI 10.3390/ma10020190.
53. Bouhifd M, Bories G, Casado J, et al., 2012, Automation of an in vitro Cytotoxicity Assay Used to Estimate Starting Doses in Acute Oral Systemic Toxicity Tests. Food Chem Toxicol, 50(6):2084-96. DOI 10.1016/j.fct.2012.03.046.
54. Jones RE, Zheng W, McKew JC, et al., 2013, An Alternative Direct Compound Dispensing Method Using the HP D300 Digital Dispenser. J Lab Auto, 18(5):367-74. DOI 10.1177/2211068213491094.
55. Ronaldson-Bouchard K, Vunjak-Novakovic G, 2018, Organs-on-a-chip: A Fast Track for Engineered Human Tissues in Drug Development. Cell Stem Cell, 22(3):310-24. DOI 10.1016/j.stem.2018.02.011.
56. Yu F, Choudhury D, 2019, Microfluidic Bioprinting for Organ-on-a-chip Models. Drug Discov Today, 24(6):1248- 57. DOI 10.1016/j.drudis.2019.03.025.
57. Park TH, Shuler ML, 2003, Integration of Cell Culture and Microfabrication Technology. Biotechnol Progr, 19(2):243-53. DOI 10.1021/bp020143k.
58. Thorsen T, Maerkl SJ, Quake SR, 2002, Microfluidic Large-scale Integration. Science, 298(5593):580-4. DOI 10.1126/ science.1076996.
59. Grover WH, Ivester RH, Jensen EC, et al., 2006, Development and Multiplexed Control of Latching Pneumatic Valves Using Microfluidic Logical Structures. Lab Chip, 6(5):623-31. DOI 10.1039/b518362f.
60. Mosadegh B, Kuo CH, Tung YC, et al., 2010, Integrated Elastomeric Components for Autonomous Regulation of Sequential and Oscillatory Flow Switching in Microfluidic Devices. Nat Phys, 6(6):433. DOI 10.1038/nphys1637.
61. Abaci HE, Gledhill K, Guo Z, et al., 2015, Pumpless Microfluidic Platform for Drug Testing on Human Skin Equivalents. Lab Chip, 15(3):882-8. DOI 10.1039/ c4lc00999a.
62. Sriram G, Alberti M, Dancik Y, et al., 2018, Full-thickness Human Skin-on-chip with Enhanced Epidermal Morphogenesis and Barrier Function. Mater Today, 21(4):326-40. DOI 10.1016/j.mattod.2017.11.002.
63. Alberti M, Dancik Y, Sriram G, et al., 2017, Multi-chamber Microfluidic Platform for High-precision Skin Permeation Testing. Lab Chip, 17(9):1625-34. DOI 10.1039/c6lc01574c.
64. Huh D, Hamilton GA, Ingber DE, 2011, From 3D Cell Culture to Organs-on-chips. Trends Cell Biol, 21(12):745-54. DOI 10.1016/j.tcb.2011.09.005.
65. Breslin S, O’Driscoll L, 2013, Three-dimensional Cell Culture: The Missing Link in Drug Discovery. Drug Discov Today, 18(5-6):240-9. DOI 10.1016/j.drudis.2012.10.003.
66. Laschke MW, Menger MD, 2017, Life is 3D: Boosting Spheroid Function for Tissue Engineering. Trends Biotechnol, 35:133-44. DOI 10.1016/j.tibtech.2016.08.004.
67. Choudhury D, Anand S, Naing MW, 2018, The Arrival of Commercial Bioprinters Towards 3D Bioprinting Revolution. Int J Bioprint, 4(2):139-58. DOI 10.18063/ijb.v4i2.139.
68. Göhl J, Markstedt K, Mark A, et al., 2018, Simulations of 3D Bioprinting: Predicting Bioprintability of Nanofibrillar Inks. Biofabrication, 10(3):34105. DOI 10.1088/1758-5090/ aac872.
69. Hölzl K, Lin S, Tytgat L, et al., 2016, Bioink Properties Before, During and After 3D Bioprinting. Biofabrication, 8(3):32002. DOI 10.1088/1758-5090/8/3/032002.
70. Choudhury D, Tun HW, Wang T, et al., 2018, Organ-derived Decellularized Extracellular Matrix: A Game Changer for Bioink Manufacturing? Trends Biotechnol, 36(8):787-805. DOI 10.1016/j.tibtech.2018.03.003.
71. Gungor-Ozkerim PS, Inci I, Zhang YS, et al., 2018, Bioinks for 3D Bioprinting: An Overview. Biomater Sci, 6(5):915-46. DOI 10.1039/c7bm00765e.
72. Eglen RM, Randle DH, 2015, Drug Discovery Goes Three-dimensional: Goodbye to Flat High-throughput Screening? Assay Drug Dev Technol, 13(5):262-5. DOI 10.1089/ adt.2015.647.
73. Mazzocchi A, Soker S, Skardal A, 2019, 3D Bioprinting for High-throughput Screening: Drug Screening, Disease Modeling, and Precision Medicine Applications. Appl Phys Rev, 6(1):11302. DOI 10.1063/1.5056188.