A multi-scale porous scaffold fabricated by a combined additive manufacturing and chemical etching process for bone tissue engineering
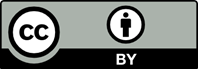
It is critical to develop a fabrication technology for precisely controlling an interconnected porous structure of scaffolds to mimic the native bone microenvironment. In this work, a novel combined process of additive manufacturing (AM) and chemical etching was developed to fabricate graphene oxide/poly(L-lactic acid) (GO/PLLA) scaffolds with multiscale porous structure. Specially, AM was used to fabricate an interconnected porous network with pore sizes of hundreds of microns. And the chemical etching in sodium hydroxide solution constructed pores with several microns or even smaller on scaffolds surface. The degradation period of the scaffolds was adjustable via controlling the size and quantity of pores. Moreover, the scaffolds exhibited surprising bioactivity after chemical etching, which was ascribed to the formed polar groups on scaffolds surfaces. Furthermore, GO improved the mechanical strength of the scaffolds.
[1]Tavolaro P, Catalano S, Martino G, et al., 2016, Zeolite inorganic scaffolds for novel biomedical application: Effect of physicochemical characteristic of zeolite membranes on cell adhesion and viability. Appl Surf Sci, 380: 135–140. http://doi.org/10.1016/j.apsusc.2016.01.279
[2]D P, AM P, A P, et al., 2016, Levofloxacin-loaded star poly(ε-caprolactone) scaffolds by additive manufacturing. J Mater Sci Mater Med, 27(3): 44. http://doi.org/10.1007/s10856-015-5658-1
[3]Fradique R, Correia T R, Miguel S P, et al., 2016, Production of new 3D scaffolds for bone tissue regeneration by rapid prototyping. J Mater Sci Mater Med, 27(4): 1–14. http://doi.org/10.1007/s10856-016-5681-x
[4]Gao C, Deng Y, Feng P, et al., 2014, Current progress in bioactive ceramic scaffolds for bone repair and regeneration. Int J Mol Sci, v15(3): 4714–4732. http://doi.org/10.3390/ijms15034714
[5]Yuan H, Zhou Q, Li B, et al., 2015, Direct printing of patterned three-dimensional ultrafine fibrous scaffolds by stable jet electrospinning for cellular ingrowth. Biofabrication, 7(4): 045004. http://doi.org/10.1088/1758-5090/7/4/045004
[6]Gao C, Peng S, Feng P, et al., 2017, Bone biomaterials and interactions with stem cells. Bone Res, 21; 5: 17059. http://doi.org/10.1038/boneres.2017.59
[7]Ng R, Zhang X, Liu N, et al., 2009, Modifications of nonwoven polyethylene terephthalate fibrous matrices via NaOH hydrolysis: Effects on pore size, fiber diameter, cell seeding and proliferation. Process Biochem, 44(9): 992–998. http://doi.org/10.1016/j.procbio.2009.04.024
[8]Bose S, Roy M, Bandyopadhyay A, 2012, Recent advances in bone tissue engineering scaffolds. Trends Biotechnol, 30(10): 546. http://doi.org/10.1016/j.tibtech.2012.07.005
[9]Inzana J A, Olvera D, Fuller S M, et al., 2014, 3D printing of composite calcium phosphate and collagen scaffolds for bone regeneration. Biomaterials, 35(13): 4026–4034.http://doi.org/10.1016/j.biomaterials.2014.01.064
[10]Sun H, Zhu F, Hu Q, et al., 2014, Controlling stem cell-mediated bone regeneration through tailored mechanical properties of collagen scaffolds. Biomaterials, 35(4): 1176–1184. http://doi.org/10.1016/j.biomaterials.2013.10.054
[11]Zhang X, Zhang Y, Ma G, et al., 2015, The Effect of Prefrozen Process on Properties of Chitosan/ Hydroxyapatite/ Poly(methyl methacrylate) Composite Prepared by Freeze Drying method Used for Bone Tissue Engineering. RSC Adv, 5(97): 79679–79686. http://doi.org/10.1039/C5RA14549J
[12]Sultana N, Wang M, 2012, PHBV/PLLA-based composite scaffolds fabricated using an emulsion freezing/freeze-drying technique for bone tissue engineering: surface modification and in vitro biological evaluation. Biofabrication, 4(1): 015003. http://doi.org/10.1088/1758-5082/4/1/015003
[13]Soh E, Kolos E, Ruys A J, 2015, Foamed high porosity alumina for use as a bone tissue scaffold. Ceram Int, 41(1): 1031–1047. https://doi.org/10.1016/j.ceramint.2014.09.026
[14]Wang C, Chen H, Zhu X, et al., 2017, An improved polymeric sponge replication method for biomedical porous titanium scaffolds. Mater Sci Eng C Mater Biol Appl, 70(Pt 2): 1192. http://doi.org/10.1016/j.msec.2016.03.037
[15]Baino F, Vitale-Brovarone C, 2014, Mechanical properties and reliability of glass–ceramic foam scaffolds for bone repair. Mater Lett, 118(3): 27–30. https://doi.org/10.1016/j.matlet.2013.12.037
[16]Kumar A, Mandal S, Barui S, et al., 2016, Low temperature additive manufacturing of three dimensional scaffolds for bone-tissue engineering applications: Processing related challenges and property assessment. Mater Sci Eng R Rep, 103: 1–39. https://doi.org/10.1016/j.mser.2016.01.001
[17]Yang Y, Wu P, Lin X, et al., 2016, System development, formability quality and microstructure evolution of selective laser-melted magnesium. Virtual Phys Prototyp, 11(3): 173–181. http://doi.org/10.1080/17452759.2016.1210522
[18]Ng W L, Goh M H, Yeong W Y, et al., 2018, Applying macromolecular crowding to 3D bioprinting: Fabrication of 3D hierarchical porous collagen-based hydrogel constructs. Biomater Sci, 6(3): 562–574. http://doi.org/10.1039/c7bm01015j
[19]Pei F, Peng S, Ping W, et al., 2016, A nano-sandwich construct built with graphene nanosheets and carbon nanotubes enhances mechanical properties of hydroxyapatite–polyetheretherketone scaffolds. Int J Nanomed, 11: 3487–3500. http://doi.org/10.2147/IJN.S110920
[20]Shuai C, Guo W, Gao C, et al., 2017, An nMgO containing scaffold: Antibacterial activity, degradation properties and cell responses. Int J Bioprint, 4(1): 120. http://dx.doi.org/10.18063/IJB.v4i1.120
[21]Deng Y, Yang Y, Gao C, et al., 2018, Mechanism for corrosion protection of β-TCP reinforced ZK60 via laser rapid solidification. Int J Bioprint, 4(1): 124. http://dx.doi.org/10.18063/IJB.v4i1.124
[22]Wang Z, Macosko C W, Bates F S, 2014, Tuning surface properties of poly(butylene terephthalate) melt blown fibers by alkaline hydrolysis and fluorination. ACS Appl Mater Interfaces, 6(14): 11640. http://doi.org/10.1021/am502398u
[23]Pei F, Peng S, Ping W, et al., 2016, A space network structure constructed by tetraneedlelike ZnO whiskers supporting boron nitride nanosheets to enhance comprehensive properties of poly(L-lacti acid) scaffolds. Sci Rep, 6: 33385. http://doi.org/10.1038/srep33385
[24]Cardea S, Baldino L, Pisanti P, et al., 2014, 3-D PLLA scaffolds formation by a supercritical freeze extraction assisted process. J Mater Sci Mater Med, 25(2): 355–362. http://doi.org/10.1007/s10856-013-5069-0
[25]Peng F, Olson J R, Shaw M T, et al., 2009, Influence of pretreatment on the surface characteristics of PLLA fibers and subsequent hydroxyapatite coating. J Biomed Mater Res B Appl Biomater, 88(1): 220. http://doi.org/10.1002/jbm.b.31172
[26]Wang H, Qiu Z, 2012, Crystallization kinetics and morphology of biodegradable poly(l-lactic acid)/graphene oxide nanocomposites: Influences of graphene oxide loading and crystallization temperature. Thermochim Acta, 527(1): 40–46.
[27]Shuai C, Feng P, Wu P, et al., 2016, A combined nanostructure constructed by graphene and boron nitride nanotubes reinforces ceramic scaffolds. Chem Eng J, 313: 487–497. https://doi.org/10.1016/j.cej.2016.11.095
[28]Gao C, Pei F, Peng S, et al., 2017, Carbon nanotubes, graphene and boron nitride nanotubes reinforced bioactive ceramics for bone repair. Acta Biomater, 61: 1–20. https://doi.org/10.1016/j.actbio.2017.05.020
[29]Shuai C, Gao C, Nie Y, et al., 2011, Structure and properties of nano-hydroxypatite scaffolds for bone tissue engineering with a selective laser sintering system. Nanotechnology, 22(28): 285703. http://doi.org/10.1088/0957-4484/22/28/285703
[30]Wu D, Xu F, Sun B, et al., 2012, Design and preparation of porous polymers. Chem Rev, 112(7): 3959.
[31]Johnson A J W, Herschler B A, 2011, A review of the mechanical behavior of CaP and CaP/polymer composites for applications in bone replacement and repair. Acta Biomater, 7(1): 16–30. http://doi.org/10.1016/j.actbio.2010.07.012
[32]Shuai C, Feng P, Gao C, et al., 2015, Graphene oxide reinforced poly(vinyl alcohol): Nanocomposite scaffolds for tissue engineering applications. RSC Adv, 5(32): 25416–25423. http://doi.org/10.1039/C4RA16702C
[33]Li Y, Wang F, Yang J, et al., 2007, In vitro synthesis and characterization of amorphous calcium phosphates with various Ca/P atomic ratios. J Mater Sci Mater Med, 18(12): 2303–2308. http://doi.org/10.1007/s10856-007-3132-4
[34]Park G E, Pattison M A, Park K, et al., 2005, Accelerated chondrocyte functions on NaOH-treated PLGA scaffolds. Biomaterials, 26(16):3075–3082. http://doi.org/10.1016/j.biomaterials.2004.08.005
[35]Gautier G, Kouassi S, Desplobain S, et al., 2012, Macroporous silicon hydrogen diffusion layers for micro-fuel cells: From planar to 3D structures. Microelectron Eng, 90(2):79–82. https://doi.org/10.1016/j.mee.2011.04.003
[36]Yerokhov V Y, Hezel R, Lipinski M, et al., 2002, Cost-effective methods of texturing for silicon solar cells. Sol Energy Mater Sol Cells, 72(1–4):291–298. https://doi.org/10.1016/S0927-0248(01)00177-5
[37]Dinarvand P, Seyedjafari E, Shafiee A, et al., 2011, New approach to bone tissue engineering: Simultaneous application of hydroxyapatite and bioactive glass coated on a poly(L-lactic acid) scaffold. ACS Appl Mater Interfaces, 3(11): 4518–4524. http://doi.org/10.1021/am201212u
[38]Wang Z, Xu Y, Wang Y, et al., 2016, Enhanced in vitro mineralization and in vivo osteogenesis of composite scaffolds through controlled surface grafting of L-lactic acid oligomer on nanohydroxyapatite. Biomacromolecules, 17(3): 818–829. http://doi.org/10.1021/acs.biomac.5b01543
[39]Liu F, Qiu W, Wang H, et al., 2013, Biomimetic deposition of apatite coatings on biomedical NiTi alloy coated with amorphous titanium oxide by microarc oxidation. Mater Sci Technol, 29(6): 749–753. https://doi.org/10.1179/1743284712Y.0000000196
[40]Jiao Y-P, Cui F-Z, 2007, Surface modification of polyester biomaterials for tissue engineering. Biomed Mater, 2(4): R24. http://doi.org/10.1088/1748-6041/2/4/R02
[41]Wu S, Liu X, Yeung K W, et al., 2014, Biomimetic porous scaffolds for bone tissue engineering. Mater Sci Eng R Rep, 80: 1–36. https://doi.org/10.1016/j.mser.2014.04.001
[42]Xia L, Feng B, Wang P, et al., 2012, In vitro and in vivo studies of surface-structured implants for bone formation. Int J Nanomed, 7: 4873. http://dx.doi.org/10.2147/IJN.S29496
[43]An Y, Xu X, Gui K, 2016, Effect of SiC whiskers and graphene nanosheets on the mechanical properties of ZrB2-SiCw-Graphene ceramic composites. Ceram Int, 42(12): 14066–14070. https://doi.org/10.1016/j.ceramint.2016.06.014
[44]Gao C, Liu T, Shuai C, et al., 2014, Enhancement mechanisms of graphene in nano-58S bioactive glass scaffold: Mechanical and biological performance. Sci Rep, 4(4): 4712. https://doi.org/10.1038/srep04712
[45]Depan D, Girase B, Shah J, et al., 2011, Structure–process–property relationship of the polar graphene oxide-mediated cellular response and stimulated growth of osteoblasts on hybrid chitosan network structure nanocomposite scaffolds. Acta Biomater, 7(9): 3432–3445. https://doi.org/10.1016/j.actbio.2011.05.019
[46]Guo C X, Zheng X T, Lu Z S, et al., 2010, Biointerface by cell growth on layered graphene–artificial peroxidase–protein nanostructure for in situ quantitative molecular detection. Adv Mater, 22(45): 5164–5167. https://doi.org/10.1002/adma.201001699
[47]Liu Y, Yu D, Zeng C, et al., 2010, Biocompatible graphene oxide-based glucose biosensors. Langmuir, 26(9): 6158–6160. https://doi.org/10.1021/la100886x