Pre-clinical evaluation of advanced nerve guideconduits using a novel 3D in vitro testing model
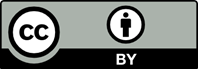
Autografts are the current gold standard for large peripheral nerve defects in clinics despite the frequentlyoccurring side effects like donor site morbidity. Hollow nerve guidance conduits (NGC) are proposed alternatives toautografts, but failed to bridge gaps exceeding 3 cm in humans. Internal NGC guidance cues like microfibresare believed to enhance hollow NGCs by giving additional physical support for directed regeneration of Schwann cellsand axons. In this study, we report a new 3D in vitro model that allows the evaluation of different intraluminal fibrescaffolds inside a complete NGC. The performance of electrospun polycaprolactone (PCL) microfibres inside 5 mmlong polyethylene glycol (PEG) conduits were investigated in neuronal cell and dorsal root ganglion (DRG) culturesinvitro. Z-stack confocal microscopy revealed the aligned orientation of neuronal cells along the fibres throughout thewhole NGC length and depth. The number of living cells in the centre of the scaffold was not significantly different tothe tissue culture plastic (TCP) control. For ex vivo analysis, DRGs were placed on top of fibre-filled NGCs to simulatethe proximal nerve stump. In 21 days of culture, Schwann cells and axons infiltrated the conduits along the microfibreswith 2.2 ± 0.37 mm and 2.1 ± 0.33 mm, respectively. We conclude that this in vitro model can help define internal NGCscaffolds in the future by comparing different fibre materials, composites and dimensions in one setup prior to animal testing.
[1]Rotshenker S, 2011, Wallerian degeneration: The innate-immune response to traumatic nerve injury. J Neuroinflammation, 8 (1): 1–14. http://doi.org/10.1186/1742–2094–8–109
[2]Sunderland S, 1951, A classification of peripheral nerve injuries producing loss of function. Brain, 74(4): 491–516.
[3]Brenner M J, Hess J R, Myckatyn T M, et al., 2006, Repair of motor nerve gaps with sensory nerve inhibits regeneration in rats. Laryngoscope, 116(9): 1685–1692. http://doi.org/10.1097/01.mlg.0000229469.31749.91
[4]Hallgren A, Bjorkman A, Chemnitz A, et al., 2013, Subjective outcome related to donor site morbidity after sural nerve graft harvesting: A survey in 41 patients. BMC Surg, 13 (1): 1–7. http://doi.org/10.1186/1471-2482–13–39
[5]Deumens R, Bozkurt A, Meek M F, et al., 2010, Repairing injured peripheral nerves: Bridging the gap. Prog Neurobiol, 92(3): 245-276. http://doi.org/10.1016/j. pneurobio.2010.10.002
[6]Meek M F, Coert J H, 2008, US Food and Drug Administration /Conformit Europe-approved absorbable nerve conduits for clinical repair of peripheral and cranial nerves. Ann Plast Surg, 60(1): 110–116. http://doi.org/10.1097/SAP.0b013e31804d441c
[7]Kehoe S, Zhang X F, Boyd D, 2012, FDA approved guidance conduits and wraps for peripheral nerve injury: A review of materials and efficacy. Injury, 43(5):553–572. http://doi.org/10.1016/j.injury.2010.12.030
[8]Ma F, Xiao Z, Meng D, et al., 2014, Use of natural neural scaffolds consisting of engineered vascular endothelial growth factor immobilized on ordered collagen fibers filled in a collagen tube for peripheral nerve regeneration in rats. Int J Mol Sci, 15(10): 18593–18609. http://doi.org/10.3390/ijms151018593
[9]Koh H S, Yong T, Teo W E, et al., 2010, In vivo study of novel nanofibrous intra-luminal guidance channels to promote nerve regeneration. J Neural Eng, 7(4): 046003. http://doi.org/10.1088/1741–2560/7/4/046003
[10]Jeffries E M, Wang Y, 2013, Incorporation of parallel electrospun fibers for improved topographical guidance in 3D nerve guides. Biofabrication, 5(3): 035015. http://doi.org/10.1088/1758–5082/5/3/035015
[11]Ngo T T, Waggoner P J, Romero A A, et al., 2003, Poly(L-lactide) microfilaments enhance peripheral nerve regeneration across extended nerve lesions. J Neurosci Res, 72(2): 227–238. http://doi.org/10.1002/ jnr.10570
[12]Faroni A, Mobasseri S A, Kingham P J, et al., 2015, Peripheral nerve regeneration: Experimental strategies and future perspectives. Adv Drug Deliv Rev, 82–83: 160–167. http://doi.org/10.1016/j.addr.2014.11.010
[13]Griffin M F, Malahias M, Hindocha S, et al., 2014, Peripheral nerve injury: Principles for repair and regeneration. Open Orthop J, 8: 199–203. http://doi.org/10.2174/1874325001408010199
[14]Toba T, Nakamura T, Shimizu Y, et al., 2001, Regeneration of canine peroneal nerve with the use of a polyglycolic acid-collagen tube filled with laminin-soaked collagen sponge: A comparative study of collagen sponge and collagen fibers as filling materials for nerve conduits. J Biomed Mater Res, 58(6): 622–630.
[15]Wang X, Hu W, Cao Y, et al., 2005, Dog sciatic nerve regeneration across a 30-mm defect bridged by a chitosan/PGA artificial nerve graft. Brain, 128(Pt 8): 1897–1910. http://doi.org/10.1093/brain/awh517
[16]Daly W T, Yao L, Abu-rub M T, et al., 2012, The effect of intraluminal contact mediated guidance signals on axonal mismatch during peripheral nerve repair. Biomaterials, 33(28):6660–6671.http://doi.org/10.1016/j.biomaterials.2012.06.002
[17]Pateman C J, Harding A J, Glen A, et al., 2015, Nerve guides manufactured from photocurable polymers to aid peripheral nerve repair. Biomaterials, 49: 77–89. http://doi.org/10.1016/j.biomaterials.2015.01.055
[18]Daud M F, Pawar K C, Claeyssens F, et al., 2012, An aligned 3D neuronal-glial co-culture model for peripheral nerve studies. Biomaterials, 33(25): 5901–5913. http://doi.org/10.1016/j.biomaterials.2012.05.008
[19]Edelman D B, Keefer E W, 2005, A cultural renaissance: In vitro cell biology embraces three-dimensional context. Exp Neurol, 192(1): 1. http://doi.org/10.1016/j.expneurol.2004.10.005
[20]Pampaloni F, Reynaud E G, Stelzer E H, 2007, The third dimension bridges the gap between cell culture and live tissue. Nat Rev Mol Cell Biol, 8(10): 839–845. http://doi.org/ 10.1038/nrm2236
[21]Ravi M, Paramesh V, Kaviya S R, et al., 2015, 3D cell culture systems: Advantages and applications. J Cell Physiol, 230(1): 16–26. http://doi.org/10.1002/jcp.24683
[22]Mazzoleni G, Di Lorenzo D, Steimberg N, 2009, Modelling tissues in 3D: The next future of pharmaco-toxicology and food research?. Genes Nutr, 4(1): 13–22. http://doi.org/10.1007/s12263–008–0107–0
[23]Kaplan H M, Mishra P, Kohn J, 2015, The overwhelming use of rat models in nerve regeneration research may compromise designs of nerve guidance conduits for humans. J Mater Sci Mater Med, 26(8): 226. http://doi.org/10.1007/s10856–015–5558–4
[24]Teixeira F G, Vasconcelos N L, Gomes E D, et al., 2016, Bioengineered cell culture systems of central nervous system injury and disease. Drug Discov Today, 21(9): 1456–1463. http://doi.org/10.1016/j.drudis.2016.04.020
[25]Bosi S, Rauti R, Laishram J, et al., 2015, From 2D to 3D: Novel nanostructured scaffolds to investigate signalling in reconstructed neuronal networks. Sci Rep, 5: 9562. http://doi.org/10.1038/srep09562
[26]Dunn J C, Chan W Y, Cristini V, et al., 2006, Analysis of cell growth in three-dimensional scaffolds. Tissue Eng, 12(4): 705–716. http://doi.org/10.1089/ten.2006.12.705
[27]Sodian R, Hoerstrup S P, Sperling J S, et al., 2000, Tissue engineering of heart valves: In vitro experiences. Ann Thorac Surg, 70(1): 140–4.
[28]Burg K J, Holder W D, Jr., Culberson C R, et al., 2000, Comparative study of seeding methods for three- dimensional polymeric scaffolds. J Biomed Mater Res, 52(3): 576.
[29]Yang T H, Miyoshi H, Ohshima N, 2001, Novel cell immobilization method utilizing centrifugal force to achieve high-density hepatocyte culture in porous scaffold. J Biomed Mater Res, 55(3): 379–86.
[30]Krames E S, 2015, The dorsal root ganglion in chronic pain and as a target for neuromodulation: A review. Neuromodulation, 18(1): 24–32. http://doi.org/10.1111/ ner.12247
[31]Gaudet A D, Popovich P G, Ramer M S, 2011, Wallerian degeneration: Gaining perspective on inflammatory events after peripheral nerve injury. J Neuroinflammation, 8: 110. 10.1186/1742–2094–8–110. http://doi.org/10.1186/1742-2094-8-110
[32]Bozkurt A, Brook G A, Moellers S, et al., 2007, In vitro assessment of axonal growth using dorsal root ganglia explants in a novel three-dimensional collagen matrix. Tissue Eng, 13(12): 297–299. http://doi.org/10.1089/ten.2007.0116
[33]Rangappa N, Romero A, Nelson K D, et al., 2000, Laminin-coated poly (L-lactide) filaments induce robust neurite growth while providing directional orientation. J Biomed Mater Res, 51(4): 625–634.
[34]Huval R M, Miller O H, Curley J L, et al., 2015, Microengineered peripheral nerve-on-a-chip for preclinical physiological testing. Lab Chip, 15(10): 22–32. http://doi.org/10.1039/c4lc01513d
[35]Graham M Land Prescott M J, 2015, The multifactorial role of the 3Rs in shifting the harm-benefit analysis in animal models of disease. Eur J Pharmacol, 759: 19–29. http://doi.org/10.1016/j.ejphar.2015.03.040
[36]Cho D W, Kang H W, 2012, Microstereolithographybased computer-aided manufacturing for tissue engineering. Methods Mol Biol, 868: 341–356. http://doi.org/10.1007/978–1–61779–764–4_21
[37]Kim Y T, Haftel V K, Kumar S, et al., 2008, The role of aligned polymer fiber-based constructs in the bridging of long peripheral nerve gaps. Biomaterials, 29(21): 31–27. http://doi.org/10.1016/j.biomaterials.2008.03.042
[38]Kwak S, Haider A, Gupta K C, et al., 2016, Micro/Nano multilayered scaffolds of PLGA and collagen by alternately electrospinning for bone tissue engineering. Nanoscale Res Lett, 11(1): 323. http://doi.org/10.1186/s11671–016–1532–4
[39]Tan Z, Wang H, Gao X, et al., 2016, Composite vascular grafts with high cell infiltration by co-electrospinning. Mater Sci Eng C Mater Biol Appl, 67: 369–377. http://doi.org/10.1016/j.msec.2016.05.067
[40]Woodruff M A, Hutmacher D W, 2010, The return of a forgotten polymer-Polycaprolactone in the 21st century. Prog Polym SCI, 35(10): 1217–1256. http://doi.org/10.1016/j.progpolymsci.2010.04.002
[41]Nectow A R, Marra K G, Kaplan D L, 2012, Biomaterials for the development of peripheral nerve guidance conduits. Tissue Eng Part B Rev, 18(1): 40–50. http://doi.org/10.1089/ten.TEB.2011.0240
[42]Hopkins T M, Little K J, Vennemeyer J J, et al., 2017, Short and long gap peripheral nerve repair with magnesium metal filaments. J Biomed Mater Res A, 105(11): 3148–3158. http://doi.org/10.1002/jbm.a.36176
[43]Shahriari D, Shibayama M, Lynam D, et al., 2017, Peripheral nerve growth within a hydrogel microchannel scaffold supported by a kink-resistant conduit. J Biomed Mater Res A, 105(12): 3392–3399. http://doi.org/10.1002/jbm.a.36186
[44]Jiang X, Lim S H, Mao H Q, et al., 2010, Current applications and future perspectives of artificial nerve conduits. Experimental Neurology, 223(1): 86–101. http://doi.org/10.1016/j.expneurol.2009.09.009
[45]Daly W, Yao L, Zeugolis D, et al., 2012, A biomaterials approach to peripheral nerve regeneration: Bridging the peripheral nerve gap and enhancing functional recovery. J R Soc Interface, 9(67): 202–221. http://doi.org/10.1098/ rsif.2011.0438