Fabrication of titanium based biphasic scaffold using selective laser melting and collagen immersion
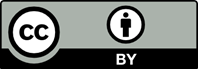
Tissue engineering approaches have been adopted to address challenges in osteochondral tissue regeneration. Single phase scaffolds, which consist of only one single material throughout the whole structure, have been used extensively in these tissue engineering approaches. However, a single phase scaffold is insufficient in providing all the properties required for regeneration and repair of osteochondral defects. Biphasic scaffolds with two distinct phases of titanium/type 1 c ollagen and titanium-tantalum/type 1 collagen were developed for the first time using selective laser melting and collagen infiltration. Observation of the biphasic scaffolds demonstrated continuous interface between the two phases and mechanical characterization of the metallic scaffolds support the feasibility of the newly developed scaffolds for tissue engineering in osteochondral defects.
1. Csaki C, Schneider P R A, and Shakibaei M, 2008, Mesenchymal stem cells as a potential pool for cartilage tissue engineering. Annals of Anatomy-Anatomischer Anzeiger, vol. 190(5): 395-412.
https://doi.org/10.1016/j.aanat.2008.07.007
2. Kon E, Filardo G, Di Martino A, et al., 2014, Clinical results and MRI evolution of a nano-composite multi-layered biomaterial for osteochondral regeneration at 5 years. American Journal of Sports Medicine, vol. 42(1): 158-165. https://doi.org/10.1177/0363546513505434
3. Panseri S, Russo A, Cunha C, et al., 2012, Osteochondral tissue engineering approaches for articular cartilage and subchondral bone regeneration. Knee Surgery Sports Traumatology Arthroscopy, vol. 20(6): 1182-1191.
https://doi.org/10.1007/s00167-011-1655-1
4. Wang S, Taraballi F, Tan L P, et al., 2012, Human keratin hydrogels support fibroblast attachment and proliferation in vitro. Cell and Tissue Research, vol. 347(3): 795- 802. https://doi.org/10.1007/s00441-011-1295-2
5. Wang S, Wang Z, Foo S E M, et al., 2015, Culturing Fibroblasts in 3D Human Hair Keratin Hydrogels. ACS Applied Materials & Interfaces, vol. 7(9): 5187-5198.
https://doi.org/10.1021/acsami.5b00854
6. Hollister S J, 2005, Porous scaffold design for tissue engineering. Nature Materials, vol. 4(7): 518-524.
https://doi.org/10.1038/nmat1421
7. Duan X, Zhu X, Dong Z, et al., 2013, Repair of large osteochondral defects in a beagle model with a novel type I collagen/glycosaminoglycan-porous titanium biphasic scaffold. Materials Science & Engineering C-Materials for Biological Applications, vol. 33(7): 3951- 3957. https://doi.org/10.1016/j.msec.2013.05.040
8. Nover A B, Lee S L, Georgescu M S, et al., 2015, Porous titanium bases for osteochondral tissue engineering. Acta Biomaterialia, vol. 27: 286-293.
https://doi.org/10.1016/j.actbio.2015.08.045
9. Chua C K, Yeong W Y, and Leong K F, 2005, Rapid prototyping in tissue engineering: A state-of-the-art report. Virtual modeling and rapid manufacturing, 19-27.
10. Yeong, W Y, Chua C K, Leong K F, et al., 2005, Development of scaffolds for tissue engineering using a 3D inkjet model maker. Virtual modelling and rapid manufacturing-advanced research in virtual and rapid proto-typing, 115-118.
11. Lee J M, Zhang M, and Yeong W Y, 2016, Characterization and evaluation of 3D printed microfluidic chip for cell processing. Microfluidics and Nanofluidics, vol. 20(5). https://doi.org/10.1007/s10404-015-1688-8
12. Cheng X Y, Li S J, Murr L E, et al., 2012, Compression deformation behavior of Ti-6A1-4V alloy with cellular structures fabricated by electron beam melting. Journal of the Mechanical Behavior of Biomedical Materials, vol. 16: 153-162.
https://doi.org/10.1016/j.jmbbm.2012.10.005
13. Sallica-Leva E, Jardini A L, and Fogagnolo J B, 2013, Microstructure and mechanical behavior of porous Ti-6Al-4V parts obtained by selective laser melting. Journal of the Mechanical Behavior of Biomedical Ma-terials, vol. 26: 98-108.
https://doi.org/10.1016/j.jmbbm.2013.05.011
14. Sing, S L, Yeong W Y, Wiria F E, et al., 2016, Characterization of Titanium Lattice Structures Fabricated by Selective Laser Melting Using an Adapted Compressive Test Method. Experimental Mechanics, vol. 56: 735-748.
https://doi.org/10.1007/s11340-015-0117-y
15. Sun, J F, Yang Y Q, and Wang D, 2013, Mechanical properties of a Ti6Al4V porous structure produced by selective laser melting. Materials & Design, vol. 49: 545-552. https://doi.org/10.1016/j.matdes.2013.01.038
16. Szymczyk P, Junka A, Ziolkowski G, et al., 2013, The ability of S.aureus to form biofilm on the TI-6Al-7Nb scaffolds produced by Selective Laser Melting and subje-cted to the different types of surface modifications. Acta of Bioengineering and Biomechanics, vol. 15(1): 69-76.
17. Yeong W Y, Yap C Y, Mapar M, et al., 2013, State-of- the-art review on selective laser melting of ceramics. High Value Manufacturing: Advanced Research in Virtual and Rapid Prototyping, 65-70.
18. Liu Z H, Zhang D Q, Sing S L, et al., 2014, Interfacial characterization of SLM parts in multi-material pro-cessing: Metallurgical diffusion between 316L stainless steel and C18400 copper alloy. Materials Characterization, vol. 94: 116-125.
https://doi.org/10.1016/j.matchar.2014.05.001
19. Sing S L, An J, Yeong W Y, et al., 2015, Laser and electron-beam powder-bed additive manufacturing of metallic implants: A review on processes, materials and designs. Journal of Orthopaedic Research, vol. 34(3): 369-385. https://doi.org/10.1002/jor.23075
20. Sing, S L, Yeong W Y, Chua C K, et al., 2013, Classical lamination theory applied on parts produced by selective laser melting in High Value Manufacturing: Advanced Research in Virtual and Rapid Prototyping, 77-82.
21. Thijs L, Sistiaga M L M, Wauthle R, et al., 2013, Strong morphological and crystallographic texture and resulting yield strength anisotropy in selective laser melted tantalum. Acta Materialia, vol. 61(12): 4657-4668.
https://doi.org/10.1016/j.actamat.2013.04.036
22. Yap C Y, Chua C K, and Dong Z L, 2016, An effective analytical model of selective laser melting. Virtual and Physical Prototyping, vol. 11(1): 21-26.
https://doi.org/10.1080/17452759.2015.1133217
23. Yap C Y, Chua C K, Dong Z L, et al., 2015, Review of selective laser melting: Materials and applications. Applied Physics Reviews, vol. 2(4): 041101.
https://doi.org/10.1063/1.4935926
24. Ciocca L, Fantini M, De Crescenzio F, et al., 2011, Direct metal laser sintering (DMLS) of a customized titanium mesh for prosthetically guided bone regeneration of atrophic maxillary arches. Medical & Biological En-gineering & Computer, vol. 49(11): 1347-1352.
https://doi.org/10.1007/s11517-011-0813-4
25. Li R, Liu J, Shi Y, et al., 2010, 316L Stainless Steel with Gradient Porosity Fabricated by Selective Laser Melting. Journal of Materials Engineering and Performance, vol. 19(5): 666-671.
https://doi.org/10.1007/s11665-009-9535-2
26. Yan C, Hao L, Hussein A, et al., 2014, Advanced light-weight 316L stainless steel cellular lattice structures fabricated via selective laser melting. Materials & Design, vol. 55: 533-541.
https://doi.org/10.1016/j.matdes.2013.10.027
27. Facchini L, Magalini E, Robotti P, et al., 2010, Ductility of a Ti-6Al-4V alloy produced by selective laser melting of prealloyed powders. Rapid Prototyping Journal, vol. 16(6): 450-459.
https://doi.org/10.1108/13552541011083371
28. Murr L E, Quinones S A, Gaytan S M, et al., 2009, Microstructure and mechanical behavior of Ti-6Al-4V produced by rapid-layer manufacturing, for biomedical applications. Journal of the Mechanical Behavior of Biomedical Materials, vol. 2(1): 20-32.
https://doi.org/10.1016/j.jmbbm.2008.05.004
29. Vrancken B, Thijs L, Kruth J P, et al., 2012, Heat treatment of Ti6Al4V produced by Selective Laser Melting: Microstructure and mechanical properties. Journal of Alloys and Compounds, vol. 541: 177-185.
https://doi.org/10.1016/j.jallcom.2012.07.022
30. Thijs L, Verhaeghe F, Craeghs T, et al., 2010, A study of the microstructural evolution during selective laser melting of Ti-6Al-4V. Acta Materialia, vol. 58(9): 3303- 3312. https://doi.org/10.1016/j.actamat.2010.02.004
31. Chlebus E, Kuznicka B, Kurzynowski T, et al., 2011, Microstructure and mechanical behaviour of Ti-6Al-7Nb alloy produced by selective laser melting. Materials Characterization, vol. 62(5): 488-495.
https://doi.org/10.1016/j.matchar.2011.03.006
32. Rotaru H, Armencea G, Spirchez D, et al., 2013, In vivo behavior of surface modified Ti6Al7Nb alloys used in selective laser melting for custom-made implants. A preliminary study. Romanian Journal of Morphology and Embryology, 54(3 SUPPL.): p. 791-796.
33. Sing S L, Yeong W Y, and Wiria F E, 2016, Selective laser melting of titanium alloy with 50 wt% tantalum: Microstructure and mechanical properties. Journal of Alloys and Compounds, vol. 660: 461-470.
https://doi.org/10.1016/j.jallcom.2015.11.141
34. Zhou YL, Niinomi M, and Akahori T, 2004, Effects of Ta content on Young's modulus and tensile properties of binary Ti-Ta alloys for biomedical applications. Ma-terials Science and Engineering A, vol. 371: 283-290.
https://doi.org/10.1016/j.msea.2003.12.011
35. Zhou Y L, Ninomi M, Akahori T, et al., 2007, Comparison of Various Properties between Titanium-Tantalum Alloy and Pure Titanium for Biomedical Applications. Materials Transactions, vol. 48(3): 380-384.
https://doi.org/10.2320/matertrans.48.380
36. Yousefi A M, Hoque M E, Prasad R G S V, et al., 2015, Current strategies in multiphasic scaffold design for osteochondral tissue engineering: A review. Journal of Biomedical Materials Research Part A, vol. 103(7): 2460-2481. https://doi.org/10.1002/jbm.a.35356
37. Duan X, Zhu X, Dong X, et al., 2013, Repair of large osteochondral defects in a beagle model with a novel type I collagen/glycosaminoglycan-porous titanium biphasic scaffold. Materials Science and Engineering C, vol. 33: 3951-3957.
https://doi.org/10.1016/j.msec.2013.05.040
38. Zhao C, Zhang H, Cai B, et al., 2012, Preparation of porous PLGA/Ti biphasic scaffold and osteochondral defect repair. Biomaterials Science, vol. 7(1): 703-710.
https://doi.org/10.1039/C3BM00199G
39. Nover A B, Lee S L, Georqescu M S, et al., 2015, Porous titanium bases for osteochondral tissue engineering. Acta Biomaterialia, vol. 27: 286-293.
https://doi.org/10.1016/j.actbio.2015.08.045
40. Zhao X, He J, Xu F, et al., 2016, Electrohydrodynamic printing: A potential tool for high-resolution hydrogel/cell patterning. Virtual and Physical Prototyping, vol. 11(1): 57-63.
https://doi.org/10.1080/17452759.2016.1139378
41. Getgood A M J, Kew S J, Brooks R, et al., 2012, Evaluation of early-stage osteochondral defect repair using a biphasic scaffold based on a collagen-glycosaminoglycan biopolymer in a caprine model. The Knee, vol. 19(4): 422-430.
https://doi.org/10.1016/j.knee.2011.03.011
42. Nemat-Nasser, S., Guo W G, and Cheng J Y, 1999, Mechanical properties and deformation mechanisms of a commercially pure titanium. Acta Materialia, vol. 47(13): 3705-3720.
https://doi.org/10.1016/S1359-6454(99)00203-7
43. Chichili D R, Ramesh K T, and Hemker K J, 1998, The high strain-rate response of alpha-titanium: Experiments, deformation mechanisms and modeling. Acta Materialia, vol. 46(3): 1025-1043.
https://doi.org/10.1016/S1359-6454(97)00287-5
44. Gurao N P, Kapoor R, and Suwas S, 2011, Deformation behavior of commerically pure titanium at extreme strain rates. Acta Materialia, vol. 59(9): 3431-3446.
https://doi.org/10.1016/j.actamat.2011.02.018
45. Zysset P K Z, Guo X E, Hoffler C E, et al., 1999, Elastic modulus and hardness of cortical and trabecular bone lamellae measured by nanoindentation in the human femur. Journal of Biomechanics, vol. 32(10): 1005-1012.
https://doi.org/10.1016/S0021-9290(99)00111-6
46. Piotrowski B, Baptista A A, Patoor E, et al., 2014, Interaction of bone-dental implant with new ultra low modulus alloy using a numerical approach. Material Science and Engineering: C, vol. 38: 151-160.
https://doi.org/10.1016/j.msec.2014.01.048
47. Traini T, Mangano C, Sammons R L, et al., 2008, Direct laser metal sintering as a new approach to fabrication of an isoelastic functionally graded material for manufacture of porous titanium dental implants. Dental Materials, vol. 24(11): 1525-1533.
https://doi.org/10.1016/j.dental.2008.03.029