3D bioprinting for tissue engineering: Stem cells in hydrogels
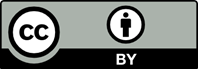
Surgical limitations require alternative methods of repairing and replacing diseased and damaged tissue. Regenerative medicine is a growing area of research with engineered tissues already being used successfully in patients. However, the demand for such tissues greatly outweighs the supply and a fast and accurate method of production is still required. 3D bioprinting offers precision control as well as the ability to incorporate biological cues and cells directly into the material as it is being fabricated. Having precise control over scaffold morphology and chemistry is a significant step towards controlling cellular behaviour, particularly where undifferentiated cells, i.e., stem cells, are used. This level of control in the early stages of tissue development is crucial in building more complex systems that morphologically and functionally mimic in vivo tissue. Here we review 3D printing hydrogel materials for tissue engineering purposes and the incorporation of cells within them. Hydrogels are ideal materials for cell culture. They are structurally similar to native extracellular matrix, have a high nutrient retention capacity, allow cells to migrate and can be formed under mild conditions. The techniques used to produce these materials, as well as their benefits and limitations, are outlined.
1. Hull CW, 1986, Apparatus for production of three-dimensional objects by stereolithography. US 4575330 A, Google Patents.
http://dx.doi.org/10.1007/s11427-015-4850-3.
2. Leigh SJ, Bradley RJ, Purssell CP et al. 2012, A Simple, Low-Cost Conductive Composite Material for 3D Printing of Electronic Sensors. PLoS ONE, vol. 7: e49365.
http://dx.doi.org/10.1371/journal.pone.0049365.
3. Berman B, 2012, 3-D printing: The new industrial revolution. Business Horizons, vol. 55: 155-162.
http://dx.doi.org/10.1016/j.bushor.2011.11.003.
4. Rengier F, Mehndiratta A, von Tengg-Kobligk H et al. 2010, 3D printing based on imaging data: review of medical applications. International Journal of Computer Assisted Radiology and Surgery, vol. 5: 335-341.
http://dx.doi.org/10.1007/s11548-010-0476-x.
5. Mehrban N, Bowen J, Vorndran E et al. 2013, Structural changes to resorbable calcium phosphate bioceramic aged in vitro. Colloids and Surfaces B: Biointerfaces, vol. 111: 469-478.
http://dx.doi.org/10.1016/j.colsurfb.2013.06.020.
6. Mehrban N, Paxton JZ, Bowen J et al 2011, Comparing physichochemical properties of printed and hand cast biocements designed for ligament replacement. Advances in Applied Ceramics, vol. 110: 162-167.
http://dx.doi.org/10.1179/1743676110Y.0000000012.
7. Ricci JL, Clark EA, Murriky A et al. 2012, Three-dimensional printing of bone repair and replacement materials: impact on craniofacial surgery. Journal of Craniofacial Surgery, vol. 23: 304-308.
http://dx.doi.org/10.1097/SCS.0b013e318241dc6e.
8. Tsang VL, Bhatia SN, 2004, Three-dimensional tissue fabrication. Advanced Drug Delivery Reviews, vol. 56: 1635-1647.
http://dx.doi.org/10.1016/j.addr.2004.05.001.
9. Mironov V, Boland T, Trusk T et al. 2003, Organ printing: computer-aided jet-based 3D tissue engineering. Trends in Biotechnology, vol. 21: 157-161.
http://dx.doi.org/10.1016/S0167-7799(03)00033-7.
10. Hollister SJ, 2005, Porous scaffold design for tissue engineering. Nature Materials, vol. 4: 518-524.
http://dx.doi.org/10.1038/nmat1421.
11. Seitz H, Rieder W, Irsen S et al. 2005, Three-dimensional printing of porous ceramic scaffolds for bone tissue engineering. Journal of Biomedical Materials Research Part B: Applied Biomaterials, vol. 74B: 782-788.
http://dx.doi.org/10.1002/jbm.b.30291.
12. Khademhosseini A, Langer R, Borenstein J et al. 2006, Microscale technologies for tissue engineering and biology. PNAS, vol. 103: 2480-2487.
http://dx.doi.org/10.1073/pnas.0507681102.
13. Murphy SV, Atala A, 2014, 3D bioprinting of tissues and organs. Nature Biotechnology, vol. 32: 773-785.
http://dx.doi.org/10.1038/nbt.2958.
14. Klebe RJ, 1988, Cytoscribing: a method for micropositioning cells and the construction of two- and three-dimensional synthetic tissues. Experimental Cell Research, vol. 179: 362-373.
http://dx.doi.org/10.1016/0014-4827(88)90275-3.
15. Singh M, Haverinen HM, Dhagat P et al. 2010, Inkjet printing- process and its applications. Advanced Materials, vol. 22: 673-685.
http://dx.doi.org/10.1002/adma.200901141.
16. Cui X, Dean D, Ruggeri ZM et al. 2010, Cell damage evaluation of thermal inkjet printed Chinese hamster ovary cells. Biotechnology and Bioengineering, vol. 106: 963-969.
http://dx.doi.org/10.1002/bit.22762.
17. Tasoglu S, Demirci U, 2013, Bioprinting for stem cell research. Trends in Biotechnology, vol. 31: 10-19.
http://dx.doi.org/10.1016/j.tibtech.2012.10.005.
18. Xu C, Zhang M, Huang Y et al. 2014, Study of droplet formation process during drop-on-demand inkjetting of living cell-laden bioink. Langmuir, vol. 30: 9130-9138.
http://dx.doi.org/10.1021/la501430x.
19. Demirci U, Montesano G, 2007, Single cell epitaxy by acoustic picolitre droplets. Lab on a Chip, vol. 7: 1139-1145.
http://dx.doi.org/10.1039/B704965J.
20. Derby B, 2012, Printing and prototyping of tissues and scaffolds. Science, vol. 338: 921-926.
http://dx.doi.org/10.1126/science.1226340.
21. Skardal A, Mack D, Kapetanovic E et al. 2012, Bioprinted amniotic fluid-derived stem cells accelerate healing of large skin wounds. Stem Cells Translational Medicine, vol. 1: 792-802.
http://dx.doi.org/10.5966/sctm.2012-0088.
22. Cui X, Breitenkamp K, Finn MG et al. 2012, Direct human cartilage repair using three-dimensional bioprinting technology. Tissue Engineering: Part A, vol. 18: 1304-1312.
http://dx.doi.org/10.1089/ten.TEA.2011.0543.
23. Duocastella M, Colina M, Fernández-Pradas JM et al. 2007, Study of the laser-induced forward transfer of liquids for laser bioprinting. Applied Surface Science, vol. 253: 7855-7859.
http://dx.doi.org/10.1016/j.apsusc.2007.02.097.
24. Mézel C, Souquet A, Hallo L et al. 2010, Bioprinting by laser-induced forward transfer for tissue engineering applications: jet formation modeling. Biofabrication, vol. 2: 014103.
http://dx.doi.org/10.1088/1758-5082/2/1/014103.
25. Ali M, Pages E, Ducom A et al. 2014, Controlling laser-induced jet formation for bioprinting mesenchymal stem cells with high viability and high resolution. Biofabrication, vol. 6: 045001.
http://dx.doi.org/10.1088/1758-5082/6/4/045001.
26. Hopp B, Smausz T, Krez N et al. 2005, Survival and proliferative ability of various living cell types after laser-induced forward transfer. Tissue Engineering, vol. 11: 1817-1823.
http://dx.doi.org/10.1089/ten.2005.11.1817.
27. Guillotin B, Souquet A, Catros S et al. 2010, Laser assisted bioprinting of engineered tissue with high cell density and microscale organization. Biomaterials, vol. 31: 7250-7256.
http://dx.doi.org/10.1016/j.biomaterials.2010.05.055.
28. Zopf DA, Hollister SJ, Nelson ME, 2013, Bioresorbable airway splint created with a three-dimensional printer. The New England Journal of Medicine, vol. 368: 2043-2045.
http://dx.doi.org/10.1056/NEJMc1206319.
29. Michael S, Sorg H, Peck C-T et al. 2013, Tissue engineered skin substitutes created by laser-assisted bioprinting form skin-like structures in the dorsal skin fold chamber in mice. PLoS ONE, vol. 8: e57741.
http://dx.doi.org/10.1371/journal.pone.0057741.
30. Keriquel V, Guillemot F, Arnault I et al. 2010, In vivo bioprinting for computer- and robotic-assisted medical intervention: preliminary study in mice. Biofabrication, vol. 2: 014101.
http://dx.doi.org/10.1088/1758-5082/2/1/014101.
31. Ragaert K, Cardon L, Dekeyser A et al. 2010, Machine design and processing considerations for the 3D plotting of thermoplastic scaffolds. Biofabrication, vol. 2: 014107.
http://dx.doi.org/10.1088/1758-5082/2/1/014107.
32. Zhang X, Zhang Y, 2015, Tissue engineering applications of three-dimensional bioprinting. Cell Biochemistry and Biophysics, vol. 72: 777-782.
http://dx.doi.org/10.1007/s12013-015-0531-x.
33. Malda J, Visser J, Melchels FP et al. 2013, 25th anniversary article: Engineering hydrogels for Biofabrication. Advanced Materials, vol. 25: 5011-5028.
http://dx.doi.org/10.1002/adma.201302042.
34. Tirella A, Vozzi F, Vozzi G et al. 2011, PAM2 (Piston Assisted Microsyringe): A new rapid prototyping technique for biofabrication of cell incorporated scaffolds. Tissue Engineering: Part C, vol. 17: 229-237.
http://dx.doi.org/10.1089/ten.tec.2010.0195.
35. DeSimone E, Schacht K, Jungst T et al. 2015, Biofabrication of 3D constructs: fabrication technologies and spider silk proteins as bioinks. Pure and Applied Chemistry, vol. 87: 737-749.
http://dx.doi.org/10.1515/pac-2015-0106.
36. Chang R, Nam J, Sun W, 2008, Effects of Dispensing Pressure and Nozzle Diameter on Cell Survival from Solid Freeform Fabrication–Based Direct Cell Writing. Tissue Engineering: Part A, vol. 14: 41-48.
http://dx.doi.org/10.1089/ten.a.2007.0004.
37. Khalil S, Nam J, Sun W, 2005, Multi-nozzle deposition for construction of 3D biopolymer tissue scaffolds. Rapid Prototyping Journal, vol. 11: 9-17.
http://dx.doi.org/10.1108/13552540510573347.
38. Jakab K, Norotte C, Marga F et al. 2010, Tissue engineering by self-assembly and bio-printing of living cells. Biofabrication, vol. 2: 022001.
http://dx.doi.org/10.1088/1758-5082/2/2/022001.
39. Duan B, Hockaday LA, Kang KH et al. 2013, 3D bioprinting of heterogeneous aortic valve conduits with alginate/gelatin hydrogels. Journal of Biomedical Materials Research A, vol. 101: 1255-1264.
http://dx.doi.org/10.1002/jbm.a.34420.
40. Chang R, Nam J, Sun W, 2008, Direct cell writing of 3D microorgan for in vitro pharmacokinetic model. Tissue Engineering Part C: Methods, vol. 14: 157-166.
http://dx.doi.org/10.1089/ten.tec.2007.0392.
41. Xu F, Celli J, Rizvi I et al. 2011, A three-dimensional in vitro ovarian cancer coculture model using a high-throughput cell patterning platform. Biotechnology Journal, vol. 6: 204-212.
http://dx.doi.org/10.1002/biot.201000340.
42. Melchels FPW, Feijen J, Grijpma DW, 2010, A review on stereolithography and its applications in biomedical engineering. Biomaterials, vol. 31: 6121-6130.
http://dx.doi.org/10.1016/j.biomaterials.2010.04.050.
43. Castro NJ, O’Brien J, Zhang LG, 2015, Integrating biologically inspired nanomaterials and table-top stereolithography for 3D printed biomimetic osteochondral scaffolds. Nanoscale, vol. 7: 14010.
http://dx.doi.org/10.1039/c5nr03425f.
44. Grogan SP, Chung PH, Soman P et al. 2013, Digital micromirror device projection printing system for meniscus tissue engineering. Acta Biomaterialia, vol. 9: 7218-7226.
45. http://dx.doi.org/10.1016/j.actbio.2013.03.020.Guo M, Qu X, Zhu W et al. 2014, Bio-inspired detoxification using 3D-printed hydrogel nanocomposites. Nature Communications, vol. 5: 3774.
http://dx.doi.org/10.1038/ncomms4774.
46. Dhariwala B, Hunt E, Boland T, 2014, Rapid prototyping of tissue-engineering constructs, using photopolymerisable hydrogels and stereolithography. Tissue Engineering, vol. 10: 1316-1322.
http://dx.doi.org/10.1089/ten.2004.10.1316.
47. Lee KY, Mooney DJ, 2001, Hydrogels for tissue engineering. Chemical Reviews, vol. 101: 1869-1879.
http://dx.doi.org/10.1021/cr000108x.
48. Hutmacher DW, Goh JC, Teoh SH, 2001, An introduction to biodegradable materials for tissue engineering applications. Annals of the Academy of Medicine, Singapore, vol. 30: 183-191.
49. Drury JL, Mooney DJ, 2003, Hydrogels for tissue engineering: scaffold design variables and applications. Biomaterials, vol. 24: 4337-4351.
http://dx.doi.org/10.1016/S0142-9612(03)00340-5.
50. O’Brien FJ, 2011, Biomaterials & scaffolds for tissue engineering. Materials Today, vol. 14: 88-95.
http://dx.doi.org/10.1016/S1369-7021(11)70058-X.
51. Iwasaki N, Yamane ST, Majima T et al. 2004, Feasibility of polysaccharide hybrid materials for scaffolds in cartilage tissue engineering: evaluation of chondrocyte adhesion to polyion complex fibers prepared from alginate and chitosan. Biomacromolecules, vol. 5: 828-833.
http://dx.doi.org/10.1021/bm0400067.
52. Costa VC, Costa HS, Vasconcelos WL et al. 2007, Preparation of hybrid biomaterials for bone tissue engineering. Materials Research, vol. 10: 21-26.
http://dx.doi.org/10.1590/S1516-14392007000100006.
53. Shah AA, Hasan F, Hameed A et al. 2008, Biological degradation of plastics: a comprehensive review. Biotechnology Advances, vol. 26: 246–265.
http://dx.doi.org/10.1016/j.biotechadv.2007.12.005.
54. Göpferich A, 1996, Mechanisms of polymer degradation and erosion. Biomaterials, vol. 17: 103–114.
http://dx.doi.org/10.1016/0142-9612(96)85755-3.
55. Salacinski HJ, Tai NR, Carson RJ et al. 2002, In vitro stability of a novel compliant poly(carbonate-urea)urethane to oxidative and hydrolytic stress. Journal of Biomedical Materials Research, vol. 59: 207–218.
http://dx.doi.org/10.1002/jbm.1234.
56. Steven MM, George GH, 2005, Exploring and engineering the cell surface interface. Science, vol. 18: 1135-1138.
http://dx.doi.org/10.1126/science.1106587.
57. Seidlits SK, Khaing ZZ, Petersen RR et al. 2010, The effects of hyaluronic acid hydrogels with tunable mechanical properties on neural progenitor cell differentiation. Biomaterials, vol. 31: 3930-3940.
http://dx.doi.org/10.1016/j.biomaterials.2010.01.125.
58. Murphy CM, Matsiko A, Haugh MG et al. 2012, Mesenchymal stem cell fate is regulated by the composition and mechanical properties of collagen-glycosaminoglycan scaffolds. Journal of the Mechanical Behaviour of Biomedical Materials, vol. 11: 53-62.
http://dx.doi.org/10.1016/j.jmbbm.2011.11.009.
59. Shim JH, Kim JY, Park M et al. 2011, Development of a hybrid scaffold with synthetic biomaterials and hydrogel using solid freeform fabrication technology. Biofabrication, vol. 3: 034102.
http://dx.doi.org/10.1088/1758-5082/3/3/034102.
60. Ahn SH, Kim YB, Lee HJ et al. 2012, A new hybrid scaffold constructed of solid freeform-fabricated PCL struts and collagen struts for bone tissue regeneration: fabrication, mechanical properties, and cellular activity. Journal of Materials Chemistry, vol. 22: 15901-15909.
http://dx.doi.org/10.1039/C2JM33310D.
61. Tan H, Marra KG, 2010, Injectable, biodegradable hydrogels for tissue engineering applications. Materials, vol. 3: 1746-1767.
http://dx.doi.org/10.3390/ma3031746.
62. Zhu J, Marchant RE, 2011, Design properties of hydrogel tissue-engineering scaffolds. Expert Review of Medical Devices, vol. 8: 607-626.
http://dx.doi.org/10.1586/erd.11.27.
63. HHunt JA, Chen R, van Veen T et al. 2014, Hydrogels for tissue engineering and regenerative medicine. Journal of Materials Chemistry B, vol. 2: 5319-5338.
http://dx.doi.org/10.1039/C4TB00775A.
64. Ahmed EM 2015, Hydrogel: Preparation, characterization, and applications: A review. Journal of Advanced Research, vol. 6: 105-121.
http://dx.doi.org/10.1016/j.jare.2013.07.006.
65. Skardal A, Atal A 2015, Biomaterials for integration with 3-D bioprinting. Annals of Biomedical Engineering, vol. 43: 730-746.
http://dx.doi.org/10.1007/s10439-014-1207-1.
66. Vu LT, Jain G, Veres BD et al. 2015, Cell migration on planar and three-dimensional matrices: a hydrogel-based perspective. Tissue Engineering Part B: Reviews, vol. 21: 67-74.
http://dx.doi.org/10.1089/ten.TEB.2013.0782.
67. Amsden B, 1998, Solute diffusion within hydrogels. Mechanisms and models. Macromolecules, vol. 31: 8382-8395.
http://dx.doi.org/10.1021/ma980765f.
68. Tan H, Li H, Rubin JP et al. 2011, Controlled gelation and degradation rates of injectable hyaluronic acid-based hydrogels through a double crosslinking strategy. Journal of Tissue Engineering and Regenerative Medicine, vol. 5: 790-797.
http://dx.doi.org/10.1002/term.378.
69. Müller M, Becher J, Schnabelrauch M et al. 2015, Nanostructured Pluronic hydrogels as bioinks for 3D bioprinting, vol. 7: 035006.
70. http://dx.doi.org/10.1088/1758-5090/7/3/035006.Barry RA, Shepherd RF, Hanson JN et al. 2009, Direct-write assembly of 3D hydrogel scaffolds for guided cell growth. Advanced Materials, vol. 21: 2407-2410.
http://dx.doi.org/10.1002/adma.200803702.
71. Cha C, Soman P, Zhu W et al. 2014, Structural reinforcement of cell-laden hydrogels with microfabricated three dimensional scaffolds. Biomaterials Science, vol. 1: 703-709.
http://dx.doi.org/10.1039/C3BM60210A.
72. Geckil H, Xu F, Zhang X et al. 2010, Engineering hydrogels as extracellular matrix mimics. Nanomedicine (London), vol. 5: 469-48.
http://dx.doi.org/10.2217/nnm.10.12.
73. Frantz C, Stewart KM, Weaver VM, 2010, The extracellular matrix at a glance, vol. 123: 4195-4200.
http://dx.doi.org/10.1242/jcs.023820.
74. Taubenberger AV, Woodruff MA, Bai H et al. 2010, The effect of unlocking RGD-motifs in collagen I on pre-osteoblast adhesion and differentiation. Biomaterials, vol. 31: 2827-2835.
http://dx.doi.org/10.1016/j.biomaterials.2009.12.051.
75. Galus R, Antiszko A, Wlodarski P, 2006, Clinical applications of hyaluronic acid. Polski Merkuriusz Lekarski, vol. 20: 606-608.
76. Skardal A, Zhang J, McCoard L et al. 2010, Photocrosslinkable hyaluronan-gelatin hydrogels for two-step bioprinting. Tissue Engineering: Part A, vol. 16: 2675-2685.
http://dx.doi.org/10.1089/ten.TEA.2009.0798.
77. Skardal A, Zhang J, McCoard L et al. 2010, Dynamically crosslinked gold nanoparticle-hyaluronan hydrogels. Advanced Materials, vol. 22: 4736-4740.
http://dx.doi.org/10.1002/adma.201001436.
78. Jackson MR, 2001, Fibrin sealants in surgical practice: An overview. The American Journal of Surgery, vol. 182: S1-S7.
http://dx.doi.org/10.1016/S0002-9610(01)00770-X.
79. Traver MA, Assimos DG, 2006, New generation tissue sealants and hemostatic agents: Innovative urologic applications. Reviews in Urology, vol. 8: 104-111.
80. Ahmed TA, Dare EV, Hincke M, 2008, Fibrin: a versatile scaffold for tissue engineering applications. Tissue Engineering Part B: Reviews, vol. 14: 199-215.
http://dx.doi.org/10.1089/ten.teb.2007.0435.
81. Masutani EM, Kinoshita CK, Tanaka TT et al. 2014, Increasing thermal stability of gelatin by UV-induced cross-linking with glucose. International Journal of Biomaterials, vol. 2014: Article ID 979636.
http://dx.doi.org/10.1155/2014/979636.
82. Sun J, Tan H, 2013, Alginate-based biomaterials for regenerative medicine applications. Materials, vol. 6: 1285-1309.
http://dx.doi.org/10.3390/ma6041285.
83. Duan B, Kapetanovic E, Hockaday LA et al. 2014, Three-dimensional printed trileaflet valve conduits using biological hydrogels and human valve interstitial cells. Acta Biomaterialia, vol. 10: 1836-1846.
http://dx.doi.org/10.1016/j.actbio.2013.12.005.
84. Kundu J, Shim J-H, Jang J et al. 2013, An Additive manufacturing-based PCL-alginate-chondrocyte bioprinted scaffold for cartilage tissue engineering. Journal of Tissue Engineering and Regenerative Medicine.
http://dx.doi.org/10.1002/term.1682.
85. Rutz AL, Hyland KE, Jakus AE et al. 2015, A multimaterial bioink method for 3D printing tunable, cell-compatible hydrogels. Advanced Materials, vol. 27: 1607-1614.
http://dx.doi.org/10.1002/adma.201405076.
86. Ozbolat IT, Chen H, Yu Y, 2014, Development of ‘multi-arm bioprinter’ for hybrid biofabrication of tissue engineered constructs. Robotics and Computer-Integrated Manufacturing, vol. 30: 295-304.
http://dx.doi.org/10.1016/j.rcim.2013.10.005.
87. Khalil S, Sun W, 2009, Bioprinting endothelial cells with alginate for 3D tissue constructs. Journal of Biomedical Engineering, vol. 131: 111002.
http://dx.doi.org/10.1115/1.3128729.
88. Nakamura M, Iwanaga S, Henmi C et al. 2010, Biomatrices and biomaterials for future developments of bioprinting and biofabrication. Biofabrication, vol. 2: 014110.
http://dx.doi.org/10.1088/1758-5082/2/1/014110.
89. Pati F, Jang J, Ha D-H et al. 2013, Printing three-dimensional tissue analogues with decellularized extracellular matrix bioink. Nature Communications, vol. 5: Article number 3935.
http://dx.doi.org/10.1038/ncomms4935.
90. Xu T, Jin J, Gregory C et al. 2005, Inkjet printing of viable mammalian cells. Biomaterials, vol. 26: 93-99.
http://dx.doi.org/10.1016/j.biomaterials.2004.04.011.
91. Kolesky DB, Truby RL, Gladman AS et al. 2014, 3D bioprinting of vascularized, heterogenous cell-laden tissue constructs. Advanced Materials, vol. 26: 3124-3130.
http://dx.doi.org/10.1002/adma.201305506.
92. Williams CG, Malik AN, Kim TK et al. 2005, Variable cytocompatibility of six cell lines with photoinitiators used for polymerizing hydrogels and cell encapsulation. Biomaterials, vol. 26: 1211-1218.
http://dx.doi.org/10.1016/j.biomaterials.2004.04.024.
93. Bianco P, Robey PG, 2001, Stem cells in tissue engineering. Nature, vol. 414: 118-121.
http://dx.doi.org/10.1038/35102181.
94. Gimble JM, Guilak F, 2003, Adipose-derived adult stem cells: isolation, characterization, and differentiation potential. Cytotherapy, vol. 5: 362-369.
http://dx.doi.org/10.1080/14653240310003026.
95. Winter A, Breit S, Parsch D et al. 2003, Cartilage-like gene expression in differentiated human stem cell spheroids: A comparison of bone marrow–derived and adipose tissue–derived stromal cells. Arthritis and Rheumatology, vol. 48: 418-429.
http://dx.doi.org/10.1002/art.10767.
96. Barry FP, Murphy JM, 2004, Mesenchymal stem cells: clinical applications and biological characterization. The International Journal of Biochemistry and Cell Biology, vol. 36: 568-584.
http://dx.doi.org/10.1016/j.biocel.2003.11.001.
97. Dalby MJ, Riehle MO, Johnstone H et al. 2002, In vitro reaction of endothelial cells to polymer demixed nanotopography. Biomaterials, vol. 23: 2945–2954.
http://dx.doi.org/10.1016/S0142-9612(01)00424-0.
98. Knoepfler PS, 2009, Deconstructing stem cell tumorigenicity: a roadmap to safe regenerative medicine. Stem cells, vol. 27: 1050–1056.
http://dx.doi.org/10.1002/stem.37.
99. Vats A, Tolley NS, Bishop AE, Polak JM, 2005, Embryonic stem cells and tissue engineering: delivering stem cells to the clinic. Journal of the Royal Society of Medicine, vol. 98: 346-350.
http://dx.doi.org/10.1258/jrsm.98.8.346.
100. Tsai CC, Su PF, Huang YF et al. 2012, Oct4 and Nanog directly regulate Dnmt1 to maintain self-renewal and undifferentiated state in mesenchymal stem cells. Molecular Cell, vol. 47: 169-182.
http://dx.doi.org/10.1016/j.molcel.2012.06.020.
101. Körbling M, Estrov Z, Champlin R, 2003, Adult stem cells and tissue repair. Bone Marrow Transplantation, vol. 32: S23-S24.
http://dx.doi.org/10.1038/sj.bmt.1703939.
102. Pittenger MF, Mackay AM, Beck SC et al. 1999, Multilineage potential of adult human mesenchymal stem cells. Science, vol. 284: 143–147.
http://dx.doi.org/10.1126/science.284.5411.143.
103. Sterodimas A, de Faria J, Nicaretta B et al. 2010, Tissue engineering with adipose-derived stem cells (ADSCs): Current and future applications. Journal of Plastic, Reconstructive & Aesthetic Surgery, vol. 63: 1886-1892.
http://dx.doi.org/10.1016/j.bjps.2009.10.028.
104. Zuk P 2013, Adipose-derived stem cells in tissue regeneration: A review. International Scholarly Research Notices, vol. 2013: Article ID 713959.
http://dx.doi.org/10.1155/2013/713959.
105. Takahashi K, Yamanaka S, 2006, Induction of pluripotent stem cells from mouse embryonic and adult fibroblast cultures by defined factors. Cell, vol. 126: 663-676.
http://dx.doi.org/10.1182/blood-2010-02-269589.
106. Wang A, Tang Z, Park I-H et al. 2011, Induced Pluripotent Stem Cells for Neural Tissue Engineering. Biomaterials, vol. 32: 5023-502.
http://dx.doi.org/10.1016/j.biomaterials.2011.03.070.
107. Diekman BO, Christoforou N, Willard VP et al. 2012, Cartilage tissue engineering using differentiated and purified induced pluripotent stem cells. PNAS, vol. 109: 19172-19177.
http://dx.doi.org/10.1073/pnas.1210422109.
108. De Peppo GM, Marcos-Campos I, Kahler DJ et al. Engineering bone tissue substitutes from human induced pluripotent stem cells. PNAS, vol. 110: 8680-8685.
http://dx.doi.org/10.1073/pnas.1301190110.
109. Cho H-J, Lee C-S, Kwon Y-W et al. 2010, Induction of pluripotent stem cells from adult somatic cells by protein-based reprogramming without genetic manipulation. Blood, vol. 116: 386-395.
http://dx.doi.org/10.1182/blood-2010-02-269589.
110. Peerani R, Rao BM, Bauwens C et al. 2007, Niche-mediated control of human embryonic stem cell self-renewal and differentiation. The EMBO Journal, vol. 26: 4744-4755.
http://dx.doi.org/10.1038/sj.emboj.7601896
111. Park J, Cho CH, Parashurama N et al. 2007, Microfabrication-based modulation of embryonic stem cell differentiation.Lab on a Chip, vol. 8:1018-1028.
http://dx.doi.org/10.1039/B704739H.
112. Raof NA, Schiele NR, Xie Y et al. 2011, The maintenance of pluripotency following laser direct-write of mouse embryonic stem cells, vol. 32: 1802-1808.
http://dx.doi.org/10.1016/j.biomaterials.2010.11.015.
113. Gruene M, Deiwick A, Koch L et al. 2011, Laser printing of stem cells for biofabrication of scaffold-free autologous grafts. Tissue Engineering Part C: Methods, vol. 17: 79–87.
http://dx.doi.org/10.1089/ten.tec.2010.0359.
114. Le Visage C, Dunham B, Flint P et al. 2004 Coculture of mesenchymal stem cells and respiratory epithelial cells to engineer a human composite respiratory mucosa. Tissue Engineering, vol. 10: 1426–1435.
http://dx.doi.org/10.1089/ten.2004.10.1426.
115. Gaebel R, Ma N, Liu J et al. 2011, Patterning human stem cells and endothelial cells with laser printing for cardiac regeneration. Biomaterials, vol. 32: 9218–9230.
http://dx.doi.org/10.1016/j.biomaterials.2011.08.071.
116. Teoh GZ, Crowley C, Birchall MA et al. 2015, Development of resorbable nanocomposite tracheal and bronchial scaffolds for paediatric applications. British Journal of Surgery, vol. 102: e140–e150.
http://dx.doi.org/10.1002/bjs.9700.
117. Wang S, Lee JM, Yeong WY, 2015, Smart hydrogels for 3D bioprinting. International Journal of Bioprinting, vol. 1: 3-14.
http://dx.doi.org/10.18063/IJB.2015.01.005.
118. Seras-Franzoso J, Tsimbouri PM, Burgess KV et al. 2014, Topographically targeted osteogenesis of mesenchymal stem cells stimulated by inclusion bodies attached to polycaprolactone surfaces. Nanomedicine, vol. 9: 207–220.
http://dx.doi.org/10.2217/nnm.13.43.
119. Gruene M, Pflaum M, Deiwick A et al. 2011 Adipogenic differentiation of laser-printed 3D tissue grafts consisting of human adipose-derived stem cells. Biofabrication, vol. 3: 015005.
http://dx.doi.org/10.1088/1758-5082/3/1/015005..
120. Gao G, Yonezawa T, Hubbell K et al. 2015, Inkjet-bioprinted acrylated peptides and PEG hydrogel with human mesenchymal stem cells promote robust bone and cartilage formation with minimal printhead clogging. Biotechnology Journal, vol. 10: 1568-1577.
http://dx.doi.org/10.1002/biot.201400635.
121. Holmes B and Zhang LG 2013, Enahnced human bone marrow mesenchymal stem cell functions in 3D bioprinted biologically inspired osteochondral construct. The American Society of Chemical Engineers Proceedings, vol. 3A: V03AT03A002.
http://dx.doi.org/10.1115/IMECE2013-66118.
122. Lee V, Singh G, Tarasatti JP et al. 2014, Design and fabrication of human skin by three-dimensional bioprinting. Tissue Engineering Part C: Methods, vol. 20: 473-484.
http://dx.doi.org/10.1089/ten.TEC.2013.0335.
123. Censi R, Schuurman W, Malda J et al. 2011, A printable photopolymerizable thermosensitive p(HPMAm-lactate)-PEG hydrogel for tissue engineering. Advanced Functional Materials, vol. 21: 1833-1842.
http://dx.doi.org/10.1002/adfm.201002428.
124. Jackson N, Stam F, 2015, Optimization of electrical stimulation parameters for electro-responsive hydrogels for biomedical applications. Journal of Applied Polymer Science, vol. 132: app. 41687.
http://dx.doi.org/10.1002/app.41687.
125. Giani G, Fedi S, Barbucci R, 2012, Hybrid magnetic hydrogel: A potential system for controlled drug delivery by means of alternating magnetic fields. Polymers, vol. 4: 1157-1169.
http://dx.doi.org/10.3390/polym4021157.
126. Pati F, Jang J, Ha D-H et al. 2014, Printing three-dimensional tissue analogues with decellularized extracellular matrix bioink. Nature Communications, vol. 5: Article Number 3935.
http://dx.doi.org/10.1038/ncomms4935.