Investigation of process parameters of electrohydrodynamic jetting for 3D printed PCL fibrous scaffolds with complex geometries
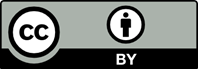
Tissue engineering is a promising technology in the field of regenerative medicine with its potential to create tissues de novo. Though there has been a good progress in this field so far, there still exists the challenge of providing a 3D micro-architecture to the artificial tissue construct, to mimic the native cell or tissue environment. Both 3D printing and 3D bioprinting are looked upon as an excellent solution due to their capabilities of mimicking the native tissue architecture layer-by-layer with high precision and appreciable resolution. Electrohydrodynamic jetting (E-jetting) is one type of 3D printing, in which, a high electric voltage is applied between the extruding nozzle and the substrate in order to print highly controlled fibres. In this study, an E-jetting system was developed in-house for the purpose of 3D printing of fibrous scaffolds. The effect of various E-jetting parameters, namely the supply voltage, solution concentration, nozzle-to-substrate distance, stage (printing) speed and solution dispensing feed rate on the diameter of printed fibres were studied at the first stage. Optimized parameters were then used to print Polycaprolactone (PCL) scaffolds of highly complex geometries, i.e., semi-lunar and spiral geometries, with the aim of demonstrating the flexibility and capability of the system to fabricate complex geometry scaffolds and biomimic the complex 3D micro-architecture of native tissue environment. The spiral geometry is expected to result in better cell migration during cell culture and tissue maturation.
1. Hutmacher D W, 2001, Scaffold design and fabrication technologies for engineering tissues—state of the art and
future perspectives. Journal of Biomaterials Science, Polymer Edition, vol.12(1): 107–124. http://dx.doi.org/10.1163/156856201744489
2. Hutmacher D W, Schantz T, Zein I, et al. 2001, Mechanical properties and cell cultural response of polycaprolactone scaffolds designed and fabricated via fused deposition modelling. Journal of Biomedical Materials Research, vol.55(2): 203–216. http://dx.doi.org/10.1002/1097-4636(200105)55:23.0.CO;2-7
3. Cukierman E, Pankov R, Stevens D R, et al. 2001, Taking cell-matrix adhesions to the third dimension. Science, vol.294(5547): 1708–1712. http://dx.doi.org/10.1126/science.1064829
4. Cukierman E, Pankov R and Yamada K M, 2002, Cell interactions with three-dimensional matrices. Current Opinion in Cell Biology, vol.14(5): 633–640. http://dx.doi.org/10.1016/S0955-0674(02)00364-2
5. Edelman D B and Keefer E W, 2005, A cultural renaissance: in vitro cell biology embraces three- dimensional context. Experimental Neurology, vol.192(1): 1–6. http://dx.doi.org/10.1016/j.expneurol.2004.10.005
6. Sachlos E and Czernuszka J T, 2003, Making tissue engineering scaffold work: Review on the application of SFF technology to the production of tissue engineering scaffolds. European Cells and Materials, vol.5(1): 29–40.
7. Whitesides G M, Ostuni E, Takayama S, et al. 2001, Soft lithography in biology and biochemistry. Annual Review of Biomedical Engineering, vol.3(1): 335–373. http://dx.doi.org/10.1146/annurev.bioeng.3.1.335
8. Walker G M, Zeringue H C and Beebe D J, 2004, Microenvironment design considerations for cellular scale studies. Lab on a Chip, vol.4(2): 91–97. http://dx.doi.org/10.1039/b311214d
9. Khademhosseini A, Langer R, Borenstein J, et al. 2006, Microscale technologies for tissue engineering and biology. Proceedings of the National Academy of Sciences of the United States of America, vol.103(8): 2480–2487. http://dx.doi.org/10.1073/pnas.0507681102
10. Lannutti J, Reneker D,Ma T, et al. 2007, Electrospinning for tissue engineering scaffolds. Materials Science and Engineering: C, vol.27(3): 504–509. http://dx.doi.org/10.1016/j.msec.2006.05.019
11. Agarwal S, Wendorff J H and Greiner A, 2009, Progress in the field of electrospinning for tissue engineering applications. Advanced Materials, vol.21(32–33): 3343– 3351. http://dx.doi.org/10.1002/adma.200803092
12. LiW J, Laurencin C T, Caterson E J, et al. 2002, Elec-trospun nanofibrous structure: A novel scaffold for tissue engineering. Journal of Biomedical Materials Research, vol.60(4): 613–621. http://dx.doi.org/10.1002/jbm.10167
13. Sill T J and von RecumH A, 2008, Electrospinning: Applications in drug delivery and tissue engineering. Biomaterials, vol.29(13): 1989–2006. http://dx.doi.org/10.1016/j.biomaterials.2008.01.011
14. Yang F,Murugan R,Wang S, et al. 2005, Electrospinning of nano/micro scale poly (L-lactic acid) aligned fibers and their potential in neural tissue engineering. Biomate-rials, vol.26(15): 2603–2610. http://dx.doi.org/10.1016/j.biomaterials.2004.06.051
15. Richards D J, Tan Y, Jia J, et al. 2013, 3D printing for tissue engineering. Israel Journal of Chemistry, vol.53(9–10): 805–814. http://dx.doi.org/10.1002/ijch.201300086
16. Mironov V, Boland T, Trusk T, et al. 2003, Organ printing: Computer-aided jet-based 3D tissue engineering. Trends in Biotechnology, vol.21(4): 157–161. http://dx.doi.org/0.1016/S0167-7799(03)00033-7
17. Hollister S J, 2005, Porous scaffold design for tissue engineering. Nature Materials, vol.4(7): 518–524. http://dx.doi.org/10.1038/nmat1421
18. Boland T, Xu T, Damon B, et al. 2006, Application of inkjet printing to tissue engineering. Biotechnology Journal, vol.1(9): 910–917. http://dx.doi.org/10.1002/biot.200600081
19. YeongW Y, ChuaC K, LeongK F, et al. 2004, Rapid prototyping in tissue engineering: Challenges and potential. Trends in Biotechnology, vol.22(12): 643–652. http://dx.doi.org/10.1016/j.tibtech.2004.10.004
20. An J, Teoh J E M, Suntornnond R, et al. 2015, Design and 3D printing of scaffolds and tissues. Engineering, vol.1(2), 261–268. http://dx.doi.org/10.15302/J-ENG-2015061
21. Gupta A, Seifalian A M, Ahmad Z, et al. 2007, Novel electrohydrodynamic printing of nanocomposite biopolymer scaffolds. Journal of Bioactive and Compatible Polymers, vol.22(3), 265–280. http://dx.doi.org/10.1177/0883911507078268
22. Wei C and Dong J, 2013, Direct fabrication of high-resolution three-dimensional polymeric scaffolds using electrohydrodynamic hot jet plotting. Journal of Microme-chanics and Microengineering, vol.23(2): 025017. http://dx.doi.org/10.1088/0960-1317/23/2/025017
23. Ahmad Z, Rasekh M and Edirisinghe M, 2010, Electrohydrodynamic direct writing of biomedical polymers and composites. Macromolecular Materials and Engineering, vol.295(4): 315–319. http://dx.doi.org/10.1002/mame.200900396
24. Li J L, Cai Y L, Guo Y L, et al. 2014, Fabrication of three-dimensional porous scaffolds with controlled filament orientation and large pore size via an improved E-jetting technique. Journal of Biomedical Materials Research Part B: Applied Biomaterials, vol.102(4): 651–658. http://dx.doi.org/10.1002/jbm.b.33043
25. Gasperini L, Maniglio D, Motta A, et al. 2014, An electrohydrodynamic bioprinter for alginate hydrogels containing living cells. Tissue Engineering Part C: Methods, vol.21(2): 123–132. http://dx.doi.org/10.1089/ten.TEC.2014.0149
26. Cai Y, Li J L, Poh C K, et al. 2013, Collagen grafted 3D polycaprolactone scaffolds for enhanced cartilage regeneration. Journal of Materials Chemistry B, vol.1(43): 5971–5976. http://dx.doi.org/0.1039/C3TB20680G
27. Doshi J and Reneker D H, 1993, Electrospinning process and applications of electrospun fibers, In Industry Applications Society Annual Meeting, Conference Record of the 1993 IEEE: 1698–1703.
28. Mitchell G R, AhnK H and Davis F J, 2011, The potential of electrospinning in rapid manufacturing processes. Virtual and Physical Prototyping, vol.6(2): 63–77. http://dx.doi.org/10.1080/17452759.2011.590387
29. Thompson C J, Chase G G, Yarin A L, et al. 2007, Effects of parameters on nanofiber diameter determined from electrospinning model. Polymer, vol.48(23): 6913– 6922. http://dx.doi.org/10.1016/j.polymer.2007.09.017
30. Bu N, Huang Y, Wang X, et al. 2012, Continuously tunable and oriented nanofiber direct-written by mechano-electrospinning. Materials and Manufacturing Processes, vol.27(12): 1318–1323. http://dx.doi.org/10.1080/10426914.2012.700145
31. Chanthakulchan A, Koomsap P, Auyson K, et al. 2015, Development of an electrospinning-based rapid proto-typing for scaffold fabrication. Rapid Prototyping Jour-nal, vol.21(3): 329–339. http://dx.doi.org/10.1108/RPJ-11-2013-0119
32. Auyson K, Koomsap P, Chanthakulchan A, et al. 2013, Investigation of applying electrospinning in fused deposition modeling for scaffold fabrication. In High Value Manufacturing: Advanced Research in Virtual and Rapid Prototyping, Proceedings of the 6th International Conference on Advanced Research in Virtual and Rapid Prototyping, CRC Press: 149.
33. Bisht G S, Canton G, Mirsepassi A, et al. 2011, Controlled continuous patterning of polymeric nanofibers on three-dimensional substrates using low-voltage near-field Electrospinning. Nano Letters, vol.11(4): 1831–1837. http://dx.doi.org/10.1021/nl2006164
34. Chang C, Limkrailassiri K and Lin L, 2008, Continuous near-field electrospinning for large area deposition of orderly nanofiber patterns. Applied Physics Letters, vol.93(12): 123111. http://dx.doi.org/10.1063/1.2975834
35. Li J L, Guo Y L, Thian E S, et al. 2013, 3-Dimensional meniscal fibrillar scaffolds, apparatus and process for the fabrication thereof, UK Patent filing, 2013. Application No. 1315074.3