The effect of manufacturing method; direct compression, hot-melt extrusion, and 3D printing on polymer stability and drug release from polyethylene oxide tablets
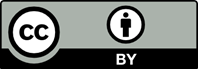
Thermal 3D printing has gained substantial attention in pharmaceutical formulation, especially concerning its potential use in personalized dose delivery. The choice of a printable polymer is crucial in this technique, but it is restricted due to technical issues such as thermal stability and thermal-rheological properties of the polymers. Polyethylene oxide (PEO) is a widely used polymer in drug formulation designs, with potential application in 3D printing due to its favorable rheological properties. However, the thermal stability of PEOs exposed to high temperatures during fused deposition modeling (FDM) needs to be characterized. This research focused on the characterization of two molecular weights (Mw) of PEO (7 and 0.9 M) under various manufacturing methods and formulation compositions. PEO was mixed with other low-viscosity polymers of hydroxypropyl cellulose (HPC) or ethyl cellulose (EC) to achieve printable formulations (PEO/HPC or PEO/EC). Tablets were manufactured by direct compression, compression of hot-melt extrudates (HME) at 150°, or by FDM 3D-printing at 220°. Differential scanning calorimetry (DSC), X-ray powder diffraction (XRPD), gel permeation chromatography (GPC), dissolution tests, and their kinetics studies were carried out. Results demonstrated that thermal processes could reduce the crystallinity of PEO and induce Mw reduction that varies depending on the Mw of PEO. As a result, dissolution efficiency (DE%) varied based on the formulation composition and manufacturing method. For formulations containing PEO and HPC, 3D-printed and HME tablets exhibited higher DE (>60%) compared to directly compressed tablets (DE < 50%), while for those with PEO and EC, 3D printing reduced DE% to <26% compared to direct compression (~30%) and HME tablets (~50%). This was attributed to the hydrophobic nature of EC and the increased hardness of the printed tablets, preventing tablet disintegration during dissolution, which outweighs the Mw reduction in PEO.
- Salem S, Byrn SR, Smith DT, et al. Impact assessment of the variables affecting the drug release and extraction of polyethylene oxide based tablets. J Drug Deliv Sci Technol. 2022;71:103337. doi: 10.1016/j.jddst.2022.103337
- Meruva S, Donovan MD. Polyethylene oxide (PEO) molecular weight effects on abuse-deterrent properties of matrix tablets. AAPS PharmSciTech. 2020;21: 1-10. doi: 10.1208/s12249-019-1565-y
- Pajander J, Rensonnet A, Hietala S, et al. The evaluation of physical properties of injection molded systems based on poly(ethylene oxide) (PEO). Int J Pharm. 2017;518 (1–2):203-212. doi: 10.1016/j.ijpharm. 2016.12.050
- Maggi L, Bruni R, Conte U. High molecular weight polyethylene oxides (PEOs) as an alternative to HPMC in controlled release dosage forms. Int J Pharm. 2000;195 (1–2):229-238. doi: 10.1016/S0378-5173(99)00402-0
- Malik P, Castro M, Carrot C. Thermal degradation during melt processing of poly(ethylene oxide), poly(vinylidenefluoride-co-hexafluoropropylene) and their blends in the presence of additives, for conducting applications. Polym Degrad Stab. 2006;91(4):634-640. doi: 10.1016/j.polymdegradstab.2005.01.020
- Crowley MM, Zhang F, Koleng JJ, et al. Stability of polyethylene oxide in matrix tablets prepared by hot-melt extrusion. Biomaterials. 2002;23(21):4241-428. doi: 10.1016/S0142-9612(02)00187-4
- Cantin O. PEO Hot Melt Extrudates for Controlled Drug Delivery (Doctoral dissertation, Université du Droit et de la Santé–Lille II). 2017. https://tel.archives-ouvertes.fr/tel-01540630
- Shojaee S, Asare-Addo K, Kaialy W, et al. An investigation into the stabilization of diltiazem HCl release from matrices made from aged polyox powders. AAPS PharmSciTech. 2013;14(3):1190-1198. doi: 10.1208/s12249-013-0013-7
- Shojaee S, Cumming I, Kaialy W, et al. The influence of vitamin E succinate on the stability of polyethylene oxide PEO controlled release matrix tablets. Colloids Surf B Biointerfaces. 2013;111:486-492. doi: 10.1016/j.colsurfb.2013.06.038
- Vrandečić NS, Erceg M, Jakić M, et al. Kinetic analysis of thermal degradation of poly(ethylene glycol) and poly(ethylene oxide)s of different molecular weight. Thermochim Acta. 2010;498(1–2):71-80. doi: 10.1016/j.tca.2009.10.005
- Smith KL, Van Cleve R. High molecular weight polymers of ethylene oxide plastic properties. Indus Eng Chem. 1958;50(1):12-16. doi: 10.1021/ie50577a024
- Repka MA, McGinity JW. Influence of vitamin E TPGS on the properties of hydrophilic films produced by hot-melt extrusion. Int J Pharm. 2000;202(1–2):63-70. doi: 10.1016/S0378-5173(00)00418-X
- Cantin O, Siepmann F, Danede F, et al. PEO hot melt extrudates for controlled drug delivery: importance of the molecular weight. J Drug Deliv Sci Technol. 2016;36:130-140. doi: 10.1016/j.jddst.2016.09.003
- Isreb A, Baj K, Wojsz M, et al. 3D printed oral theophylline doses with innovative ‘radiator-like’ design: impact of polyethylene oxide (PEO) molecular weight. Int J Pharm. 2019;564:98-105. doi: 10.1016/j.ijpharm.2019.04.017
- Ong JJ, Awad A, Martorana A, et al. 3D printed opioid medicines with alcohol-resistant and abuse-deterrent properties. Int J Pharm. 2020;579:119169. doi: 10.1016/j.ijpharm.2020.119169
- Nashed N, Lam M, Ghafourian T, et al. An insight into the impact of thermal process on dissolution profile and physical characteristics of theophylline tablets made through 3D printing compared to conventional methods. Biomedicines. 2022;10(6):1-18. doi: 10.3390/biomedicines10061335
- Quinten T. Evaluation of injection molding as a pharmaceutical production technology for sustained-release matrix tablets. Ghent University. 2010. http://library1.nida.ac.th/termpaper6/sd/2554/19755.pdf
- Shodexhplc, Poly (Ethylene Oxide) Standards. Available from: shodexhplc.com/applications/poly-ethylene-oxide-standards/
- Stringano E, Gea A, Salminen JP, et al. Simple solution for a complex problem: proanthocyanidins, galloyl glucoses and ellagitannins fit on a single calibration curve in high performance-gel permeation chromatography. J Chromatogr A. 2011;1218(43):7804-7812. doi: 10.1016/j.chroma.2011.08.082
- Dishman KL. Sieving in particle size analysis. In: Encyclopedia of Analytical Chemistry. Wiley; 2000. doi: 10.1002/9780470027318.a1514.
- Tan DK, Maniruzzaman M, Nokhodchi A. Development and optimisation of novel polymeric compositions for sustained release theophylline caplets (PrintCap) via FDM 3D printing. Polymers. 2020;12(1):1-18. doi: 10.3390/polym12010027
- Anderson NH, Bauer M, Boussac N, et al. An evaluation of fit factors and dissolution efficiency for the comparison of in vitro dissolution profiles. J Pharm Biomed Anal. 1998;17 (4–5):811-822. doi: 10.1016/S0731-7085(98)00011-9
- Zhang Y, Huo M, Zhou J, et al. DDSolver: an add-in program for modeling and comparison of drug dissolution profiles. AAPS J. 2010;12(3):263-271. doi: 10.1208/s12248-010-9185-1
- Ritger PL, Peppas NA. A simple equation for description of solute release I. Fickian and non-fickian release from non-swellable devices in the form of slabs, spheres, cylinders or discs. J Control Release. 1987;5(1):23-36. doi: 10.1016/0168-3659(87)90034-4
- Bruschi ML. Mathematical models of drug release. In: Strategies to Modify the Drug Release from Pharmaceutical Systems. Sawston, UK: Woodhead Publishing; 2015:63-86. doi: 10.1016/B978-0-08-100092-2.00005-9
- Shi K, Slavage JP, Maniruzzaman M, et al. Role of release modifiers to modulate drug release from fused deposition modelling (FDM) 3D printed tablets. Int J Pharm. 2021;597:120315. doi: 10.1016/j.ijpharm.2021.120315
- Ashland Inc. Klucel Hydroxypropylcellulose Physical and Chemical Properties (Datasheet). 2017. http://www.ashland.com/file_source/Ashland/Product/ Documents/Pharmaceutical/PC_11229_Klucel_HPC.pdf
- Homaee Borujeni S, Mirdamadian SZ, Varshosaz J, et al. Three-dimensional (3D) printed tablets using ethyl cellulose and hydroxypropyl cellulose to achieve zero order sustained release profile. Cellulose. 2020;27(3):1573–1589. doi: 10.1007/s10570-019-02881-4
- Polaskova M, Peer P, Cermak R, et al. Effect of thermal treatment on crystallinity of poly(ethylene oxide) electrospun fibers. Polymers. 2019;11(9):1384. doi: 10.3390/polym11091384
- PerkinElmer. Polymer crystallinity studies by DSC- Raman spectroscopy. 2009. https://perkinelmer.cl/wp-content/uploads/2018/05/ Polymer-Crystallinity-by-DSC-Raman.pdf
- Park MS, Kim JK. Phase behavior and crystallization of a poly(ethylene oxide)/cellulose acetate butyrate blend. J Polym Sci B Polym Phys. 2002;40(15):1673-1681. doi: 10.1002/polb.10225
- Leineweber A. Reflection splitting-induced microstrain broadening. Powder Diffraction. 2017;32(S1):S35-S39. doi: 10.1017/S0885715617000665
- Bhagia S, Gallego NC, Hiremath N, et al. Fine grinding of thermoplastics by high speed friction grinding assisted by guar gum. J Appl Polym Sci. 2021;138(32):50797. doi: 10.1002/app.50797
- Schmidt J, Plata M, Tröger S, et al. Production of polymer particles below 5μm by wet grinding. Powder Technol. 2012;228:84-90. doi: 10.1016/j.powtec.2012.04.064.
- Jung H, Lee YJ, Yoon WB. Effect of moisture content on the grinding process and powder properties in food: a review. Processes. 2018;6(6):69. doi: 10.3390/pr6060069
- Eckert A, Abbasi M, Mang T, et al. Structure, mechanical properties, and dynamics of polyethylenoxide/nanoclay nacre-mimetic nanocomposites. Macromolecules. 2020;53(5):1716-1725. doi: 10.1021/acs.macromol.9b01931
- Xiao P, Guo Y, Wang J, et al. The effect of granules characters on mechanical properties of press-coated tablets: a comparative study. Int J Pharm. 2022;624:121986. doi: 10.1016/j.ijpharm.2022.121986
- Vinayagamoorthy R. Effect of particle sizes on the mechanical behaviour of limestone-reinforced hybrid plastics. Polym Polym Compos. 2020;28(6): 410-420. doi: 10.1177/0967391119883163
- Huang Y, Paul DR. Effect of temperature on physical aging of thin glassy polymer films. Macromolecules. 2005;38(24):10148-1054. doi: 10.1021/ma051284g
- Greiner R, Schwarzl FR. Volume relaxation and physical aging of amorphous polymers I. theory of volume relaxation after single temperature jumps. Colloid Polym Sci. 1989;267(1):39-47. doi: 10.1007/BF01410147
- Zhang J, Feng X, Patil H, et al. Coupling 3D printing with hot-melt extrusion to produce controlled-release tablets. Int J Pharm. 2016;519:186-197. doi: 10.1016/j.ijpharm.2016.12.049