Advancements in 3D bioprinting for precision medicine: Enhancing patient-derived organoids and extracellular vesicle applications in inflammatory diseases
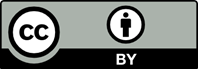
The integration of three-dimensional (3D) bioprinting with cultures of patient-derived organoids represents a transformative advancement in precision medicine, enabling the creation of anatomically accurate and physiologically relevant tissue models. These models are pivotal for personalized disease modeling, therapeutic development, and regenerative medicine. 3D bioprinting enhances the structural and functional fidelity of organoids, thereby improving their application in drug screening and disease intervention strategies. Additionally, this technology facilitates the study of extracellular vesicles (EVs) released from patient-derived organoids, which are rich in bioactive molecules and play crucial roles in modulating immune responses. Particularly in chronic inflammatory conditions, these organoid-derived EVs are instrumental in cellular communication and improve our understanding of disease mechanisms beyond traditional biopsies. This review focuses on the latest developments in 3D bioprinting techniques for organoids and EVs, highlighting their potential to revolutionize the treatment of inflammatory diseases through precision medicine.

- Karami Fath M, Azami J, Jaafari N, et al. Exosome application in treatment and diagnosis of B-cell disorders: leukemias, multiple sclerosis, and arthritis rheumatoid. Cell Mol Biol Lett. 2022;27(1):74. doi: 10.1186/s11658-022-00377-x
- Mozdiak E, O’Malley JW, Arasaradnam RP. Inflammatory bowel disease. BMJ. 2015;351:h4416. doi: 10.1136/bmj.h4416
- Zhao Z, Chen X, Dowbaj AM, et al. Organoids. Nat Rev Methods Primers. 2022;2(1):94. doi: 10.1038/s43586-022-00174-y
- Lancaster MA, Knoblich JA. Organogenesis in a dish: modeling development and disease using organoid technologies. Science. 2014:345(6194):1247125. doi: 10.1126/science.1247125
- Grassi L, Alfonsi R, Francescangeli F, et al. Organoids as a new model for improving regenerative medicine and cancer personalized therapy in renal diseases. Cell Death Dis. 2019;10(3):201. doi: 10.1038/s41419-019-1453-0
- Pauli C, Hopkins BD, Prandi D, et al. Personalized in vitro and in vivo cancer models to guide precision medicine. Cancer Discov. 2017;7(5):462-477. doi: 10.1158/2159-8290.Cd-16-1154
- Zhang Y, Li G, Wang J, Zhou F, Ren X, Su J. Small joint organoids 3D bioprinting: construction strategy and application. Small. 2024;20(8):e2302506. doi: 10.1002/smll.202302506
- Datta P, Dey M, Ataie Z, Unutmaz D, Ozbolat IT. 3D bioprinting for reconstituting the cancer microenvironment. NPJ Precis Oncol. 2020; 4:18. doi: 10.1038/s41698-020-0121-2
- Augustine R, Kalva SN, Ahmad R, et al. 3D bioprinted cancer models: revolutionizing personalized cancer therapy. Transl Oncol. 2021;14(4):101015. doi: 10.1016/j.tranon.2021.101015
- Zhang J, Wehrle E, Rubert M, Müller R. 3D bioprinting of human tissues: biofabrication, bioinks, and bioreactors. Int J Mol Sci. 2021;22(8):3971. doi: 10.3390/ijms22083971
- Dey M, Ozbolat IT. 3D bioprinting of cells, tissues and organs. Sci Rep. 2020;10(1):14023. doi: 10.1038/s41598-020-70086-y
- Gopalakrishnan S, Bakke I, Hansen MD, et al. Comprehensive protocols for culturing and molecular biological analysis of IBD patient-derived colon epithelial organoids. Front Immunol. 2023;14:1097383. doi: 10.3389/fimmu.2023.1097383
- Pott J, Kabat AM, Maloy KJ. Intestinal epithelial cell autophagy is required to protect against TNF-induced apoptosis during chronic colitis in mice. Cell Host Microbe. 2018;23(2):191-202.e194. doi: 10.1016/j.chom.2017.12.017.
- Liu H, Su J. Organoid and organoid extracellular vesicles for osteoporotic fractures therapy: current status and future perspectives. Interdiscip Med. 2023;1(3):e20230011. doi: 10.1002/inmd.20230011
- Wang H. Modeling neurological diseases with human brain organoids. Front Synaptic Neurosci. 2018;10:15. doi: 10.3389/fnsyn.2018.00015
- Szvicsek Z, Oszvald Á, Szabó L, et al. Extracellular vesicle release from intestinal organoids is modulated by Apc mutation and other colorectal cancer progression factors. Cell Mol Life Sci. 2019;76(12):2463-2476. doi: 10.1007/s00018-019-03052-1
- Gabrielli M, Tozzi F, Verderio C, Origlia N. Emerging roles of extracellular vesicles in Alzheimer’s disease: focus on synaptic dysfunction and vesicle-neuron interaction. Cells. 2022;12(1):63. doi: 10.3390/cells12010063
- Bakinowska E, Kiełbowski K, Pawlik A. The role of extracellular vesicles in the pathogenesis and treatment of rheumatoid arthritis and osteoarthritis. Cells. 2023;12(23):2716. doi: 10.3390/cells12232716
- Arthur P, Kandoi S, Lee S, et al. Biophysical, molecular and proteomic profiling of human retinal organoid-derived exosomes. Pharm Res. 2022;40(4):801-816. doi: 10.1007/s11095-022-03350-7
- Szvicsek Z, Oszvald Á, Szabó L, et al. Extracellular vesicle release from intestinal organoids is modulated by Apc mutation and other colorectal cancer progression factors. Cell Mol Life Sci. 2019;76(12):2463-2476. doi: 10.1007/s00018-019-03052-1
- Arthur P, Kandoi S, Lee S, et al. Biophysical, molecular and proteomic profiling of human retinal organoid-derived exosomes. Pharm Res. 2022;40(4):801-816. doi: 10.1007/s11095-022-03350-7
- Buenafe AC, Dorrell C, Reddy AP, Klimek J, Marks DL. Proteomic analysis distinguishes extracellular vesicles produced by cancerous versus healthy pancreatic organoids. Sci Rep. 2022;12(1):3556. doi: 10.1038/s41598-022-07451-6
- Liu Y, Li N, Zhu Y. Pancreatic organoids: A frontier method for investigating pancreatic-related diseases. Int J Mol Sci. 2023;24(4):4027. doi: 10.3390/ijms24044027
- Prasad M, Kumar R, Buragohain L, Kumari A, Ghosh M. Organoid technology: a reliable developmental biology tool for organ-specific nanotoxicity evaluation. Front Cell Dev Biol. 2021;9:696668. doi: 10.3389/fcell.2021.696668
- Liu H, Sun J, Wang M, Wang S, Su J, Xu C. Intestinal organoids and organoids extracellular vesicles for inflammatory bowel disease treatment. Chem Eng J. 2023;465:142842. doi: 10.1016/j.cej.2023.142842
- Lucafò M, Muzzo A, Marcuzzi M, Giorio L, Decorti G, Stocco G. Patient-derived organoids for therapy personalization in inflammatory bowel diseases. World J Gastroenterol. 2022;28(24):2636-2653. doi: 10.3748/wjg.v28.i24.2636
- Velasco V, Shariati SA, Esfandyarpour R. Microtechnology-based methods for organoid models. Microsyst Nanoeng. 2020;6(1):76. doi: 10.1038/s41378-020-00185-3
- Teixeira MC, Lameirinhas NS, Carvalho JPF, Silvestre AJD, Vilela C, Freire CSR. A guide to polysaccharide-based hydrogel bioinks for 3D bioprinting applications. Int J Mol Sci. 2022;23(12):6564. doi: 10.3390/ijms23126564
- Hospodiuk M, Dey M, Sosnoski D, Ozbolat IT. The bioink: a comprehensive review on bioprintable materials. Biotechnol Adv. 2017;35(2):217-239. doi: 10.1016/j.biotechadv.2016.12.006
- 30 Sun B, Han Y, Jiang W, Dai K. 3D printing bioink preparation and application in cartilage tissue reconstruction in vitro. J Shanghai Jiaotong Univ (Sci). 2021;26(3):267-271. doi: 10.1007/s12204-021-2292-6
- Liu W, Heinrich MA, Zhou Y, et al. Extrusion bioprinting of shear-thinning gelatin methacryloyl bioinks. Adv Healthc Mater. 2017;6(12):1601451. doi: 10.1002/adhm.201601451
- Wan H, Xiang J, Mao G, Pan S, Li B, Lu Y. Recent advances in the application of 3d-printing bioinks based on decellularized extracellular matrix in tissue engineering. ACS Omega. 2024;9(23):24219-24235. doi: 10.1021/acsomega.4c02847
- Takahashi K, Tanabe K, Ohnuki M, et al. Induction of pluripotent stem cells from adult human fibroblasts by defined factors. Cell. 2007;131(5):861-872. doi: 10.1016/j.cell.2007.11.019
- Benwood C, Walters-Shumka J, Scheck K, Willerth SM. 3D bioprinting patient-derived induced pluripotent stem cell models of Alzheimer’s disease using a smart bioink. Bioelectron Med. 2023;9(1):10. doi: 10.1186/s42234-023-00112-7
- Liu H, Gong Y, Zhang K, et al. Recent advances in decellularized matrix-derived materials for bioink and 3d bioprinting. Gels. 2023;9(3):195. doi: 10.3390/gels9030195
- Song SS, Park HJ, Kim YK, Kang SW. Revolutionizing biomedical research: the imperative need for heart–kidney-connected organoids. Apl Bioeng. 2024;8(1):010902. doi: 10.1063/5.0190840
- Xu C, Chai W, Huang Y, Markwald RR. Scaffold‐free inkjet printing of three‐dimensional zigzag cellular tubes. Biotechnol Bioeng. 2012;109(12):3152-3160. doi: 10.1002/bit.24591
- Serex L, Sharma K, Rizov V, Bertsch A, McKinney JD, Renaud P. Microfluidic-assisted bioprinting of tissues and organoids at high cell concentrations. Biofabrication. 2021;13(2):025006. doi: 10.1088/1758-5090/abca80
- Muenks D, Kyosev Y. Productivity comparison between vat polymerization and fused filament fabrication methods for additive manufacturing of polymers. 3D Print Addit Manuf. 2021;10(1):40-49. doi: 10.1089/3dp.2021.0009
- Cendrero AM, Fortunato GM, Munoz-Guijosa JM, De Maria C, Díaz Lantada A. Benefits of non-planar printing strategies towards eco-efficient 3D printing. Sustainability. 2021;13(4):1599. doi: 10.3390/su13041599
- Wan X, Luo L, Liu Y, Leng J. Direct ink writing based 4D printing of materials and their applications. Adv Sci. 2020;7(16):2001000. doi: 10.1002/advs.202001000
- Cui H, Nowicki M, Fisher JP, Zhang LG. 3D bioprinting for organ regeneration. Adv Healthc Mater. 2017;6(1):1601118. doi: 10.1002/adhm.201601118
- Hansen CJ, Saksena R, Kolesky DB, et al. High-throughput printing via microvascular multinozzle arrays. Adv Mater. 2013;25(1):96-102. doi: 10.1002/adma.201203321
- Ravanbakhsh H, Karamzadeh V, Bao G, Mongeau L, Juncker D, Zhang YS. Emerging technologies in multi-material bioprinting. Adv Mater. 2021;33(49):2104730. doi: 10.1002/adma.202104730
- Singh M, Haverinen HM, Dhagat P, Jabbour GE. Inkjet printing—process and its applications. Adv Mater. 2010;22(6):673-685. doi: 10.1002/adma.200901141
- Cui X, Boland T. Human microvasculature fabrication using thermal inkjet printing technology. Biomaterials. 2009;30(31):6221-6227. doi: 10.1016/j.biomaterials.2009.07.056
- Park JA, Yoon S, Kwon J, et al. Freeform micropatterning of living cells into cell culture medium using direct inkjet printing. Sci Rep. 2017;7(1):14610. doi: 10.1038/s41598-017-14726-w
- Matsusaki M, Sakaue K, Kadowaki K, Akashi M. Three‐dimensional human tissue chips fabricated by rapid and automatic inkjet cell printing. Adv Healthc Mater. 2013;2(4):534-539. doi: 10.1002/adhm.201200299
- Jamieson C, Keenan P, Kirkwood D, et al. A review of recent advances in 3D bioprinting with an eye on future regenerative therapies in veterinary medicine. Front Vet Sci. 2020;7:584193. doi: 10.3389/fvets.2020.584193
- Li J, Chen M, Fan X, Zhou H. Recent advances in bioprinting techniques: approaches, applications and future prospects. J Transl Med. 2016;14(1):271. doi: 10.1186/s12967-016-1028-0
- Carberry BJ, Hergert JE, Yavitt FM, et al. 3D printing of sacrificial thioester elastomers using digital light processing for templating 3D organoid structures in soft biomatrices. Biofabrication. 2021;13(4):044104. doi: 10.1088/1758-5090/ac1c98
- Zhang Y, Li G, Wang J, Zhou F, Ren X, Su J. Small joint organoids 3D bioprinting: construction strategy and application. Small. 2023;20(8):2302506. doi: 10.1002/smll.202302506
- Kačarević ŽP, Rider PM, Alkildani S, et al. An introduction to 3D bioprinting: possibilities, challenges and future aspects. Materials (Basel). 2018;11(11):2199. doi: 10.3390/ma11112199
- Chang MY, Bogacheva MS, Lou YR. Challenges for the applications of human pluripotent stem cell-derived liver organoids. Front Cell and Dev Biol. 2021; 9:748576. doi: 10.3389/fcell.2021.748576
- Hospodiuk M, Dey M, Sosnoski DM, Özbolat İT. The bioink: a comprehensive review on bioprintable materials. Biotechnol Adv. 2017;35(2):217-239. doi: 10.1016/j.biotechadv.2016.12.006
- Li J, Chen M, Fan X, Zhou H. Recent advances in bioprinting techniques: approaches, applications and future prospects. J Transl Med. 2016;14:1-15. doi: 10.1186/s12967-016-1028-0
- Kryou C, Leva V, Chatzipetrou M, Zergioti I. Bioprinting for liver transplantation. Bioengineering. 2019;6(4):95. doi: 10.3390/bioengineering6040095
- Bertassoni LE, Cardoso JC, Manoharan V, et al. Direct-write bioprinting of cell-laden methacrylated gelatin hydrogels. Biofabrication. 2014;6(2):024105. doi: 10.1088/1758-5082/6/2/024105
- Paxton N, Smolan W, Böck T, Melchels FPW, Groll J. Proposal to assess printability of bioinks for extrusion-based bioprinting and evaluation of rheological properties governing bioprintability. Biofabrication. 2017; 9:044107. doi: 10.1088/1758-5090/aa8dd8
- Liu W, Heinrich MA, Zhou Y, et al. Extrusion bioprinting of shear‐thinning gelatin methacryloyl bioinks. Adv Healthc Mater. 2017;6(12):1601451. doi: 10.1002/adhm.201601451
- Bernal PN, Bouwmeester M, Madrid-Wolff J, et al. Volumetric bioprinting of organoids and optically tuned hydrogels to build liver-like metabolic biofactories. Adv Mater. 2022;34(15):2110054. doi: 10.1002/adma.202110054
- Grix T, Ruppelt A, Thomas A, et al. Bioprinting perfusion-enabled liver equivalents for advanced organ-on-a-chip applications. Genes. 2018;9(4):176. doi: 10.3390/genes9040176
- Pamarthy S, Sabaawy HE. Patient derived organoids in prostate cancer: improving therapeutic efficacy in precision medicine. Mol Cancer. 2021;20(1):125. doi: 10.1186/s12943-021-01426-3
- Vlachogiannis G, Hedayat S, Vatsiou A, et al. Patient-derived organoids model treatment response of metastatic gastrointestinal cancers. Science. 2018;359(6378):920-926. doi: 10.1126/science.aao2774
- Sachs N, Ligt JD, Kopper O, et al. A living biobank of breast cancer organoids captures disease heterogeneity. Cell. 2018;172(1–2):373-386.e310. doi: 10.1016/j.cell.2017.11.010
- Maenhoudt N, Defraye C, Boretto M, et al. Developing organoids from ovarian cancer as experimental and preclinical models. Stem Cell Rep. 2020;14(4):717-729. doi: 10.1016/j.stemcr.2020.03.004
- Aboulkheyr Es H, Montazeri L, Aref AR, Vosough M, Baharvand H. Personalized cancer medicine: an organoid approach. Trends Biotechnol. 2018;36(4):358-371. doi: 10.1016/j.tibtech.2017.12.005
- Schene IF, Joore IP, Oka R, et al. Prime editing for functional repair in patient-derived disease models. Nat Commun. 2020;11(1):5352. doi: 10.1038/s41467-020-19136-7
- Gopal S, Rodrigues AL, Dordick JS. Exploiting CRISPR Cas9 in three-dimensional stem cell cultures to model disease. Front Bioeng Biotechnol. 2020;8:692. doi: 10.3389/fbioe.2020.00692
- Dekkers JF, Wiegerinck CL, de Jonge HR, et al. A functional CFTR assay using primary cystic fibrosis intestinal organoids. Nat Med. 2013;19(7):939-945. doi: 10.1038/nm.3201
- Inak G, Rybak-Wolf A, Lisowski P, et al. Defective metabolic programming impairs early neuronal morphogenesis in neural cultures and an organoid model of Leigh syndrome. Nat Commun. 2021;12(1):1929. doi: 10.1038/s41467-021-22117-z
- Zhang W, Ma L, Yang M, et al. Cerebral organoid and mouse models reveal a RAB39b-PI3K-mTOR pathway-dependent dysregulation of cortical development leading to macrocephaly/autism phenotypes. Genes Dev. 2020; 34(7–8):580-597. doi: 10.1101/gad.332494.119
- An HL, Kuo HC, Tang TK. Modeling human primary microcephaly with hiPSC-derived brain organoids carrying CPAP-E1235V disease-associated mutant protein. Front Cell Dev Biol. 2022;10:830432. doi: 10.3389/fcell.2022.830432
- Xiaoshuai L, Qiushi W, Rui W. Advantages of CRISPR-Cas9 combined organoid model in the study of congenital nervous system malformations. Front Bioeng Biotechnol. 2022;10:932936. doi: 10.3389/fbioe.2022.932936
- Geurts MH, de Poel E, Pleguezuelos-Manzano C, et al. Evaluating CRISPR-based prime editing for cancer modeling and CFTR repair in organoids. Life Sci Alliance. 2021;4(10). doi: 10.26508/lsa.202000940
- Geurts MH, de Poel E, Amatngalim GD, et al. CRISPR-based adenine editors correct nonsense mutations in a cystic fibrosis organoid biobank. Cell Stem Cell. 2020;26(4): 503-510.e507. doi: 10.1016/j.stem.2020.01.019
- Al-Mansour AHM, Rizk ANGA, Al-Mansour HMS, et al. Updates in the use of 3D bioprinting in biomedical engineering for clinical application: a review. J Pharm Res Int. 2022;34:42-53. doi: 10.9734/jpri/2022/v34i587263
- Miller JS, Stevens KR, Yang MT, et al. Rapid casting of patterned vascular networks for perfusable engineered three-dimensional tissues. Nat Mater. 2012;11(9):768-774. doi: 10.1038/nmat3357
- Daly AC, Davidson MD, Burdick JA. 3D bioprinting of high cell-density heterogeneous tissue models through spheroid fusion within self-healing hydrogels. Nat Commun. 2021;12(1):753. doi: 10.1038/s41467-021-21029-2
- Ayan B, Heo DN, Zhang Z, et al. Aspiration-assisted bioprinting for precise positioning of biologics. Sci Adv. 2020;6(10):eaaw5111. doi: 10.1126/sciadv.aaw5111
- Kang HW, Lee SJ, Ko IK, Kengla C, Yoo JJ, Atala A. A 3D bioprinting system to produce human-scale tissue constructs with structural integrity. Nat Biotechnol. 2016;34(3): 312-319. doi: 10.1038/nbt.3413
- Zhang YS, Yue K, Aleman J, et al. 3D bioprinting for tissue and organ fabrication. Ann Biomed Eng. 2017;45(1):148-163. doi: 10.1007/s10439-016-1612-8
- Brassard JA, Nikolaev M, Hübscher T, Hofer M, Lutolf MP. Recapitulating macro-scale tissue self-organization through organoid bioprinting. Nat Mater. 2021;20(1):22-29. doi: 10.1038/s41563-020-00803-5
- Kolesky DB, Truby RL, Gladman AS, Busbee TA, Homan KA, Lewis JA. 3D bioprinting of vascularized, heterogeneous cell-laden tissue constructs. Adv Mater. 2014;26(19):3124-3130. doi: 10.1002/adma.201305506
- Freeman FE, Burdis R, Kelly DJ. Printing new bones: from print-and-implant devices to bioprinted bone organ precursors. Trends Mol Med. 2021;27(7):700-711. doi: 10.1016/j.molmed.2021.05.001
- Qu J, Kalyani FS, Liu L, Cheng T, Chen L. Tumor organoids: synergistic applications, current challenges, and future prospects in cancer therapy. Cancer Commun. 2021;41(12):1331-1353. doi: 10.1002/cac2.12224
- Li YE, Jodat YA, Samanipour R, et al. Toward a neurospheroid niche model: optimizing embedded 3D bioprinting for fabrication of neurospheroid brain-like co-culture constructs. Biofabrication. 2020;13(1):015014. doi: 10.1088/1758-5090/abc1be
- Flores-Torres S, Peza-Chavez O, Kuasne H, et al. Alginate-gelatin-matrigel hydrogels enable the development and multigenerational passaging of patient-derived 3D bioprinted cancer spheroid models. Biofabrication. 2021;13(2):025001. doi: 10.1088/1758-5090/abdb87
- Chen H, Wu Z, Gong Z, et al. Acoustic bioprinting of patient-derived organoids for predicting cancer therapy responses. Adv Healthc Mater. 2022;11(13):e2102784. doi: 10.1002/adhm.202102784
- Soman SS, Vijayavenkataraman S. Applications of 3D bioprinted-induced pluripotent stem cells in healthcare. Int J Bioprint. 2020;6(4):280. doi: 10.18063/ijb.v6i4.280
- Almutary AG, Alnuqaydan AM, Almatroodi SA, Bakshi HA, Chellappan DK, Tambuwala MM. Development of 3D-bioprinted colitis-mimicking model to assess epithelial barrier function using albumin nano-encapsulated anti-inflammatory drugs. Biomimetics (Basel). 2023;8(1):41. doi: 10.3390/biomimetics8010041
- Lin J, Sun AR, Li J, et al. A three-dimensional co-culture model for rheumatoid arthritis pannus tissue. Front Bioeng Biotechnol. 2021;9:764212. doi: 10.3389/fbioe.2021.764212
- Madden LR, Nguyen TV, Garcia-Mojica S, et al. Bioprinted 3D primary human intestinal tissues model aspects of native physiology and ADME/tox functions. iScience. 2018;2:156-167. doi: 10.1016/j.isci.2018.03.015
- Zhu B, Wang D, Pan H, et al. Three-in-one customized bioink for islet organoid: GelMA/ECM/PRP orchestrate pro-angiogenic and immunoregulatory function. Colloids Surf B: Biointerfaces. 2023;221:113017. doi: 10.1016/j.colsurfb.2022.113017
- Wang D, Guo Y, Zhu J, et al. Hyaluronic acid methacrylate/ pancreatic extracellular matrix as a potential 3D printing bioink for constructing islet organoids. Acta Biomater. 2023;165:86-101. doi: 10.1016/j.actbio.2022.06.036
- Ao Z, Song S, Tian C, et al. Understanding immune-driven brain aging by human brain organoid microphysiological analysis platform. Adv Sci. 2022;9(27):2200475. doi: 10.1002/advs.202200475
- Ao Z, Cai H, Wu Z, et al. Tubular human brain organoids to model microglia-mediated neuroinflammation. Lab Chip. 2021;21(14):2751-2762. doi: 10.1039/d1lc00030f
- Jian H, Li X, Dong Q, Tian S, Bai S. In vitro construction of liver organoids with biomimetic lobule structure by a multicellular 3D bioprinting strategy. Cell Prolif. 2023;56(5):e13465. doi: 10.1111/cpr.13465
- Jelinsky SA, Derksen M, Bauman EB, et al. Molecular and functional characterization of human intestinal organoids and monolayers for modeling epithelial barrier. Inflamm Bowel Dis. 2022;29(2):195-206. doi: 10.1093/ibd/izac212
- Qu M, Xiong L, Lyu Y, et al. Establishment of intestinal organoid cultures modeling injury-associated epithelial regeneration. Cell Res. 2021;31(3):259-271. doi: 10.1038/s41422-020-00453-x
- d’Aldebert E, Quaranta M, Sébert M, et al. Characterization of human colon organoids from inflammatory bowel disease patients. Front Cell Dev Biol. 2020;8:363. doi: 10.3389/fcell.2020.00363
- Meir M, Salm J, Fey C, et al. Enteroids generated from patients with severe inflammation in Crohn’s disease maintain alterations of junctional proteins. J Crohn’s Colitis. 2020;14(10):1473-1487. doi: 10.1093/ecco-jcc/jjaa085
- Niklinska-Schirtz BJ, Venkateswaran S, Anbazhagan M, et al. Ileal derived organoids from Crohn’s disease patients show unique transcriptomic and secretomic signatures. Cell Mol Gastroenterol Hepatol. 2021;12(4):1267-1280. doi: 10.1016/j.jcmgh.2021.06.018
- Sun X, Cui Z, Liang Y, et al. One-stop assembly of adherent 3D retinal organoids from hiPSCs based on 3D-printed derived PDMS microwell platform. Biofabrication. 2023;15(3):035005. doi: 10.1088/1758-5090/acc761
- Fuller S, Steele M, Münch G. Activated astroglia during chronic inflammation in Alzheimer’s disease--do they neglect their neurosupportive roles? Mutat Res. 2010; 690(1–2):40-49. doi: 10.1016/j.mrfmmm.2009.08.016
- Bélarbi K, Cuvelier E, Bonte MA, et al. Glycosphingolipids and neuroinflammation in Parkinson’s disease. Mol Neurodegener. 2020;15(1):59. doi: 10.1186/s13024-020-00408-1
- Chiurchiù V, Maccarrone M. Chronic inflammatory disorders and their redox control: from molecular mechanisms to therapeutic opportunities. Antioxid Redox Signal. 2011;15(9):2605-2641. doi: 10.1089/ars.2010.3547
- Bélanger M, Magistretti PJ. The role of astroglia in neuroprotection. Dialogues Clin Neurosci. 2009;11(3): 281-295. doi: 10.31887/dcns.2009.11.3/mbelanger
- Bordoni M, Rey F, Fantini V, et al. From neuronal differentiation of iPSCs to 3D neuro-organoids: modelling and therapy of neurodegenerative diseases. Int J Mol Sci. 2018;19(12):3972. doi: 10.3390/ijms19123972
- Ormel PR, Sá RVD, Bodegraven EJV, et al. Microglia innately develop within cerebral organoids. Nat Commun. 2018;9(1):4167. doi: 10.1038/s41467-018-06684-2
- Bi FC, Yang XH, Cheng X, et al. Optimization of cerebral organoids: a more qualified model for Alzheimer’s disease research. Transl Neurodegener. 2021;10(1):27. doi: 10.1186/s40035-021-00252-3
- Kim H, Park HJ, Choi H, et al. Modeling G2019S-LRRK2 sporadic Parkinson’s disease in 3D midbrain organoids. Stem Cell Rep. 2019;12(3):518-531. doi: 10.1016/j.stemcr.2019.01.020
- Lozano R, Stevens L, Thompson BC, et al. 3D printing of layered brain-like structures using peptide modified gellan gum substrates. Biomaterials. 2015;67: 264-273. doi: 10.1016/j.biomaterials.2015.07.022
- Kuzmenko V, Karabulut E, Pernevik E, Enoksson P, Gatenholm P. Tailor-made conductive inks from cellulose nanofibrils for 3D printing of neural guidelines. Carbohydr Polym. 2018;189:22-30. doi: 10.1016/j.carbpol.2018.01.097
- Zhou Z, Cong L, Cong X. Patient-derived organoids in precision medicine: drug screening, organoid-on-a-chip and living organoid biobank. Front Oncol. 2021;11:762184. doi: 10.3389/fonc.2021.762184
- Ayan B, Heo DN, Zhang Z, et al. Aspiration-assisted bioprinting for precise positioning of biologics. Sci Adv. 2020;6(10):eaaw5111. doi: 10.1126/sciadv.aaw5111
- Lehmann R, Lee CM, Shugart EC, et al. Human organoids: a new dimension in cell biology. Mol Biol Cell. 2019;30(10):1129-1137. doi: 10.1091/mbc.E19-03-0135
- Cidonio G, Glinka M, Dawson JI, Oreffo ROC. The cell in the ink: improving biofabrication by printing stem cells for skeletal regenerative medicine. Biomaterials. 2019;209: 10-24. doi: 10.1016/j.biomaterials.2019.04.009
- Dey M, Özbolat İT. 3D bioprinting of cells, tissues and organs. Sci Rep. 2020;10(1):14023. doi: 10.1038/s41598-020-70086-y
- Rajasekar S, Lin D, Abdul L, et al. IFlowPlate – a customized 384‐well plate for the culture of perfusable vascularized colon organoids. Adv Mater. 2020;32(46):e2002974. doi: 10.1002/adma.202002974
- Cakir B, Xiang Y, Tanaka Y, et al. Engineering of human brain organoids with a functional vascular-like system. Nat Methods. 2019;16(11):1169-1175. doi: 10.1038/s41592-019-0586-5
- Zhang YS, Arneri A, Bersini S, et al. Bioprinting 3D microfibrous scaffolds for engineering endothelialized myocardium and heart-on-a-chip. Biomaterials. 2016;110:45-59. doi: 10.1016/j.biomaterials.2016.09.003
- Zhang J, Griesbach J, Ganeyev M, et al. Long-term mechanical loading is required for the formation of 3D bioprinted functional osteocyte bone organoids. Biofabrication. 2022;14(3):035018. doi: 10.1088/1758-5090/ac73b9
- Kronemberger GS, Miranda GSD, Tavares RSN, Kopke ÚDA, Baptista LS. Recapitulating tumorigenesis in vitro: opportunities and challenges of 3D bioprinting. Front Bioeng Biotechnol. 2021;9:682498. doi: 10.3389/fbioe.2021.682498
- Abdollahi S. Extracellular vesicles from organoids and 3D culture systems. Biotechnol Bioeng. 2021;118(3):1029-1049. doi: 10.1002/bit.27606
- Agarwal P, Anees A, Harsiddharay RK, Kumar P, Tripathi PK. A comprehensive review on exosome: recent progress and outlook. Pharm Nanotechnol. 2023;12(1):2-13. doi: 10.2174/2211738511666230523114311
- Zhang Y, Liu Y, Liu H, Tang WH. Exosomes: biogenesis, biologic function and clinical potential. Cell Biosci. 2019;9(1):19. doi: 10.1186/s13578-019-0282-2
- Teng F, Fussenegger M. Shedding light on extracellular vesicle biogenesis and bioengineering. Adv Sci. 2020;8(1):2003505. doi: 10.1002/advs.202003505
- Spiers JG, Vassileff N, Hill AF. Neuroinflammatory modulation of extracellular vesicle biogenesis and cargo loading. Neuromolecular Med. 2022;24(4):385-391. doi: 10.1007/s12017-022-08704-3
- Niel GV, D’Angelo G, Raposo G. Shedding light on the cell biology of extracellular vesicles. Nat Rev Mol Cell Biol. 2018;19(4):213-228. doi: 10.1038/nrm.2017.125
- Krylova SV, Feng D. The machinery of exosomes: biogenesis, release, and uptake. Int J Mol Sci. 2023;24(2):1337. doi: 10.3390/ijms24021337
- Sedgwick AE, D’Souza-Schorey C. The biology of extracellular microvesicles. Traffic. 2018;19(5):319-327. doi: 10.1111/tra.12558
- Thippabhotla S, Zhong C, He M. 3D cell culture stimulates the secretion of in vivo like extracellular vesicles. Sci Rep. 2019;9(1):13012. doi: 10.1038/s41598-019-49671-3
- Bettio V, Mazzucco E, Antona A, et al. Extracellular vesicles from human plasma for biomarkers discovery: impact of anticoagulants and isolation techniques. PLoS One. 2023;18(5):e0285440. doi: 10.1371/journal.pone.0285440
- Clevers, H. Modeling development and disease with organoids. Cell. 2016;165(7):1586-1597. doi: 10.1016/j.cell.2016.05.082
- Rocha S, Carvalho J, Oliveira P, et al. 3D cellular architecture affects MicroRNA and protein cargo of extracellular vesicles. Adv Sci (Weinh).2019;6(4):1800948. doi: 10.1002/advs.201800948
- Zhang Y, Chopp M, Zhang ZG, et al. Systemic administration of cell-free exosomes generated by human bone marrow derived mesenchymal stem cells cultured under 2D and 3D conditions improves functional recovery in rats after traumatic brain injury. Neurochem Int. 2017;111:69-81. doi: 10.1016/j.neuint.2016.08.003
- Zhang Y, Yan Y, Meng J, Girotra M, Ramakrishnan S, Roy S. Immune modulation mediated by extracellular vesicles of intestinal organoids is disrupted by opioids. Mucosal Immunol. 2021;14(4):887-898. doi: 10.1038/s41385-021-00392-9
- Cao J, Wang B, Tang T, et al. Three-dimensional culture of MSCs produces exosomes with improved yield and enhanced therapeutic efficacy for cisplatin-induced acute kidney injury. Stem Cell Res Ther. 2020;11(1):206. doi: 10.1186/s13287-020-01719-2
- Yan L, Wu X. Exosomes produced from 3D cultures of umbilical cord mesenchymal stem cells in a hollow-fiber bioreactor show improved osteochondral regeneration activity. Cell Biol Toxicol. 2020;36(2):165-178. doi: 10.1007/s10565-019-09504-5
- Ji X, Zhou S, Wang N, et al. Cerebral-organoid-derived exosomes alleviate oxidative stress and promote LMX1A-dependent dopaminergic differentiation. Int J Mol Sci. 2023;24(13):11048. doi: 10.3390/ijms241311048
- Zhou J, Flores-Bellver M, Pan J, et al. Human retinal organoids release extracellular vesicles that regulate gene expression in target human retinal progenitor cells. Sci Rep. 2021;11(1):21128. doi: 10.1038/s41598-021-00542-w
- Zhou J, Flores-Bellver M, Pan J, et al. Human retinal organoids release extracellular vesicles that regulate gene expression in target human retinal progenitor cells. Sci Rep. 2021;11(1):21128. doi: 10.1038/s41598-021-00542-w
- 144. Manai F, Smedowski A, Kaarniranta K, Comincini S, Amadio M. Extracellular vesicles in degenerative retinal diseases: a new therapeutic paradigm. J Control Release. 2024;365:448-468. doi: 10.1016/j.jconrel.2023.11.035
- Rocha S, Carvalho J, Oliveira P, et al. 3D cellular architecture affects MicroRNA and protein cargo of extracellular vesicles. Adv Sci. 2018;6(4):1800948. doi: 10.1002/advs.201800948
- Abdollahi S. Extracellular vesicles from organoids and 3D culture systems. Biotechnol Bioeng. 2020;118(3):1029-1049. doi: 10.1002/bit.27606
- Li H, Gao L, Du J, Ma T, Ye Z, Li Z. To better generate organoids, what can we learn from teratomas? Front Cell Dev Biol. 2021;9:700482. doi: 10.3389/fcell.2021.700482
- Maiullari F, Chirivì M, Costantini M, et al. In vivo organized neovascularization induced by 3D bioprinted endothelial-derived extracellular vesicles. Biofabrication. 2021;13(3):035014. doi: 10.1088/1758-5090/abdacf
- Zhang Y, Huo M, Wang Y, et al. A tailored bioactive 3D porous poly(lactic-acid)-exosome scaffold with osteo-immunomodulatory and osteogenic differentiation properties. J Biol Eng. 2022;16(1):22. doi: 10.1186/s13036-022-00301-z
- Kang Y, Xu J, Meng L, et al. 3D bioprinting of dECM/Gel/ QCS/nHAp hybrid scaffolds laden with mesenchymal stem cell-derived exosomes to improve angiogenesis and osteogenesis. Biofabrication. 2023;15(2):024103. doi: 10.1088/1758-5090/acb6b8
- Sun Y, Zhang B, Zhai D, Wu C. Three-dimensional printing of bioceramic-induced macrophage exosomes: immunomodulation and osteogenesis/angiogenesis. NPG Asia Mater. 2021;13(1):72. doi: 10.1038/s41427-021-00340-w
- Yerneni SS, Lathwal S, Shrestha P, et al. Rapid on-demand extracellular vesicle augmentation with versatile oligonucleotide tethers. ACS Nano. 2019;13(9): 10555-10565. doi: 10.1021/acsnano.9b04651
- Chen P, Zheng L, Wang Y, et al. Desktop-stereolithography 3D printing of a radially oriented extracellular matrix/mesenchymal stem cell exosome bioink for osteochondral defect regeneration. Theranostics. 2019;9(9): 2439-2459. doi: 10.7150/thno.31017
- Yerneni SS, Whiteside TL, Weiss LE, Campbell PG. Bioprinting exosome-like extracellular vesicle microenvironments. Bioprinting. 2019;13:e00041. doi: 10.1016/j.bprint.2019.e00041
- Theodoraki MN, Yerneni S, Gooding WE, et al. Circulating exosomes measure responses to therapy in head and neck cancer patients treated with cetuximab, ipilimumab, and IMRT. OncoImmunology. 2019;8(7):e1593805. doi: 10.1080/2162402X.2019.1593805
- Buenafe AC, Dorrell C, Reddy AP, Klimek J, Marks DL. Proteomic analysis distinguishes extracellular vesicles produced by cancerous versus healthy pancreatic organoids. Sci Rep. 2022;12(1):3556. doi: 10.1038/s41598-022-07451-6
- Huang L, Bockorny B, Paul I, et al. Pancreatic tumor organoids for modeling in vivo drug response and discovering clinically-actionable biomarkers. bioRxiv. 2019:513267. doi: 10.1101/513267
- Risha Y, Minic Z, Ghobadloo SM, Berezovski MV. The proteomic analysis of breast cell line exosomes reveals disease patterns and potential biomarkers. Sci Rep. 2020;10(1):13572. doi: 10.1038/s41598-020-70393-4
- Hyung S, Ko J, Heo YJ, et al. Patient-derived exosomes facilitate therapeutic targeting of oncogenic MET in advanced gastric cancer. Sci Adv. 2023;9(47):eadk1098. doi: 10.1126/sciadv.adk1098
- Han P, Ivanovski S. 3D bioprinted extracellular vesicles for tissue engineering-a perspective. Biofabrication. 2022;15(1):013001. doi: 10.1088/1758-5090/ac9809
- Bartnikowski M, Vaquette C, Ivanovski S. Workflow for highly porous resorbable custom 3D printed scaffolds using medical grade polymer for large volume alveolar bone regeneration. Clin Oral Implants Res. 2020;31(5):431-441. doi: 10.1111/clr.13579
- Liu W, Heinrich MA, Zhou Y, et al. Extrusion bioprinting of shear‐thinning gelatin methacryloyl bioinks. Adv Healthc Mater. 2017;6(12):1601451. doi: 10.1002/adhm.201601451