Biomimetic structural design and performance study of 3D-printed graded minimal surface bone scaffolds with enhanced bioactivity
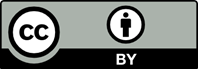
Bone bionics and structural engineering have played a vital role in bone regeneration, with artificial scaffolds generating widespread interest. However, the mechanical properties and bone regeneration potential of biomimetic structures remain unclear. Herein, biodegradable polymer composites based on poly(butylene adipate-co-terephthalate)/poly(lactic acid) (PBAT/PLA) were 3D-printed into lattice structures as tissue engineering scaffolds. For structural design, graded diamond (D) minimal surfaces were proposed and designed to mimic the natural bone structure. The graded topologies were realized by designing gradient thickness either radially from center to edge or vertically from top to bottom. The mechanical performance of these graded samples displayed better load-carrying and energy absorption capacity than the uniform counterparts. No obvious damage was detected in the internal microstructure of the compressed samples using computed tomography. Subsequently, platelet-rich plasma (PRP), containing diverse cytokines, was loaded on the graded scaffolds. The PRP-loaded D-scaffold reported improved in vitro cell proliferation and osteoblast differentiation. Finally, femoral condyle defect repair results indicated that the PRP-loaded D-scaffold effectively promoted early-stage bone regeneration. Overall, this work provides insights into fabricating artificial scaffolds with bioactive factors and biomimetic lattice structures.
- Zhang YT, Aiyiti W, Du S, Jia R, Jiang HF. Design and mechanical behaviors of a novel tantalum lattice structure fabricated by SLM. Virtual Phys Prototy. 2023;18(1):2192702. doi: 10.1080/17452759.2023.2192702
- Chang CM, Wong PC, Ou SL, Ko CE, Wang YT. Optimizing implant lattice design for large distal femur defects: stimulating interface bone growth to enhance osseointegration. Int J Bioprint. 2024;10:544-562. doi: 10.36922/ijb.2590
- Cuan-Urquizo E, Silva RG. Fused filament fabrication of cellular, lattice and porous mechanical metamaterials: a review. Virtual Phys Prototy. 2023;18:2224300. doi: 10.1080/17452759.2023.2224300
- Maskery I, Ashcroft IA. The deformation and elastic anisotropy of a new gyroid-based honeycomb made by laser sintering. Addi Manuf. 2020;36:101548. doi: 10.1016/j.addma.2020.101548
- Xi HF, Zhou ZC, Zhang HH, Huang SQ, Xiao H. Multi-morphology TPMS structures with multi-stage yield stress platform and multi-level energy absorption: design, manufacturing, and mechanical properties. Eng Struct. 2023;294:116733. doi: 10.1016/j.engstruct.2023.116733
- Yin HF, Tan DW, Wen GL, Tian WY, Wu QK. Crashworthiness analysis and optimization design of TPMS-filled structure. Int J Crashworthiness. 2022;27:1481-1498. doi: 10.1080/13588265.2021.1959171
- Wang HW, Chen CH, Chen KH, Zeng YH, Lin CL. Designing a 3D-printed medical implant with mechanically macrostructural topology and microbionic lattices: a novel wedge-shaped spacer for high tibial osteotomy and biomechanical study. Int J Bioprint. 2024;10:1584. doi: 10.36922/ijb.1584
- Wang XB, Zhang L, Song B, et al. Anisotropic mechanical and mass-transport performance of Ti6Al4V plate-lattice scaffolds prepared by laser powder bed fusion. Acta Biomater. 2022;148:374-388. doi: 10.1016/j.actbio.2022.06.016
- Fu H, Huang X, Kaewunruen S. Experimental investigations into nonlinear dynamic behaviours of triply periodical minimal surface structures. Compos Struct. 2023;323:117510. doi: 10.1016/j.compstruct.2023.117510
- Cai JX, Ma YB, Deng ZC. On the effective elastic modulus of the ribbed structure based on Schwarz Primitive triply periodic minimal surface. Thin Wall Struct. 2022;170:108642. doi: 10.1016/j.tws.2021.108642
- Oh SH, An CH, Seo B, Kim J, Park CY, Park K. Functional morphology change of TPMS structures for design and additive manufacturing of compact heat exchangers. Addi Manuf. 2023;76:103778. doi: 10.1016/j.addma.2023.103778
- Reynolds BW, Fee CJ, Morison KR, Holland DJ. Characterisation of heat transfer within 3D printed TPMS heat exchangers. Int J Heat Mass Transfer. 2023;212:124264. doi: 10.1016/j.ijheatmasstransfer.2023.124264
- Sang L, Wu WY, Sun ZQ, et al. Reusability and energy absorption behavior of 4D-printed heterogeneous lattice structures based on biomass shape memory polyester. J Mater Res Technol. 2023;27:1563-1578. doi: 10.1016/j.jmrt.2023.09.323
- Laskowska D, Szatkiewicz T, Balasz B, Mitura K. Mechanical properties and energy absorption abilities of diamond TPMS cylindrical structures fabricated by selective laser melting with 316L stainless steel. Materials. 2023;16:3196. doi: 10.3390/ma16083196
- Bo L, Jiawei F, Zhiwei L, Yong H, Jianzhong F. Controllable three-dimension auxetic structure design strategies based on triply periodic minimal surfaces and the application in hip implant. Virtual Phys Prototy. 2023;18:e2170890. doi: 10.1080/17452759.2023.2170890
- Zou SJ, Mu YR, Pan BC, et al. Mechanical and biological properties of enhanced porous scaffolds based on triply periodic minimal surfaces. Mater Des. 2022;219:110803. doi: 10.1016/j.matdes.2022.110803
- Zhang Q, Ma LM, Ji XF, et al. High-strength hydroxyapatite scaffolds with minimal surface macrostructures for load-bearing bone regeneration. Adv Funct Mater. 2022;32:2204182. doi: 10.1002/adfm.202204182
- Zhang ZH, He FT, Wang B, et al. Biodegradable PGA/PBAT blends for 3D printing: material performance and periodic minimal surface structures. Polymers. 2021;13(1):3757. doi: 10.3390/polym13213757
- Cao YX, Lai SY, Wu WY, et al. Design and mechanical evaluation of additively-manufactured graded TPMS lattices with biodegradable polymer composites. J Mater Res Technol. 2023;23:2868-2880. doi: 10.1016/j.jmrt.2023.01.221
- Ulbrich LM, Balbinot GD, Brotto GL, et al. 3D printing of poly(butylene adipate-co-terephthalate) (PBAT)/ niobium containing bioactive glasses (BAGNb) scaffolds: characterization of composites, in vitro bioactivity, and in vivo bone repair. J Tissue Eng Regen Med. 2022;16:267-278. doi: 10.1002/term.3276
- Qiu SW, Xia YH, Sun JD, Wang SS, Xing QS. Poly (butylene adipate-co-terephthalate)/Sodium alginate blends have superior characteristics and can be used to fabricate vascular stents. Mater Res Express. 2022;9:055401. doi: 10.1088/2053-1591/ac6a4c
- Zeng C, Zhang MM. 3D-printed PCL/β-TCP/CS composite artificial bone and histocompatibility study. J Orthop Surg Res. 2024;18:981. doi: 10.1186/s13018-023-04489-8
- Yeo T, Ko YG, Kim EJ, Kwon OK, Chuang HY, Kwon OH. Promoting bone regeneration by 3D-printed poly(glycolic acid)/hydroxyapatite composite scaffolds. J Ind Eng Chem. 2021;94:343-351. doi: 10.1016/j.jiec.2020.11.004
- Mushtaq RT, Wang YE, Bao CW, et al. Maximizing performance and efficiency in 3D printing of polylactic acid biomaterials: Unveiling of microstructural morphology, and implications of process parameters and modeling of the mechanical strength, surface roughness, print time, and print energy for fused filament fabricated (FFF) bioparts. Int J Biol Macromol. 2024;259:12920. doi: 10.1016/j.ijbiomac.2024.129201
- Natarajan A, Sivadas VP, Nair PD. 3D-printed biphasic scaffolds for the simultaneous regeneration of osteochondral tissues. Biomed Mater. 2021;16:054102. doi: 10.1088/1748-605X/ac14cb
- Kechagias J, Chaidas D, Vidakis N, Salonitis K, Vaxevanidis NM. Key parameters controlling surface quality and dimensional accuracy: a critical review of FFF process. Mater Manuf Process. 2022;37(9):963-984. doi: 10.1080/10426914.2022.2032144
- Bahraminasab M, Doostmohammadi N, Talebi A, et al. 3D printed polylactic acid/gelatin-nano-hydroxyapatite/ platelet-rich plasma scaffold for critical-sized skull defect regeneration. Biomed Eng Online. 2022;21:86. doi: 10.1186/s12938-022-01056-w
- Wei L, Wu SH, Kuss M, et al. 3D printing of silk fibroin-based hybrid scaffold treated with platelet rich plasma for bone tissue engineering. Bioact Mater. 2019;4:256-260. doi: 10.1016/j.bioactmat.2019.09.001
- Liu W, Sang L, Zhang ZH, Ju SL, Wang F, Zhao YP. Compression and resilient behavior of graded triply periodic minimal surface structures with soft materials fabricated by fused filament fabrication. J Manuf Process. 2023;105:1-13. doi: 10.1016/j.jmapro.2023.09.034
- Huang SF, Chang CM, Lian CY, Chan YT, Liu ZY, Lin CL. Biomechanical evaluation of an osteoporotic anatomical 3D printed posterior lumbar interbody fusion cage with internal lattice design based on weighted topology optimization. Int J Bioprinting. 2023,9:697. doi: 10.18063/ijb.697
- Liu QY, Wei F, Coathup M, Shen W, Wu DZ. Effect of porosity and pore shape on the mechanical and biological properties of additively manufactured bone scaffolds. Adv Healthc Mater. 2023;12:2301111. doi: 10.1002/adhm.202301111
- Seker S, Aral D, Elcin AE, Murat EY. Biomimetic mineralization of platelet lysate/oxidized dextran cryogel as a macroporous 3D composite scaffold for bone repair. Biomed Mater. 2024;19:025006. doi: 10.1088/1748-605X/ad1c9a
- Li ZL, Wang YX, Zhao CK, et al. Polysaccharide hybrid scaffold encapsulated endogenous factors for microfracture enhancement by sustainable release and cell recruitment. Compos Part B Eng. 2024;273:111235. doi: 10.1016/j.compositesb.2024.111235
- Xue P, Tan XX, Xi HZ, et al. Low-temperature deposition 3D printing biotin-doped PLGA/β-TCP scaffold for repair of bone defects in osteonecrosis of femoral head. Int J Bioprint. 2024;10:1152. doi: 10.36922/ijb.1152
- Gao X, Wang H, Luan S, Zhou G. Low-temperature printed hierarchically porous induced-biomineralization polyaryletherketone scaffold for bone tissue engineering. Adv Healthc Mater. 2022;11:e2200977. doi: 10.1002/adhm.202200977
- Zhu H, Lin ZH, Luan QF, et al. Angiogenesis-promoting composite TPMS bone tissue engineering scaffold for mandibular defect regeneration. Int J Bioprint. 2024;10(1);0153. doi: 10.36922/ijb.0153