Oozing: An accessible technique to create 3D-printed scaffolds suitable for tissue engineering
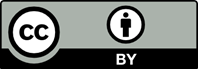
Tissue-engineered constructs require mimicking the extracellular matrix microenvironment of native tissue for better promoting cell growth. Commercial three-dimensional (3D) printers provide a versatile platform to fabricate tissue models, but they possess certain constraints regarding the reproduction of natural tissue structures due to the limited functionality of current slicing strategies and hardware. In this study, we present a new approach to 3D-printing polylactic acid (PLA) constructs with fibers in the range of microns by combining the oozing effect and algorithm-aided design (AAD) with a conventional fused deposition modeling printer. Three different oozing geometries were compared with two controls to explore their mechanical behavior and their cellular culture growth potential. Microscopic analysis revealed that oozing groups possessed higher porosity and statistically significantly thinner fibers than controls. Sodium hydroxide treatment reversibly increased the hydrophilicity of PLA without affecting the scaffolds’ mechanical properties in the compression tests. In addition, cell culture assays showed that oozing specimens exhibited a greater capacity of promoting SaOs-2 osteoblastic cell proliferation after 7 days in comparison with controls. We demonstrated that randomly distributed microfibered environments can be fabricated with an ordinary 3D printer utilizing the oozing effect and advanced AAD, resulting in improved biomimetic 3D constructs for tissue-engineering strategies.
- Langer R, Vacanti JP. Tissue engineering. Science. 1993;260(5110):920-926. doi: 10.1126/science.8493529
- Ikada Y. Challenges in tissue engineering. J R Soc Interface. 2006;3(10):589-601. doi: 10.1098/rsif.2006.0124
- Shin H, Jo S, Mikos AG. Biomimetic materials for tissue engineering. Biomaterials. 2003;24(24):4353-4364. doi: 10.1016/S0142-9612(03)00339-9
- Yue B. Biology of the extracellular matrix: an overview. J Glaucoma. 2014;23(8):S20-S23. doi: 10.1097/IJG.0000000000000108
- Ceretti E, Ginestra P, Neto PI, Fiorentino A, da Silva JVL. Multi-layered scaffolds production via fused deposition modeling (FDM) using an open source 3D printer: process parameters optimization for dimensional accuracy and design reproducibility. Procedia CIRP. 2017;65:13-18. doi: 10.1016/j.procir.2017.04.042
- Jiang X, Zheng W, Takayama S, Chapman RG, Kane RS, Whitesides GM. Micro-scale patterning of cells and their environment. In: Lanza R, Langer R, Vacanti JP, eds. Principles of Tissue Engineering. 4th ed. Cambridge, Massachusetts: Elsevier Inc.; 2013: 359-384. doi: 10.1016/B978-0-12-398358-9.00019-7
- Richards DJ, Tan Y, Jia J, Yao H, Mei Y. 3D printing for tissue engineering. ISR J Chem. 2013;53(9-10):805-814. doi: 10.1002/ijch.201300086
- Lee M, Wu BM. Recent advances in 3D printing of tissue engineering scaffolds. Methods Mol Biol. 2012;868:257-267. doi: 10.1007/978-1-61779-764-4_15
- Choi JW, Kim N. Clinical application of three-dimensional printing technology in craniofacial plastic surgery. Arch Plast Surg. 2015;42(3):267-277. doi: 10.5999/aps.2015.42.3.267
- Jakus AE, Secor EB, Rutz AL, Jordan SW, Hersam MC, Shah RN. Three-dimensional printing of high-content graphene scaffolds for electronic and biomedical applications. ACS Nano. 2015;9(4):4636-4648. doi: 10.1021/acsnano.5b01179
- Huang H, Zhang B, Zhong J, et al. The behavior between fluid and structure from coupling system of bile, bile duct, and polydioxanone biliary stent: a numerical method. Med Eng Phys. 2023;113:103966. doi: 10.1016/J.MEDENGPHY.2023.103966
- Xu Y, Zhang F, Zhai W, Cheng S, Li J, Wang Y. Unraveling of advances in 3D-printed polymer-based bone scaffolds. Polymers. 2022;14(3). doi: 10.3390/polym14030566
- Valashani SMM, Barrett CJ, Barthelat F. Self-assembly of microscopic tablets within polymeric thin films: a possible pathway towards new hybrid materials. RSC Adv. 2015;5(7):4780-4787. doi: 10.1039/c4ra15166f
- Turnbull G, Clarke J, Picard F, et al. 3D bioactive composite scaffolds for bone tissue engineering. Bioact Mater. 2018;3(3):278-314. doi: 10.1016/j.bioactmat.2017.10.001
- Szojka A, Lalh K, Andrews SHJ, Jomha NM, Osswald M, Adesida AB. Biomimetic 3D printed scaffolds for meniscus tissue engineering. Bioprinting. 2017;8:1-7. doi: 10.1016/j.bprint.2017.08.001
- Kabirian F, Ditkowski B, Zamanian A, Heying R, Mozafari M. An innovative approach towards 3D-printed scaffolds for the next generation of tissue-engineered vascular grafts. Mater Today Proc. 2018;5(7):15586-15594. doi: 10.1016/j.matpr.2018.04.167
- DeStefano V, Khan S, Tabada A. Applications of PLA in modern medicine. Eng Regen. 2020;1:76-87. doi: 10.1016/j.engreg.2020.08.002
- Mota C, Puppi D, Chiellini F, Chiellini E. Additive manufacturing techniques for the production of tissue engineering constructs. J Tissue Eng Regen Med. 2015;9(3):174-190. doi: 10.1002/term.1635
- Chung JJ, Im H, Kim SH, Park JW, Jung Y. Toward biomimetic scaffolds for tissue engineering: 3D printing techniques in regenerative medicine. Front Bioeng Biotechnol. 2020;8. doi: 10.3389/fbioe.2020.586406
- Alagoz AS, Hasirci V. 3D printing of polymeric tissue engineering scaffolds using open-source fused deposition modeling. Emergent Mater. 2020;3(4):429-439. doi: 10.1007/s42247-019-00048-2
- Gillispie G, Prim P, Copus J, et al. Assessment methodologies for extrusion-based bioink printability. Biofabrication. 2020;12(2). doi: 10.1088/1758-5090/ab6f0d
- Papaioannou TG, Manolesou D, Dimakakos E, Tsoucalas G, Vavuranakis M, Tousoulis D. 3D bioprinting methods and techniques: applications on artificial blood vessel fabrication. Acta Cardiol Sin. 2019;35(3):284-289. doi: 10.6515/ACS.201905_35(3).20181115A
- Lee JS, Hong JM, Jung JW, Shim JH, Oh JH, Cho DW. 3D printing of composite tissue with complex shape applied to ear regeneration. Biofabrication. 2014;6(2). doi: 10.1088/1758-5082/6/2/024103
- Lee A, Hudson AR, Shiwarski DJ, et al. 3D bioprinting of collagen to rebuild components of the human heart. Science. 2019;365(6452):482-487. doi: 10.1126/science.aav9051
- Jung JW, Lee JS, Cho DW. Computer-aided multiple-head 3D printing system for printing of heterogeneous organ/ tissue constructs. Sci Rep. 2016;6. doi: 10.1038/srep21685
- Liu J, Zhou Y, Lu J, et al. Injectable, tough and adhesive zwitterionic hydrogels for 3D-printed wearable strain sensors. Chem Eng J. 2023;475:146340. doi: 10.1016/J.CEJ.2023.146340
- Ramesh S, Harrysson OLA, Rao PK, et al. Extrusion bioprinting: recent progress, challenges, and future opportunities. Bioprinting. 2021;21. doi: 10.1016/j.bprint.2020.e00116
- Ng WL, Huang X, Shkolnikov V, Suntornnond R, Yeong WY. Polyvinylpyrrolidone-based bioink: influence of bioink properties on printing performance and cell proliferation during inkjet-based bioprinting. Bio-Des Manuf. 2023;6(6):676-690. doi: 10.1007/s42242-023-00245-3
- Chartrain NA, Williams CB, Whittington AR. A review on fabricating tissue scaffolds using vat photopolymerization. Acta Biomater. 2018;74:90-111. doi: 10.1016/j.actbio.2018.05.010
- Moroni L, Boland T, Burdick JA, et al. Biofabrication: a guide to technology and terminology. Trends Biotechnol. 2018;36(4):384-402. doi: 10.1016/j.tibtech.2017.10.015
- Mwema FM, Akinlabi ET. Basics of fused deposition modelling (FDM). In: Castro M, eds. SpringerBriefs in Applied Sciences and Technology. Cham, Switzerland: Springer; 2020: 1-15. doi: 10.1007/978-3-030-48259-6_1
- Hoque ME, Chuan YL, Pashby I. Extrusion based rapid prototyping technique: an advanced platform for tissue engineering scaffold fabrication. Biopolymers. 2012; 97(2):83-93. doi: 10.1002/bip.21701
- Gross BC, Erkal JL, Lockwood SY, Chen C, Spence DM. Evaluation of 3D printing and its potential impact on biotechnology and the chemical sciences. Anal Chem. 2014;86(7):3240-3253. doi: 10.1021/ac403397r
- Yazar A, Top N, Bülbül R, Şahin I. Effect of infill density and infill pattern on mechanical properties in fused deposition modeling (FDM). In: Proceedings of the Innovative Approaches in Additive Manufacturing Congress (IA4AM). Gazi University; 2021.
- Fernandez-Vicente M, Calle W, Ferrandiz S, Conejero A. Effect of infill parameters on tensile mechanical behavior in desktop 3D printing. 3D Print Addit Manuf. 2016;3(3):183-192. doi: 10.1089/3dp.2015.0036
- Zein I, Hutmacher DW, Cheng Tan K, Hin Teoh S. Fused deposition modeling of novel scaffold architectures for tissue engineering applications. Biomaterials. 2002;23(4): 1169-1185. doi: 10.1016/S0142-9612(01)00232-0
- Boparai KS, Singh R, Singh H. Development of rapid tooling using fused deposition modeling: a review. Rapid Prototyp J. 2016;22(2):281-299. doi: 10.1108/RPJ-04-2014-0048
- Günaydın K, Türkmen HS. Common FDM 3D printing defects. In: Proceedings of the International Congress on 3D Printing (Additive Manufacturing) Technologies and Digital Industry; 2018.
- Gupta S, Bissoyi A, Bit A. A review on 3D printable techniques for tissue engineering. Bionanoscience. 2018;8(3):868-883. doi: 10.1007/s12668-018-0525-4
- Yang S, Leong KF, Du ZME, Chua CK. The design of scaffolds for use in tissue engineering. Part II. Rapid prototyping techniques. Tissue Eng. 2002;8(1):1-11. doi: 10.1089/107632702753503009
- Sukindar NA, Ariffin MKA, Baharudin BTHT, Jaafar CNA, Ismail MIS. Analyzing the effect of nozzle diameter in fused deposition modeling for extruding polylactic acid using open source 3D printing. J Teknol. 2016;78(10):7-15. doi: 10.11113/jt.v78.6265
- del Barrio Cortés E, Matutano Molina C, Rodríguez-Lorenzo L, Cubo-Mateo N. Generation of controlled micrometric fibers inside printed scaffolds using standard FDM 3D printers. Polymers. 2023;15(1). doi: 10.3390/polym15010096
- Zhang Y, Harrison C. Tomo: wearable, low-cost, electrical impedance tomography for hand gesture recognition. In: Proceedings of the 28th Annual ACM Symposium on User Interface Software and Technology. Association for Computing Machinery, Inc.; 2015:167-173. doi: 10.1145/2642918.2647356
- Lu Q, Song KY, Feng Y, Xie J. Fabrication of suspended uniform polymer microfibers by FDM 3D printing. CIRP J Manuf Sci Technol. 2021;32:179-187. doi: 10.1016/j.cirpj.2020.11.005
- Paraskevoudis K, Karayannis P, Koumoulos EP. Real-time 3d printing remote defect detection (Stringing) with computer vision and artificial intelligence. Processes. 2020;8(11):1-15. doi: 10.3390/pr8111464
- Visscher DO, Bos EJ, Peeters M, et al. Cartilage tissue engineering: preventing tissue scaffold contraction using a 3D-printed polymeric cage. Tissue Eng Part C Methods. 2016;22(6):573-584. doi: 10.1089/ten.tec.2016.0073
- Zhang Z, He H, Fu W, Ji D, Ramakrishna S. Electro-hydrodynamic direct-writing technology toward patterned ultra-thin fibers: advances, materials and applications. Nano Today. 2020;35. doi: 10.1016/j.nantod.2020.100942
- Lannutti J, Reneker D, Ma T, Tomasko D, Farson D. Electrospinning for tissue engineering scaffolds. Mater Sci Eng C. 2007;27(3):504-509. doi: 10.1016/j.msec.2006.05.019
- Chlanda A, Kijeńska E, Święszkowski W. Microscopic methods for characterization of selected surface properties of biodegradable, nanofibrous tissue engineering scaffolds. In: Proceedings of the Materials Science Forum. Trans Tech Publications Ltd; 2017:213-216. doi: 10.4028/www.scientific.net/MSF.890.213
- Kara Y, Kovács NK, Nagy-György P, Boros R, Molnár K. A novel method and printhead for 3D printing combined nano-/microfiber solid structures. Addit Manuf. 2023;61. doi: 10.1016/j.addma.2022.103315
- Gleadall A. FullControl GCode designer: open-source software for unconstrained design in additive manufacturing. Addit Manuf. 2021;46. doi: 10.1016/j.addma.2021.102109
- Naghieh S, Badrossamay M, Foroozmehr E, Kharaziha M. Combination of PLA micro-fibers and PCL-gelatin nano-fibers for development of bone tissue engineering scaffolds. IJSIEC. 2017;6(1):1-4. doi: 10.4172/2090-4908.1000150
- Molde J, Steele JAM, Pastino AK, Mahat A, Murthy NS, Kohn J. A step toward engineering thick tissues: distributing microfibers within 3D printed frames. J Biomed Mater Res A. 2020;108(3):581-591. doi: 10.1002/jbm.a.36838
- Corapi D, Morettini G, Pascoletti G, Zitelli C. Characterization of a polylactic acid (PLA) produced by fused deposition modeling (FDM) technology. Procedia Struct Integr. 2019;24:289-295. doi: 10.1016/j.prostr.2020.02.026
- Nagarajan S, Radhakrishnan S, Kalkura SN, Balme S, Miele P, Bechelany M. Overview of protein-based biopolymers for biomedical application. Macromol Chem Phys. 2019;220(14). doi: 10.1002/macp.201900126
- Ebrahimi F, Ramezani Dana H. Poly lactic acid (PLA) polymers: from properties to biomedical applications. Int J Polym Mater Polym Biomater. 2022;71(15):1117-1130. doi: 10.1080/00914037.2021.1944140
- Gregor A, Filová E, Novák M, et al. Designing of PLA scaffolds for bone tissue replacement fabricated by ordinary commercial 3D printer. J Biol Eng. 2017;11(1). doi: 10.1186/s13036-017-0074-3
- Wasyłeczko M, Sikorska W, Chwojnowski A. Review of synthetic and hybrid scaffolds in cartilage tissue engineering. Membranes. 2020;10(11):1-28. doi: 10.3390/membranes10110348
- Szojka A, Lalh K, Andrews SHJ, Jomha NM, Osswald M, Adesida AB. Biomimetic 3D printed scaffolds for meniscus tissue engineering. Bioprinting. 2017;8:1-7. doi: 10.1016/j.bprint.2017.08.001
- Singhvi MS, Zinjarde SS, Gokhale DV. Polylactic acid: synthesis and biomedical applications. J Appl Microbiol. 2019;127(6):1612-1626. doi: 10.1111/jam.14290
- Lasprilla AJR, Martinez GAR, Lunelli BH, Jardini AL, Filho RM. Poly-lactic acid synthesis for application in biomedical devices - a review. Biotechnol Adv. 2012;30(1):321-328. doi: 10.1016/j.biotechadv.2011.06.019
- Alafaghani A, Qattawi A, Ablat MA. Design consideration for additive manufacturing: fused deposition modelling. Open J Appl Sci. 2017;7(6):291-318. doi: 10.4236/ojapps.2017.76024
- Zhang B, Seong B, Nguyen VD, Byun D. 3D printing of high-resolution PLA-based structures by hybrid electrohydrodynamic and fused deposition modeling techniques. J Micromech Microeng. 2016;26(2). doi: 10.1088/0960-1317/26/2/025015
- Heljak MK, Kurzydlowski KJ, Swieszkowski W. Computer aided design of architecture of degradable tissue engineering scaffolds. Comput Methods Biomech Biomed Engin. 2017;20(15):1623-1632. doi: 10.1080/10255842.2017.1399263
- Chen S, McCarthy A, John JV, Su Y, Xie J. Converting 2D nanofiber membranes to 3D hierarchical assemblies with structural and compositional gradients regulates cell behavior. Adv Mater. 2020;32(43). doi: 10.1002/adma.202003754
- Collins MN, Ren G, Young K, Pina S, Reis RL, Oliveira JM. Scaffold fabrication technologies and structure/function properties in bone tissue engineering. Adv Funct Mater. 2021;31(21). doi: 10.1002/adfm.202010609
- Choi WJ, Hwang KS, Kwon HJ, et al. Rapid development of dual porous poly(lactic acid) foam using fused deposition modeling (FDM) 3D printing for medical scaffold application. Mater Sci Eng C. 2020;110. doi: 10.1016/j.msec.2020.110693
- Söhling N, Neijhoft J, Nienhaus V, et al. 3D-printing of hierarchically designed and osteoconductive bone tissue engineering scaffolds. Materials. 2020;13(8). doi: 10.3390/MA13081836
- Sankaravel SG, Syed RB, Manivachakan V. In vitro and mechanical characterization of PLA/egg shell biocomposite scaffold manufactured using fused deposition modeling technology for tissue engineering applications. Polym Compos. 2022;43(1):1-638. doi: 10.1002/pc.26365
- da Silva K, Kumar P, Choonara YE, du Toit LC, Pillay V. Three-dimensional printing of extracellular matrix (ECM)-mimicking scaffolds: a critical review of the current ECM materials. J Biomed Mater Res A. 2020;108(12): 2324-2350. doi: 10.1002/jbm.a.36981
- Zaszczyńska A, Moczulska-Heljak M, Gradys A, Sajkiewicz P. Advances in 3D printing for tissue engineering. Materials. 2021;14(12). doi: 10.3390/ma14123149
- Tamay DG, Usal TD, Alagoz AS, Yucel D, Hasirci N, Hasirci V. 3D and 4D printing of polymers for tissue engineering applications. Front Bioeng Biotechnol. 2019;7(JUL). doi: 10.3389/fbioe.2019.00164
- Gleadall A, Visscher D, Yang J, Thomas D, Segal J. Review of additive manufactured tissue engineering scaffolds: relationship between geometry and performance. Burns Trauma. 2018;6. doi: 10.1186/s41038-018-0121-4
- Baptista R, Guedes M, Pereira MFC, Maurício A, Carrelo H, Cidade T. On the effect of design and fabrication parameters on mechanical performance of 3D printed PLA scaffolds. Bioprinting. 2020;20. doi: 10.1016/j.bprint.2020.e00096
- Schneider M, Fritzsche N, Puciul-Malinowska A, et al. Surface etching of 3D printed poly(lactic acid) with NaOH: a systematic approach. Polymers. 2020;12(8). doi: 10.3390/POLYM12081711
- Guduric V, Metz C, Siadous R, et al. Layer-by-layer bioassembly of cellularized polylactic acid porous membranes for bone tissue engineering. J Mater Sci Mater Med. 2017;28(5). doi: 10.1007/s10856-017-5887-6