Recent developments and challenges of 3D bioprinting technologies
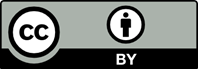
Three-dimensional (3D) bioprinting technologies play significant roles in various facets of the medical field, such as bioengineering, tissue repair, scaffolds, biomedical devices, and drug. As a versatile manufacturing technology, 3D bioprinting is able to overcome the constraints of other conventional methods and shows potential for future advancements in the field of biology. Nevertheless, the existing 3D bioprinting technologies still grapple with significant challenges in materials, equipment, and applications. Therefore, it is essential to select appropriate bioprinting method in alignment with the required application. In this review, we aim to cover the development, classification, and application of 3D bioprinting, with a particular emphasis on the fundamental printing principles. Additionally, we discuss the potential of 3D bioprinting in terms of materialization, structuralization, and functionalization, highlighting its prospective applications. We firmly believe that 3D printing technology will witness widespread adoption in the future, as it has the potential to address the limitations associated with multi-size, multi-material, multi-cell, and high-precision bioprinting.
- Lu YQ, Xu J, Su Y, et al. A biocompatible double-crosslinked gelatin/sodium alginate/dopamine/quaterniazed chitosan hydrogel for wound dressings based on 3D bioprinting technology. Int J Bioprint. 2023;9(2):439-452. doi: 10.18063/ijb.v9i1.689
- Ding YW, Zhang XW, Mi CH, Qi XY, Zhou J, Wei DX. Recent advances in hyaluronic acid-based hydrogels for 3D bioprinting in tissue engineering applications. Smart Mater Med. 2022;4:59-68. doi: 10.1016/j.smaim.2022.07.003
- Khalaf AT, Wei YY, Wan J, et al. Bone tissue engineering through 3D bioprinting of bioceramic scaffolds: a review and update. Life. 2022;12(6):903-930. doi: 10.3390/life12060903
- Wang PP, Sun LJ, Li CC, et al. Study on drug screening multicellular model for colorectal cancer constructed by three-dimensional bioprinting technology. Int J Bioprint. 2023;9(3) 379-398. doi: 10.18063/ijb.694
- Neufeld L, Yeini E, Pozzi S, et al. 3D bioprinted cancer models: from basic biology to drug development. Nat Rev Cancer. 2022;22(12):679-692. doi: 10.1038/s41568-022-00514-w
- Chae S, Cho DW. Three-dimensional bioprinting with decellularized extracellular matrix-based bioinks in translational regenerative medicine. MRS Bullet. 2022;47(1):70-79. doi: 10.1557/s43577-021-00260-8
- Muskan, Gupta D, Negi NP. 3D bioprinting: printing the future and recent advances. Bioprinting. 2022;27:e00211. doi: 10.1016/j.bprint.2022.e00211
- Antezana PE, Municoy S, Álvarez-Echazú MI, et al. The 3D bioprinted scaffolds for wound healing. Pharmaceutics, 2022;14(2):464-509. doi: 10.3390/pharmaceutics14020464
- Jiang Y, Zhou DZ, Yang B. 3D bioprinted GelMA/GO composite induces osteoblastic differentiation. J Biomater Appl. 2022;37(3):527-537. doi: 10.1177/08853282221098235
- Koch L, Michael S, Reimers K, Strauß S, Vogt PM, Chichkov B. Bioprinting for skin. In: 3D Bioprinting and Nanotechnology in Tissue Engineering and Regenerative Medicine. Elsevier;2022(9):397-425. doi: 10.1016/B978-0-12-824552-1.00011-6
- McMillan A, McMillan N, Gupta N, Kanotra SP, Salem AK. 3D bioprinting in otolaryngology: a review. Adv Healthc Mater. 2023;3268-3284. doi: 10.1002/adhm.202203268
- Shen MK, Wang LL, Gao Y, et al. 3D bioprinting of in situ vascularized tissue engineered bone for repairing large segmental bone defects. Mater Today Bio. 2022;16 100382. doi: 10.1016/j.mtbio.2022.100382
- Prabhakaran P, Palaniyandi T, Kanagavalli B, et al. Prospect and retrospect of 3D bio-printing. Acta Histochem. 2022;124(7):151932. doi: 10.1016/j.acthis.2022.151932
- Banerjee D, Singh YP, Datta P, et al. Strategies for 3D bioprinting of spheroids: a comprehensive review. Biomaterials. 2022;291:121881. doi: 10.1016/j.biomaterials.2022.121881
- Blevins KM, Danilkowicz RM, Fletcher AN, Allen NB, Johnson LG, Adams SB. In situ 3D bioprinting of musculoskeletal tissues in orthopedic surgery. J 3D Print Med. 2022;6(1):25-36. doi: 10.2217/3dp-2021-0022
- Mohammadrezaei D, Moghimi N, Vandvajdi S, Powathil G, Hamis S, Kohandel M. Predicting and elucidating the post-printing behavior of 3D printed cancer cells in hydrogel structures by integrating in-vitro and in-silico experiments. Sci Rep. 2023;13(1):1211-1223. doi: 10.1038/s41598-023-28286-9
- Alheib O, da Silva LP, Youn YH, Kwon IK, Reis RL, Correlo VM. 3D bioprinting of gellan gum‐based hydrogels tethered with laminin‐derived peptides for improved cellular behavior. J Biomed Mater Res Part A. 2022;110(10): 1655-1668. doi: 10.1002/jbm.a.37415
- Lovecchio J, Cortesi M, Zani M, Govoni M, Dallari D, Giordano E. Fiber thickness and porosity control in a biopolymer scaffold 3D printed through a converted commercial FDM device. Materials. 2022;15(7):2394-2403. doi: 10.3390/ma15072394
- Koch F, Thaden O, Conrad S, et al. Mechanical properties of polycaprolactone (PCL) scaffolds for hybrid 3D-bioprinting with alginate-gelatin hydrogel. J Mech Behav Biomed Mater. 2022;130:105219. doi: 10.1016/j.jmbbm.2022.105219
- Soni VK, Sinha SK, Sharma HK. A review on 3D bioprinting materials and technique. J Phase Change Mater. 2022;2(2):7-24. doi: 10.58256/jpcm.v2i2.26
- Kantaros A. 3D printing in regenerative medicine: technologies and resources utilized. Int J Mol Sci. 2022;23(23): 14621-14633. doi: 10.3390/ijms232314621
- Yang XT, Ma Y, Wang XT, et al. A 3D‐bioprinted functional module based on decellularized extracellular matrix bioink for periodontal regeneration. Adv Sci. 2023;10(5): 5041-5058. doi: 10.1002/advs.202205041
- Zhang W, Ye WB, Yan YF. Advances in photocrosslinkable materials for 3D bioprinting. Adv Eng Mater. 2022;24(1): 663-675. doi: 10.1002/adem.202100663
- Zhu J, Wu PW, Chao YH, et al. Recent advances in 3D printing for catalytic applications. Chem Eng J. 2022;433:134341. doi: 10.1016/j.cej.2021.134341
- Vrana NE, Gupta S, Mitra K, et al. From 3D printing to 3D bioprinting: the material properties of polymeric material and its derived bioink for achieving tissue specific architectures. Cell Tissue Bank. 2022;27:417-440. doi: 10.1007/s10561-021-09975-z
- Jandyal A, Chaturvedi I, Wazir I, Raina A, Haq MIU. 3D printing–A review of processes, materials and applications in industry 4.0. Sustain Operation Comput. 2022;3:33-42. doi: 10.1016/j.susoc.2021.09.004
- Yap YL, Toh W, Giam A, et al. Topology optimization and 3D printing of micro-drone: numerical design with experimental testing. Int J Mech Sci. 2023;237:107771. doi: 10.1016/j.ijmecsci.2022.107771
- Tao J, Zhu SY, Liao XY, et al. DLP-based bioprinting of void-forming hydrogels for enhanced stem-cell-mediated bone regeneration. Mater Today Bio. 2022;17:100487. doi: 10.1016/j.mtbio.2022.100487
- Daikuara LY, Chen XF, Yue ZL, et al. 3D bioprinting constructs to facilitate skin regeneration. Adv Funct Mater. 2022;32(3):5080-5103. doi: 10.1002/adfm.202105080
- Noroozi R, Shamekhi MA, Mahmoudi R, et al. In vitro static and dynamic cell culture study of novel bone scaffolds based on 3D-printed PLA and cell-laden alginate hydrogel. Biomed Mater. 2022;17(4):045024. doi: 10.1088/1748-605x/ac7308
- Wang YM, Xu XY, Chen XY, Li JS. Multifunctional biomedical materials derived from biological membranes. Adv Mater. 2022;34(46):7406-7432. doi: 10.1002/adma.202107406
- Cheng W, Zhu Y, Jiang GY, et al. Sustainable cellulose and its derivatives for promising biomedical applications. Prog Mater Sci. 2023;138:101152. doi: 10.1016/j.pmatsci.2023.101152
- Markstedt K, Mantas A, Tournier I, Avila HM, Hagg D, Gatenholm P. 3D bioprinting human chondrocytes with nanocellulose–alginate bioink for cartilage tissue engineering applications. Biomacromolecules. 2015;16(5):1489-1496. doi: 10.1021/acs.biomac.5b00188
- Sydney Gladman A, Matsumoto EA, Nuzzo RG, Mahadevan L, Lewis JA. Biomimetic 4D printing. Nat Mater. 2016;15(4):413-418. doi: 10.1038/nmat4544
- Lee S, Hao LT, Park J, Oh DX, Hwang DS. Nanochitin and nanochitosan: chitin nanostructure engineering with multiscale properties for biomedical and environmental applications. Adv Mater. 2023;35(4):3325-3360. doi: 10.1002/adma.202203325
- Deng LZ, Zhang LM. Rheological characteristics of chitin/ionic liquid gels and electrochemical properties of regenerated chitin hydrogels. Colloids Surf A: Physicochem Eng Asp. 2020;586:124220. doi: 10.1016/j.colsurfa.2019.124220
- Kotla NG, Mohd Isa IL, Larrañaga A, et al. Hyaluronic acid‐based bioconjugate systems, scaffolds, and their therapeutic potential. Adv Healthc Mater. 2023;2203104. doi: 10.1002/adhm.202203104
- Le HV, Cerf DL. Colloidal polyelectrolyte complexes from hyaluronic acid: preparation and biomedical applications. Small. 2022;18(51):4283-4308. doi: 10.1002/smll.202204283
- Park KH, Ryu B, Song JJ, et al. Hyaluronic acid microparticles for effective spheroid culture and transplantation in liver tissue. Chem Eng J. 2023;464:142666. doi: 10.1016/j.cej.2023.142666
- Chen QX, Lu YH, Zhang JP, et al. Flexible structural polyethylene films for dynamically tunable energy harvesting from the sun and outer space. Nano Energy. 2023;114:108610. doi: 10.1016/j.nanoen.2023.108610
- Zhang XQ, Shen RF, Guo XJ, et al. Bimetallic Ag-Cu nanoparticles anchored on polypropylene (PP) nonwoven fabrics: superb catalytic efficiency and stability in 4-nitrophenol reduction. Chem Eng J. 2021;408:128018. doi: 10.1016/j.cej.2020.128018
- Wang LW, Liu Y, Han Q, Lin HB, Liu F. A novel poly (4-methyl-1-pentene)/polypropylene (PMP/PP) thin film composite (TFC) artificial lung membrane for enhanced gas transport and excellent hemo-compatibility. J Membr Sci. 2022;649:120359. doi: 10.1016/j.memsci.2022.120359
- Yang L, Sheng L, Gao XX, et al. rGO/Li-Al-LDH composite nanosheets modified commercial polypropylene (PP) separator to suppress lithium dendrites for lithium metal battery. Electrochim Acta. 2022;430:141073. doi: 10.1016/j.electacta.2022.141073
- Pasternak G, Ormeno-Cano N, Rutkowski P. Recycled waste polypropylene composite ceramic membranes for extended lifetime of microbial fuel cells. Chem Eng J. 2021;425:130707. doi: 10.1016/j.cej.2021.130707
- Smith P, Obando AG, Griffin A, Robertson M, Bounds E, Qiang Z. Additive manufacturing of carbon using commodity polypropylene. Adv Mater. 2023;35(17): 8029-8040. doi: 10.1002/adma.202208029
- Huang JJ, Zhang XD, Liu RX, Ding YH Guo DJ. Polyvinyl chloride-based dielectric elastomer with high permittivity and low viscoelasticity for actuation and sensing. Nat Commun. 2023;14(1):1483-1492. doi: 10.1038/s41467-023-37178-5
- Park H, Oh SJ, Kim M, et al. Plasticizer structural effect for sustainable and high-performance PVC gel-based triboelectric nanogenerators. Nano Energy. 2023;114:108615. doi: 10.1016/j.nanoen.2023.108615
- He QS, Zhong QY, Sun Z, et al. Highly stretchable, repeatable, and easy-to-prepare ionogel based on polyvinyl chloride for wearable strain sensors. Nano Energy. 2023;113:108535. doi: 10.1016/j.nanoen.2023.108535
- Choi DS, Bae JW, Lee SH, et al. Emotion-interactive empathetic transparent skin cushion with tailored frequency-dependent hydrogel–plasticized nonionic polyvinyl chloride interconnections. Chem Eng J. 2022;442:136142. doi: 10.1016/j.cej.2022.136142
- Park H, Oh SJ, Kim D, et al. Plasticized PVC‐gel single layer‐based stretchable triboelectric nanogenerator for harvesting mechanical energy and tactile sensing. Adv Sci. 2022;9(22):1070-1078. doi: 10.1002/advs.202201070
- Liu ZW, Li BB, Liu YD, Liang YR. Integrated bending actuation and the self‐sensing capability of poly (vinyl chloride) gels with ionic liquids. Adv Funct Mater. 2022;32(35):4259-4270. doi: 10.1002/adfm.202204259
- Ye HR, Jiang JX, Yang Y, et al. Ultra-strong and environmentally friendly waste polyvinyl chloride/paper biocomposites. Adv Compos Hybrid Mater. 2023;6(2):81-92. doi: 10.1007/s42114-023-00664-x
- Liu DS, Cao YF, Jiang P, et al. Tough, transparent, and slippery PVA hydrogel led by syneresis. Small. 2023;19(14): 6819-6829. doi: 10.1002/smll.202206819
- Li MK, Sun YY, Feng DY, Ruan KP, Liu X, Gu JW. Thermally conductive polyvinyl alcohol composite films via introducing hetero-structured MXene@ silver fillers. Nano Res. 2023;16(5):7820-7828. doi: 10.1007/s12274-023-5594-1
- Qiu W, Gehlen J, Bernero M, et al. A synthetic dynamic polyvinyl alcohol photoresin for fast volumetric bioprinting of functional ultrasoft hydrogel constructs. Adv Funct Mater. 2023;33(20):14393-14403. doi: 10.1002/adfm.202214393
- Sanchis‐Gual R, Ye H, Ueno T, et al. 3D printed template‐assisted casting of biocompatible polyvinyl alcohol‐based soft microswimmers with tunable stability. Adv Funct Mater. 2023;2212952. doi: 10.1002/adfm.202212952
- Wei Y, Baskaran N, Wang HY, Su YC, Nabilla SC, Chung RJ. Study of polymethylmethacrylate/tricalcium silicate composite cement for orthopedic application. Biomed J. 2023;46(3):100540-100549. doi: 10.1016/j.bj.2022.05.005
- Wang Z, Yuh SJ, Renaud-Charest E, et al. Cervical spine reconstruction with chest tube technique after metastasis resection: a single-center experience. World Neurosurg. 2022;157:e49-e56. doi: 10.1016/j.wneu.2021.09.088
- Jian L. Effect of sizing agent on interfacial properties of carbon fiber-reinforced PMMA composite. Compos Adv Mater. 2021;30:2633366X20978657. doi: 10.1177/2633366x20978657
- Da HR, Pan SS, Li J, et al. Greatly recovered electrochemical performances of regenerated graphite anode enabled by an artificial PMMA solid electrolyte interphase layer. Energy Stor Mater. 2023;56:457-467. doi: 10.1016/j.ensm.2023.01.038
- Duan YX, Yang HB, Liu K, et al. 2023, Cellulose nanofibril aerogels reinforcing polymethyl methacrylate with high optical transparency, Advanced Composites and Hybrid Materials, Vol. 6 (3):123-134. doi: 10.1007/s42114-023-00700-w
- Hu Y, Lin XY, Liu DT, et al. Electrospun polymethyl methacrylate fibers-based membrane with heterogeneous structure achieving a full-particle size separation of oil-water emulsion. J Membr Sci. 2023;680:121716. doi: 10.1016/j.memsci.2023.121716
- Zafar MS. Prosthodontic applications of polymethyl methacrylate (PMMA): an update. Polymers. 2020;12(10):2299-2333. doi: 10.3390/polym12102299
- Kwak G, Jeong YS, Kim SW, et al. Hybrid photothermal structure based on Cr-MgF2 solar absorber/PMMA-graphene heat reservoir for enhanced thermoelectric power generation. Nano Energy. 2023;110:108352. doi: 10.1016/j.nanoen.2023.108352
- Backes EH, Harb SV, Beatrice CAG, et al. Polycaprolactone usage in additive manufacturing strategies for tissue engineering applications: a review. J Biomed Mater Res Part B: Appl Biomater. 2022;110(6): 1479-1503. doi: 10.1002/jbm.b.34997
- Yang YT, Wu HC, Fu QL, et al. 3D-printed polycaprolactone-chitosan based drug delivery implants for personalized administration. Mater Des. 2022;214:110394. doi: 10.1016/j.matdes.2022.110394
- Singh AP, Prakash O, Kumar S, Shukla A, Maiti P. Poly (lactic acid-co-glycolic acid) as sustained drug delivery vehicle for melanoma therapy. Mater Today Commun. 2022;31:103661. doi: 10.1016/j.mtcomm.2022.103661
- Lin JL, Huang JC, Wu J, Tang B, Li CB, Xiao HJ. Poly (lactic acid-co-glycolic acid)-based celecoxib extended-release microspheres for the local treatment of traumatic heterotopic ossification. J Biomater Appl. 2022;36(8): 1458-1468. doi: 10.1177/08853282211056937
- Diedkova K, Pogrebnjak AD, Kyrylenko S, et al. Polycaprolactone–MXene nanofibrous scaffolds for tissue engineering. ACS Appl Mater Interfaces. 2023;15(11): 14033-14047. doi: 10.1021/acsami.2c22780
- Wei JW, Xia X, Xiao SQ, et al. Sequential dual‐biofactor release from the scaffold of mesoporous HA microspheres and PLGA matrix for boosting endogenous bone regeneration. Adv Healthc Mater. 2023;624-637. doi: 10.1002/adhm.202300624
- Li PF, Ruan LM, Jiang GH, et al. Design of 3D polycaprolactone/ε-polylysine-modified chitosan fibrous scaffolds with incorporation of bioactive factors for accelerating wound healing. Acta Biomater. 2022;152: 197-209. doi: 10.1016/j.actbio.2022.08.075
- Huang YQ, Du ZY, Li K, et al. ROS-scavenging electroactive polyphosphazene-based core–shell nanofibers for bone regeneration. Adv Fiber Mater. 2022;4(4):894-907. doi: 10.1007/s42765-022-00153-8
- Long Q, Liu ZH, Shao QW, et al. Autologous skin fibroblast‐based PLGA nanoparticles for treating multiorgan fibrosis. Adv Sci. 2022;9(21):856-869. doi: 10.1002/advs.202200856
- Sheffey VV, Siew EB, Tanner EEL, Eniola-Adefeso O. PLGA’s plight and the role of stealth surface modification strategies in its use for intravenous particulate drug delivery. Adv Healthc Mater. 2022;11(8):1536-1569. doi: 10.1002/adhm.202101536
- Liu JJ, Qu SX, Suo ZG, Yang W. Functional hydrogel coatings. Natl Sci Rev. 2021;8(2):nwaa254. doi: 10.1093/nsr/nwaa254
- Sun X, Yao FL, Li JJ. Nanocomposite hydrogel-based strain and pressure sensors: a review. J Mater Chem A. 2020;8(36):18605-18623. doi: 10.1039/d0ta06965e
- Shen ZQ, Zhang ZL, Zhang NB, et al. High‐stretchability, ultralow‐hysteresis conductingpolymer hydrogel strain sensors for soft machines. Adv Mater. 2022;34(32): 3650-3657. doi: 10.1002/adma.202203650
- Mandal A, Clegg JR, Anselmo AC, Mitragotri S. Hydrogels in the clinic. Bioeng Transl Med. 2020;5(2):e10158. doi: 10.1002/btm2.10158
- Unagolla JM, Jayasuriya AC. Hydrogel-based 3D bioprinting: a comprehensive review on cell-laden hydrogels, bioink formulations, and future perspectives. Appl Mater Today. 2020;18:100479. doi: 10.1016/j.apmt.2019.100479
- Ye WL, Yang Z, Cao FY, et al. Articular cartilage reconstruction with TGF-β1-simulating self-assembling peptide hydrogel-based composite scaffold. Acta Biomater. 2022;146:94-106. doi: 10.1016/j.actbio.2022.05.012
- Sharma A, Panwar V, Mondal B, et al. Electrical stimulation induced by a piezo-driven triboelectric nanogenerator and electroactive hydrogel composite, accelerate wound repair. Nano Energy. 2022;99:107419. doi: 10.1016/j.nanoen.2022.107419
- Peng W, Li D, Dai KL, et al. Recent progress of collagen, chitosan, alginate and other hydrogels in skin repair and wound dressing applications. Int J Biol Macromol. 2022;208:400-408. doi: 10.1016/j.ijbiomac.2022.03.002
- Li N, Liu W, Zheng XY, et al. Antimicrobial hydrogel with multiple pH-responsiveness for infected burn wound healing. Nano Res. 2023;23(6):1-10. doi: 10.1007/s12274-023-5751-6
- Li HJ, Liang Y, Gao GR, et al. Asymmetric bilayer CNTs-elastomer/hydrogel composite as soft actuators with sensing performance. Chem Eng J. 2021;415:128988. doi: 10.1016/j.cej.2021.128988
- Choi MY, Shin Y, Lee HS, Kim SY, Na JH. Multipolar spatial electric field modulation for freeform electroactive hydrogel actuation. Sci Rep. 2020;10(1): 2482-2489. doi: 10.1038/s41598-020-59318-3
- Kim DI, Song S, Jang S, et al. Untethered gripper-type hydrogel millirobot actuated by electric field and magnetic field. Smart Mater Struct. 2020;29(8):085024. doi: 10.1088/1361-665x/ab8ea4
- Peng XW, Peng Q, Wu M, et al. A pH and temperature dual-responsive microgel-embedded, adhesive, and tough hydrogel for drug delivery and wound healing. ACS Appl Mater Interfaces. 2023;15(15):19560-19573. doi: 10.1021/acsami.2c21255
- Youness RA, Tag El-deen DM, Taha MA . A review on calcium silicate ceramics: properties, limitations, and solutions for their use in biomedical applications. Silicon. 2023;15(6):2493-2505. doi: 10.1007/s12633-022-02207-3
- Shu CQ, Qin C, Chen L, et al. Metal‐organic framework functionalized bioceramic scaffolds with antioxidative activity for enhanced osteochondral regeneration. Adv Sci. 2023;10(13):6875-6888. doi: 10.1002/advs.202206875
- Kost J, Huwyler J, Puchkov M. Calcium phosphate microcapsules as multifunctional drug delivery devices. Adv Funct Mater. 2023;202303333. doi: 10.1002/adfm.202303333
- Li M, Jiang JW, Liu WB, et al. Bioadaptable bioactive glass-β- tricalcium phosphate scaffolds with TPMS-gyroid structure by stereolithography for bone regeneration. J Mater Sci Technol. 2023;155:54-65. doi: 10.1016/j.jmst.2023.01.025
- Feng YHZ, Wu D, Knaus J, et al. A bioinspired gelatin– amorphous calcium phosphate coating on titanium implant for bone regeneration. Adv Healthc Mater. 2023; 3411-3423. doi: 10.1002/adhm.202203411
- Yuan G, Xu YN, Bai XP, et al. Autophagy-targeted calcium phosphate nanoparticles enable transarterial chemoembolization for enhanced cancer therapy. ACS Appl Mater Interfaces. 2023;15(9):11431-11443. doi: 10.1021/acsami.2c18267
- Zhang YG, Li JP, Soleimani M, et al. Biodegradable elastic sponge from nanofibrous biphasic calcium phosphate ceramic as an advanced material for regenerative medicine. Adv Funct Mater. 2021;31(40):2911-2923. doi: 10.1002/adfm.202102911
- Bartmański M, Rościszewska M, Wekwejt M, Ronowska A, Nadolska-Dawidowska M, Mielewczyk-Gryń A. Properties of new composite materials based on hydroxyapatite ceramic and cross-linked gelatin for biomedical applications. Int J Mol Sci. 2022;23(16):9083-9097. doi: 10.3390/ijms23169083
- Vaiani L, Boccaccio A, Uva AE, et al. Ceramic materials for biomedical applications: an overview on properties and fabrication processes. J Funct Biomater. 2023;14(3):146-167. doi: 10.3390/jfb14030146
- Rieu J, Goeuriot P. Ceramic composites for biomedical applications. Clin Mater. 1993;12(4):211-217. doi: 10.1016/0267-6605(93)90075-I
- Swarte JC, Li YN, Hu SX, et al. Gut microbiome dysbiosis is associated with increased mortality after solid organ transplantation. Sci Transl Med. 2022;14(660):eabn7566. doi: 10.1126/scitranslmed.abn7566
- Duneton C, Winterberg PD, Ford ML. Activation and regulation of alloreactive T cell immunity in solid organ transplantation. Nat Rev Nephrol. 2022;18(10):663-676. doi: 10.1038/s41581-022-00600-0
- Xu KL, Han Y, Huang YY, Wei P, Yin J, Jiang JY. The application of 3D bioprinting in urological diseases. Mater Today Bio. 2022;100388. doi: 10.1016/j.mtbio.2022.100388
- Zennifer A, Manivannan S, Sethuraman S, Kumbar SG, Sundaramurthi D. 3D bioprinting and photocrosslinking: emerging strategies & future perspectives. Biomater Adv. 2022;134:112576-112599. doi: 10.1016/j.msec.2021.112576
- Bose S, Roy M, Bandyopadhyay A. Recent advances in bone tissue engineering scaffolds. Trends Biotechnol. 2012;30(10):546-554. doi: 10.1016/j.tibtech.2012.07.005
- Bose S, Vahabzadeh S, Bandyopadhyay A. Bone tissue engineering using 3D printing. Mater Today. 2013;16(12):496-504. doi: 10.1016/j.mattod.2013.11.017
- Ma ZC, Zhang YL, Han B, et al. Femtosecond laser programmed artificial musculoskeletal systems. Nat Commun. 2020;11(1):4536-4545. doi: 10.1038/s41467-020-18117-0
- Huang HJ, Xing WR, Zeng CJ, Huang WH. Pararectus approach combined with three-dimensional printing for anterior plate fixation of sacral fractures. Injury. 2021;52(10):2719-2724. doi: 10.1016/j.injury.2020.03.049
- Strehin I, Nahas Z, Arora K, Nguyen T, Elisseeff J. A versatile pH sensitive chondroitin sulfate-PEG tissue adhesive and hydrogel. Biomaterials. 2010;31(10):2788-2797. doi: 10.1016/j.biomaterials.2009.12.033
- Decarli MC, Seijas-Gamardo A, Morgan FLC, et al. Bioprinting of stem cell spheroids followed by post-printing chondrogenic differentiation for cartilage tissue engineering. Adv Healthc Mater. 2023;2203021. doi: 10.1002/adhm.202203021
- Liu DH, Nie W, Li DJ, et al. 3D printed PCL/SrHA scaffold for enhanced bone regeneration. Chem Eng J. 2019;362:269-279. doi: 10.1016/j.cej.2019.01.015
- Yan YF, Chen H, Zhang HB, et al. Vascularized 3D printed scaffolds for promoting bone regeneration. Biomaterials. 2019;190:97-110. doi: 10.1016/j.biomaterials.2018.10.033
- Gold KA, Saha B, Pandian NKR, et al. 3D bioprinted multicellular vascular models. Adv Healthc Mater. 2021;10(21):1141-1154. doi: 10.1002/adhm.202101141
- Fang YC, Guo YH, Wu BY, et al. Expanding embedded 3D bioprinting capability for engineering complex organs with freeform vascular networks. Adv Mat. 2023;35(22): 5082-5096. doi: 10.1002/adma.202205082
- Moore MJ, Tan RP, Yang NJ, Rnjak-Kovacina J, Wise SG. Bioengineering artificial blood vessels from natural materials. Trends Biotechnol. 2022;40(6):693-707. doi: 10.1016/j.tibtech.2021.11.003
- Silberman E, Oved H, Namestnikov M, Shapira A, Dvir T. Post‐maturation reinforcement of 3d‐printed vascularized cardiac tissues. Adv Mater. 2023;2302229. doi: 10.1002/adma.202302229
- Song BW, Wang CW, Fan SY, et al. Rapid construction of 3D biomimetic capillary networks with complex morphology using dynamic holographic processing. Adv Funct Mater. 2023;2305245. doi: 10.1002/adfm.202305245
- Radenkovic D, Solouk A, Seifalian A. Personalized development of human organs using 3D printing technology. Med Hypotheses. 2016;87:30-33. doi: 10.1016/j.mehy.2015.12.017
- Yuan XM, Zhu Z, Xia PC, et al. Tough gelatin hydrogel for tissue engineering. Adv Sci. 2023;2301665. doi: 10.1002/advs.202301665
- Wang YF, Zheng Q, Sun Z, et al. Reversal of liver failure using a bioartificial liver device implanted with clinical-grade human-induced hepatocytes. Cell Stem Cell. 2023;30(5):617-631. doi: 10.1016/j.stem.2023.03.013
- Liun C, Lou YT, Sun ZY, et al. 4D printing of personalized-tunable biomimetic periosteum with anisotropic microstructure for accelerated vascularization and bone healing. Adv Healthc Mater. 2023;12(22):2202868. doi: 10.1002/adhm.202202868
- Ahrens JH, Uzel SGM, Skylar-Scott M, et al. Programming cellular alignment in engineered cardiac tissue via bioprinting anisotropic organ building blocks. Adv Mater. 2022;34(26):217-227. doi: 10.1002/adma.202200217
- Noor N, Shapira A, Edri R, Gal I, Wertheim L, Dvir T. 3D printing of personalized thick and perfusable cardiac patches and hearts. Adv Sci. 2019;6(11):344-353. doi: 10.1002/advs.201900344
- Ng WL, Qi JTZ, Yeong WY, Naing MW. Proof-of-concept: 3D bioprinting of pigmented human skin constructs. Biofabrication. 2018;10(2):025005. doi: 10.1088/1758-5090/aa9e1e
- Hakimi N, Cheng R, Leng L, et al. Handheld skin printer: in situ formation of planar biomaterials and tissues. Lab Chip. 2018;18(10):1440-1451. doi: 10.1039/c7lc01236e
- Kim BS, Gao G, Kim JY, Cho DW. 3D cell printing of perfusable vascularized human skin equivalent composed of epidermis, dermis, and hypodermis for better structural recapitulation of native skin. Adv Healthc Mater. 2019; 8(7):1019-1029. doi: 10.1002/adhm.201801019
- Cubo N, Garcia M, Cañizo JFD, Velasco D, Jorcano JL. 3D bioprinting of functional human skin: production and in vivo analysis. Biofabrication. 2016;9(1):015006. doi: 10.1088/1758-5090/9/1/015006
- Ma J, Qin C, Wu JF, et al. 3D printing of strontium silicate microcylinder‐containing multicellular biomaterial inks for vascularized skin regeneration. Adv Healthc Mater. 2021;10(16):523-536. doi: 10.1002/adhm.202100523
- Zhang DQ, Lai LY, Fu HJ, Fu Q, Chen ML. 3D-bioprinted biomimetic multilayer implants comprising microfragmented adipose extracellular matrix and cells improve wound healing in a murine model of full-thickness skin defects. ACS Appl Mater Interfaces. 2023;15:29713-29728. doi: 10.1021/acsami.2c21629
- Pourchet LJ, Thepot A, Albouy M, et al. Human skin 3D bioprinting using scaffold‐free approach. Adv Healthc Mater. 2017;6(4):1101-1107. doi: 10.1002/adhm.201601101
- Bose S, Darsell J, Kintner M , Hosick H, Bandyopadhyay A. Ore size and pore volume effects on alumina and TCP ceramic scaffolds. Mater Sci Eng C. 2003;23:479-486. doi: 10.1016/s0928-4931(02)00129-7
- Bose S, Ke D, Sahasrabudhe H, Bandyopadhyay A. Additive manufacturing of biomaterials. Prog Mater Sci. 2018;93:45-111. doi: 10.1016/j.pmatsci.2017.08.003
- Han J, Jeong W, Kim MK, Nam SH, Park EK, Kang HW. Demineralized dentin matrix particle-based bio-ink for patient-specific shaped 3D dental tissue regeneration. Polymers. 2021;13(8):1294-1307. doi: 10.3390/polym13081294
- Nejad ZM, Zamanian A, Saeidifar M, Vanaei HR, Amoli MS. 3D bioprinting of polycaprolactone-based scaffolds for pulp-dentin regeneration: investigation of physicochemical and biological behavior. Polymers. 2021;13(24):4442-4455. doi: 10.3390/polym13244442
- Lin YT, Hsu YT, Liu YW, Kao CT, Huang TH. Bidirectional differentiation of human-derived stem cells induced by biomimetic calcium silicate-reinforced gelatin methacrylate bioink for odontogenic regeneration. Biomedicines. 2021;9(8):929-945. doi: 10.3390/biomedicines9080929
- Wang CY, Chiu YC, Lee AKX, Lin YA, Lin PY, Shie MY. Biofabrication of gingival fibroblast cell-laden collagen/ strontium-doped calcium silicate 3D-printed bi-layered scaffold for osteoporotic periodontal regeneration. Biomedicines. 2021;9(4):431-448. doi: 10.3390/biomedicines9040431
- Kim D, Lee H, Lee GH, Hoang TH, Kim HR, Kim GH. Fabrication of bone‐derived decellularized extracellular matrix/ceramic‐based biocomposites and their osteo/ odontogenic differentiation ability for dentin regeneration. Bioeng Transl Med. 2022;7(3):e10317. doi: 10.1002/btm2.10317
- Choi YJ, Jun YJ, Kim DY, et al. A 3D cell printed muscle construct with tissue-derived bioink for the treatment of volumetric muscle loss. Biomaterials. 2019;206: 160-169. doi: 10.1016/j.biomaterials.2019.03.036
- Ribezzi D, Gueye M, Florczak S, et al. Shaping synthetic multicellular and complex multimaterial tissues via embedded extrusion‐volumetric printing of microgels. Adv Mater. 2023;2301673. doi: 10.1002/adma.202301673
- Ali M, Pr AK, Yoo JJ, Zahran F, Atala A, Lee SJ. A photo‐crosslinkable kidney ECM‐derived bioink accelerates renal tissue formation. Adv Healthc Mater. 2019;8(7):992-1001. doi: 10.1002/adhm.201800992
- Singh NK, Han W, Nam SA, et al. Three-dimensional cell-printing of advanced renal tubular tissue analogue. Biomaterials. 2020;23(2):119734-119748. doi: 10.1016/j.biomaterials.2019.119734
- Kim W, Lee H, Lee J, et al. Efficient myotube formation in 3D bioprinted tissue construct by biochemical and topographical cues. Biomaterials. 2020;230:119632-119647. doi: 10.1016/j.biomaterials.2019.119632
- Zhang PF, Abate AR. High‐definition single‐cell printing: cell‐by‐cell fabrication of biological structures. Adv Mater. 2020;32(52):5346-5354. doi: 10.1002/adma.202005346