Decellularized porcine kidney-incorporated hydrogels for cell-laden bioprinting of renal cell carcinoma model
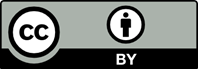
More than 90% of kidney cancers are attributed to renal cell carcinoma (RCC), which is however highly resistant to traditional chemotherapy. The challenges met in treating RCC signify an imperative to accelerate the development of new and effective drugs. Preclinical testing has served as a foundation for evaluating potential effectiveness of new drugs, but this endeavor is deeply restricted by the current generation of in vitro two-dimensional culture models, which cannot accurately mimic the tumor microenvironment (TME). Therefore, new in vitro three-dimensional (3D) cell culture models that can better mimic the components and architecture of TME have been developed for preclinical testing, but only a few existing 3D cell culture models can simulate the TME of RCC, representing a limitative obstacle impeding the development of novel drugs for RCC. In this study, we prepared a bioink by mixing porcine kidney decellularized extracellular matrix (dECM) powders with gelatin methacryloyl (GelMA) to bioprint an in vitro 3D cell culture model for RCC. We found that GelMA stability, mechanical properties, and printability were all significantly improved following the addition of the dECM powder. Moreover, cell cultures using ACHN cells suggested that kidney dECM powders significantly improved the cellular proliferation and metastasis via upregulation of markers related to epithelial– mesenchymal transition, along with activation of several cancer progression-related signaling pathways. More importantly, ACHN cells also demonstrated higher resistance to sunitinib under the stimulation of kidney dECM, indicating that GelMA-kidney dECM hydrogels may be an appropriate preclinical model to be used for building an in vitro RCC platform for drug screening and development.
- Capitanio U, Bensalah K, Bex A, et al. Epidemiology of renal cell carcinoma. Eur Urol. 2019;75(1):74-84. doi: 10.1016/j.eururo.2018.08.036
- Siegel RL, Miller KD, Fuchs HE, Jemal A. Cancer statistics, 2022. CA Cancer J Clin. 2022;72(1):7-33. doi: 10.3322/caac.21708
- Safiri S, Kolahi AA, Mansournia MA, et al. The burden of kidney cancer and its attributable risk factors in 195 countries and territories, 1990-2017. Sci Rep. 2020;10(1):13862. doi: 10.1038/s41598-020-70840-2
- Makhov P, Joshi S, Ghatalia P, et al. Resistance to systemic therapies in clear cell renal cell carcinoma: Mechanisms and management strategies. Mol Cancer Therap. 2018;17(7): 1355-1364. doi: 10.1158/1535-7163.MCT-17-1299
- Nerich V, Hugues M, Paillard MJ, et al. Clinical impact of targeted therapies in patients with metastatic clear-cell renal cell carcinoma. Onco Targets Ther. 2014;7:365-374. doi: 10.2147/OTT.S56370
- Goyal R, Gersbach E, Yang XJ, Rohan SM. Differential diagnosis of renal tumors with clear cytoplasm: Clinical relevance of renal tumor subclassification in the era of targeted therapies and personalized medicine. Arch Pathol Lab Med. 2013;137(4):467-480. doi: 10.5858/arpa.2012-0085-RA
- Hui L, Chen Y. Tumor microenvironment: Sanctuary of the devil. Cancer Lett. 2015;368(1):7-13. doi: 10.1016/j.canlet.2015.07.039
- Jonasch E, Walker CL, Rathmell WK. Clear cell renal cell carcinoma ontogeny and mechanisms of lethality. Nat Rev Nephrol. 2021;17(4):245-261. doi: 10.1038/s41581-020-00359-2
- Vuong L, Kotecha RR, Voss MH, Hakimi AA. Tumor microenvironment dynamics in clear-cell renal cell carcinoma. Cancer Discov. 2019;9(10):1349-1357. doi: 10.1158/2159-8290.CD-19-0499
- Meszaros M, Yusenko M, Domonkos L, Peterfi L, Kovacs G, Banyai D. Expression of TXNIP is associated with angiogenesis and postoperative relapse of conventional renal cell carcinoma. Sci Rep. 2021;11(1):17200. doi: 10.1038/s41598-021-96220-y
- Li S, Huang C, Hu G, et al. Tumor-educated B cells promote renal cancer metastasis via inducing the IL-1β/HIF-2α/ Notch1 signals. Cell Death Dis. 2020;11(3):163. doi: 10.1038/s41419-020-2355-x
- Frantz C, Stewart K, Weaver V. The extracellular matrix at a glance. J Cell Sci. 2010;123:4195-4200. doi: 10.1242/jcs.023820
- Majo S, Courtois S, Souleyreau W, Bikfalvi A, Auguste P. Impact of extracellular matrix components to renal cell carcinoma behavior. Front Oncol. 2020;10:625. doi: 10.3389/fonc.2020.00625
- Afify A, Purnell P, Nguyen L. Role of CD44s and CD44v6 on human breast cancer cell adhesion, migration, and invasion. Exp Mol Pathol. 2009;86(2):95-100. doi: 10.1016/j.yexmp.2008.12.003
- Theocharis A. Versican in health and disease. Connect Tissue Res. 2008;49(3):230-234. doi: 10.1080/03008200802147571
- Marinkovich M. Tumour microenvironment: Laminin 332 in squamous-cell carcinoma. Nat Rev Cancer. 2007;7(5): 370-380. doi: 10.1038/nrc2089
- Astrof S, Hynes R. Fibronectins in vascular morphogenesis. Angiogenesis. 2009;12(2):165-175. doi: 10.1007/s10456-009-9136-6
- Hielscher A, Ellis K, Qiu C, Porterfield J, Gerecht S. Fibronectin deposition participates in extracellular matrix assembly and vascular morphogenesis. PloS One. 2016;11(1): e0147600. doi: 10.1371/journal.pone.0147600
- Unal A, West J. Synthetic ECM: Bioactive synthetic hydrogels for 3D tissue engineering. Bioconjug Chem. 2020;31(10):2253-2271. doi: 10.1021/acs.bioconjchem.0c00270
- Kabirian F, Mozafari M. Decellularized ECM-derived bioinks: Prospects for the future. Methods (San Diego, Calif.). 2020;171:108-118. doi: 10.1016/j.ymeth.2019.04.019
- Fontana F, Marzagalli M, Sommariva M, Gagliano N, Limonta P. In vitro 3D cultures to model the tumor microenvironment. Cancers (Basel). 2021;13(12).doi: 10.3390/cancers13122970
- Habanjar O, Diab-Assaf M, Caldefie-Chezet F, Delort L. 3D cell culture systems: Tumor application, advantages, and disadvantages. Int J Mol Sci. 2021;22(22). doi: 10.3390/ijms222212200
- Monteiro M, Gaspar V, Ferreira L, Mano J. Hydrogel 3D in vitro tumor models for screening cell aggregation mediated drug response. Biomater Sci. 2020;8(7):1855-1864. doi: 10.1039/c9bm02075f
- Pan T, Fong EL, Martinez M, et al. Three-dimensional (3D) culture of bone-derived human 786-O renal cell carcinoma retains relevant clinical characteristics of bone metastases. Cancer Lett. 2015;365(1):89-95. doi: 10.1016/j.canlet.2015.05.019
- Maliszewska-Olejniczak K, Brodaczewska KK, Bielecka ZF, et al. Development of extracellular matrix supported 3D culture of renal cancer cells and renal cancer stem cells. Cytotechnology. 2019;71(1):149-163. doi: 10.1007/s10616-018-0273-x
- Liu K, Cui JJ, Zhan Y, et al. Reprogramming the tumor microenvironment by genome editing for precision cancer therapy. Mol Cancer. 2022;21(1):98-121. doi: 10.1186/s12943-022-01561-5
- Nallasamy P, Nimmakayala RK, Parte S, Are AC, Batra SK, Ponnusamy MP. Tumor microenvironment enriches the stemness features: The architectural event of therapy resistance and metastasis. Mol Cancer. 2022;21(1):225-250. doi: 10.1186/s12943-022-01682-x
- Zhang X, Chen X, Hong H, Hu R, Liu J, Liu C. Decellularized extracellular matrix scaffolds: Recent trends and emerging strategies in tissue engineering. Bioact Mater. 2022;10:15-31. doi: 10.1016/j.bioactmat.2021.09.014
- Zhang W, Du A, Liu S, Lv M, Chen S. Research progress in decellularized extracellular matrix-derived hydrogels. Regen Ther. 2021;18:88-96. doi: 10.1016/j.reth.2021.04.002
- Yue K, Trujillo-de Santiago G, Alvarez M, Tamayol A, Annabi N, Khademhosseini A. Synthesis, properties, and biomedical applications of gelatin methacryloyl (GelMA) hydrogels. Biomaterials. 2015;73:254-271. doi: 10.1016/j.biomaterials.2015.08.045
- Wang F, Zhang R, Gao N, et al. Coagulation-anticoagulation-regulable and tough extracellular matrix hydrogels. Compos Part B: Eng. 2022;239: 109938. doi: 10.1016/j.compositesb.2022.109938
- Yin J, Yan M, Wang Y, Fu J, Suo H. 3D bioprinting of low-concentration cell-laden gelatin methacrylate (GelMA) bioinks with a two-step cross-linking strategy. ACS Appl Mater Interfaces. 2018;10(8):6849-6857. doi: 10.1021/acsami.7b16059
- Li Y, Mao Q, Yin J, Wang Y, Fu J, Huang Y. Theoretical prediction and experimental validation of the digital light processing (DLP) working curve for photocurable materials. Addit Manuf. 2021;37. doi: 10.1016/j.addma.2020.101716
- Habib A, Sathish V, Mallik S, Khoda B. 3D printability of alginate-carboxymethyl cellulose hydrogel. Materials (Basel). 2018;11(3). doi: 10.3390/ma11030454
- Ouyang L, Yao R, Zhao Y, Sun W. Effect of bioink properties on printability and cell viability for 3D bioplotting of embryonic stem cells. Biofabrication. 2016; 8(3):035020. doi: 10.1088/1758-5090/8/3/035020
- Ljungberg N, Bonini C, Bortolussi F, Boisson C, Heux L, Cavaille JY. New nanocomposite materials reinforced with cellulose whiskers in atactic polypropylene effect of surface and dispersion characteristics. Biomacromolecules. 2005;6:2732-2739. doi: 10.1021/bm050222v
- Shin MK, Spinks GM, Shin SR, Kim SI, Kim S. Nanocomposite hydrogel with high toughness for bioactuators. Adv Mater. 2009;21:1712-1715. doi: 10.1002/adma.200802205
- Huang K, Gu Z, Wu J. Tofu-incorporated hydrogels for potential bone regeneration. ACS Biomater Sci Eng. 2020;6(5): 3037-3045. doi: 10.1021/acsbiomaterials.9b01997
- Gao C, Sow WT, Wang Y, et al. Hydrogel composite scaffolds with an attenuated immunogenicity component for bone tissue engineering applications. J Mater Chem B. 2021;9(8):2033-2041. doi: 10.1039/d0tb02588g
- Dongre A, Weinberg RA. New insights into the mechanisms of epithelial-mesenchymal transition and implications for cancer. Nat Rev Mol Cell Biol. 2019;20(2): 69-84. doi: 10.1038/s41580-018-0080-4
- Feng D, Gao P, Henley N, et al. SMOC2 promotes an epithelial-mesenchymal transition and a pro-metastatic phenotype in epithelial cells of renal cell carcinoma origin. Cell Death Dis. 2022;13(7): 639-654. doi: 10.1038/s41419-022-05059-2
- Zhong M, Zhu M, Liu Y, et al. TNFAIP8 promotes the migration of clear cell renal cell carcinoma by regulating the EMT. J Cancer. 2020;11(10):3061-3071. doi: 10.7150/jca.40191
- Mikami S, Katsube K, Oya M, et al. Expression of Snail and Slug in renal cell carcinoma: E-cadherin repressor Snail is associated with cancer invasion and prognosis. Lab Invest. 2011;91(10):1443-1458. doi: 10.1038/labinvest.2011.111
- Liu W, Liu Y, Liu H, Zhang W, An H, Xu J. Snail predicts recurrence and survival of patients with localized clear cell renal cell carcinoma after surgical resection. Urol Oncol. 2015;33(2):69e61-10. doi: 10.1016/j.urolonc.2014.08.003
- Harada K, Miyake H, Kusuda Y, Fujisawa M. Expression of epithelial-mesenchymal transition markers in renal cell carcinoma: Impact on prognostic outcomes in patients undergoing radical nephrectomy. BJU Int. 2012;110 (11 Pt C):E1131-1137. doi: 10.1111/j.1464-410X.2012.11297.x
- Rasti A, Madjd Z, Abolhasani M, et al. Cytoplasmic expression of Twist1, an EMT-related transcription factor, is associated with higher grades renal cell carcinomas and worse progression-free survival in clear cell renal cell carcinoma. Clin Exp Med. 2018;18(2):177-190. doi: 10.1007/s10238-017-0481-2
- Kugler A, Hemmerlein B, Thelen P, Kallerhoff M, Radzun HJ, Ringert RH. Expression of metalloproteinase 2 and 9 and their inhibitors in renal cell carcinoma. J Urol. 1998;160(5):1914-1918. doi: 10.1016/s0022-5347(01)62443-1
- Chambers AF, Matrisian LM. Changing views of the role of matrix metalloproteinases in metastasis. J Natl Cancer Inst. 1997;89(17):1260-1270. doi: 10.1093/jnci/89.17.1260
- Xu H, Xu W-H, Ren F, et al. Prognostic value of epithelial-mesenchymal transition markers in clear cell renal cell carcinoma. Aging. 2020;12(1):866-883. doi: 10.18632/aging.102660
- Sugimoto M, Kohashi K, Itsumi M, et al. Epithelial to mesenchymal transition in clear cell renal cell carcinoma with rhabdoid features. Pathobiology. 2016;83(6):277-286. doi: 10.1159/000445752
- Li X, Ma X, Chen L, et al. Prognostic value of CD44 expression in renal cell carcinoma: A systematic review and meta-analysis. Sci Rep. 2015;5:13157. doi: 10.1038/srep13157
- Yao JX, Chen X,. Zhu YJ, Wang H, Hu XY, Guo JM. Prognostic value of vimentin is associated with immunosuppression in metastatic renal cell carcinoma. Front Oncol. 2020;10:1181-1190. doi: 10.3389/fonc.2020.01181
- Fiedorowicz M, Khan MI, Strzemecki D, et al. Renal carcinoma CD105-/CD44- cells display stem-like properties in vitro and form aggressive tumors in vivo. Sci Rep. 2020;10(1):5379. doi: 10.1038/s41598-020-62205-6
- Katagiri A, Watanabe R, Tomita Y. E-cadherin expression in renal cell cancer and its significance in metastasis and survival. Br J Cancer. 1995;71(2):376-379. doi: 10.1038/bjc.1995.76
- Tani T, Laitinen L, Kangas L, Lehto VP, Virtanen I. Expression of E- and N-cadherin in renal cell carcinomas, in renal cell carcinoma cell lines in vitro and in their xenografts. Int J Cancer. 1995;64(6):407-414. doi: 10.1002/ijc.2910640610
- Gao K, Zhang F, Chen K, et al. Expression patterns and prognostic value of RUNX genes in kidney cancer. Sci Rep. 2021;11(1):14934. doi: 10.1038/s41598-021-94294-2
- Rooney N, Mason SM, McDonald L, et al. RUNX1 is a driver of renal cell carcinoma correlating with clinical outcome. Cancer Res. 2020;80(11):2325-2339. doi: 10.1158/0008-5472.CAN-19-3870
- Gong D, Zhang J, Chen Y, et al. The m(6)A-suppressed P2RX6 activation promotes renal cancer cells migration and invasion through ATP-induced Ca(2+) influx modulating ERK1/2 phosphorylation and MMP9 signaling pathway. J Exp Clin Cancer Res. 2019;38(1):233-249. doi: 10.1186/s13046-019-1223-y
- Ranzuglia V, Lorenzon I, Pellarin I, et al. Serum- and glucocorticoid- inducible kinase 2, SGK2, is a novel autophagy regulator and modulates platinum drugs response in cancer cells. Oncogene. 2020;39(40): 6370-6386. doi: 10.1038/s41388-020-01433-6
- Pao AC. SGK regulation of renal sodium transport. Curr Opin Nephrol Hypertens. 2012;21(5):534-540. doi: 10.1097/MNH.0b013e32835571be
- Liu Y, Chen J-B, Zhang M, et al. SGK2 promotes renal cancer progression via enhancing ERK 1-2 and AKT phosphorylation. Eur Rev Med Pharmacol Sci. 2019;23(7):2756-2767. doi: 10.26355/eurrev_201904_17549
- Baldewijns MM, van Vlodrop IJ, Vermeulen PB, Soetekouw PM, van Engeland M, de Bruine AP. VHL and HIF signalling in renal cell carcinogenesis. J Pathol. 2010;221(2):125-138. doi: 10.1002/path.2689
- Bostrom AK, Lindgren D, Johansson ME, Axelson H. Effects of TGF-beta signaling in clear cell renal cell carcinoma cells. Biochem Biophys Res Commun. 2013;435(1):126-133. doi: 10.1016/j.bbrc.2013.04.054
- Ma L, Li Y, Wu Y, et al. The construction of in vitro tumor models based on 3D bioprinting. Bio-Des Manuf. 2020;3(3):227-236. doi: 10.1007/s42242-020-00068-6
- Xu K, Huang Y, Wu M, Yin J, Wei P. 3D bioprinting of multi-cellular tumor microenvironment for prostate cancer metastasis. Biofabrication. 2023;15(3):035020. doi: 10.1088/1758-5090/acd960
- Xu K, Han Y, Huang Y, Wei P, Yin J, Jiang J. The application of 3D bioprinting in urological diseases. Mater Today Bio. 2022;16:100388. doi: 10.1016/j.mtbio.2022.100388
- Mao S, He J, Zhao Y, et al. Bioprinting of patient-derived in vitro intrahepatic cholangiocarcinoma tumor model: Establishment, evaluation and anti-cancer drug testing. Biofabrication. 2020;12(4):045014. doi: 10.1088/1758-5090/aba0c3
- Xie F, Sun L, Pang Y, et al. Three-dimensional bio-printing of primary human hepatocellular carcinoma for personalized medicine. Biomaterials. 2021;265: 120416. doi: 10.1016/j.biomaterials.2020.120416