Designing a 3D-printed medical implant with mechanically macrostructural topology and microbionic lattices: A novel wedge-shaped spacer for high tibial osteotomy and biomechanical study
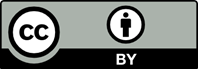
Metal three-dimensional (3D) printing has become an important manufacturing process in medical implant development. Nevertheless, the metal 3D-printed implant needs to be considered with structural optimization to reduce the stress-shielding effects and to be incorporated with a lattice design to generate better bone ingrowth environment. This study combines topology optimization (TO) and lattice design to acquire an optimal wedge-shaped spacer (OWS) for high tibial osteotomy (HTO) fixation. The OWS was manufactured using titanium alloy 3D printing to conduct biomechanical fatigue testing for mechanical performance validation. A solid wedge-shaped spacer (SWS) with three embedded screws was designed using the HTO model. An OWS was obtained under physiological loads through finite element (FE) analysis and TO. A deformed YM lattice with a porosity of 60% and pore size of 700 μm was filled at the OWS posterior region. The HTO mechanical performance was simulated for SWS, OWS, and commercial T-shaped plate (TP) fixations using FE analysis. The displacement/fracture patterns under OWS and TP fixations were verified using fatigue testing. The manufacturing errors for all 3D-printed OWS features were found to be less than 1%. The FE results revealed that the OWS fixation demonstrated reductions of 56.46%, 11.98%, and 64.31% in displacement, stress in the implant and bone, respectively, compared to the TP fixation. The fatigue test indicated that the OWS fixation exhibited smaller displacement for the HTO, as well as a higher load capacity, minor bone fracture collapse, and a greater number of cycles than the TP system. This study concluded that medical implants can be designed by integrating macro TO and microlattice design to provide enough mechanical strength and an environment for bone ingrowth after surgery. Both FE analysis and biomechanical fatigue tests confirmed that OWS mechanical performance with lattice design was more stable than the HTO TP fixations.
- Sutradhar A, Park J, Carrau D, Miller MJ. Experimental validation of 3D printed patient-specific implants using digital image correlation and finite element analysis. Comput Biol Med. 2014;52:8-17. doi: 10.1016/j.compbiomed.2014.06.002
- Guoqing Z, Junxin L, Chengguang Z, Juanjuan X, Xiaoyu Z, Anmin W. Design optimization and manufacturing of bio-fixed tibial implants using 3D printing technology. J Mech Behav Biomed Mater. 2021;117:104415. doi: 10.1016/j.jmbbm.2021.104415
- Guoqing Z, Junxin L, Xiaoyu Z, Yongsheng Z, Yuchao B. The design and processing of a 3D-printed high-performance biological fixation plate. Int J Bioprint. 2023;9:658. doi: 10.18063/ijb.v9i2.658
- Noroozi R, Tatar F, Zolfagharian A, et al. Additively manufactured multi-morphology bone-like porous scaffolds: Experiments and micro-computed tomography-based finite element modeling approaches. Int J Biopring. 2022;8(3):556. doi: 10.18063/ijb.v8i3.556
- Wang Z, Zhang M, Liu Z, et al. Biomimetic design strategy of complex porous structure based on 3D printing Ti-6Al- 4V scaffolds for enhanced osseointegration. Mater Des. 2022;218:110721. doi: 10.1016/j.matdes.2022.110721
- Wang YT, Chang CM, Liu PH, Lin CL. Feasibility evaluation of a new lattice for porous surface design in additive manufacturing medical implants under interfacial tensile bonded testing. Addit Manuf. 2023;66:103455. doi: 10.1016/j.addma.2023.103455
- Frosch S, Nüsse V, Frosch KH, Lehmann W, Buchhorn G. Osseointegration of 3D porous and solid Ti-6Al-4V implants-narrow gap push-out testing and experimental setup considerations. J Mech Behav Biomed Mater. 2021;115:104282. doi: 10.1016/j.jmbbm.2020.104282
- Chang B, Song W, Han T, et al. Influence of pore size of porous titanium fabricated by vacuum diffusion bonding of titanium meshes on cell penetration and bone ingrowth. Acta Biomater. 2016;33:311-321. doi: 10.1016/j.actbio.2016.01.022
- Liu X, Chen Z, Gao Y, zhang J, Jin Z. High tibial osteotomy: review of techniques and biomechanics. J Healthc Eng. 2019;2019:8363128. doi: 10.1155/2019/8363128
- Jang YW, Lim D, Seo H, Lee MC, Lee OS, Lee YS. Role of an anatomically contoured plate and metal block for balanced stability between the implant and lateral hinge in open-wedge high-tibial osteotomy. Arch Orthop Trauma Surg. 2018;138:911-920. doi: 10.1007/s00402-018-2918-9
- Yang JCS, Lin KY, Lin HH, Lee OK. Biomechanical evaluation of high tibial osteotomy plate with internal support block using finite element analysis. Plos One. 2021;16:e0247412. doi: 10.1371/journal.pone.0247412
- Slevin O, Ayeni OR, Hinterwimmer S, Tischer T, Feucht MJ, Hirschmann MT. The role of bone void fillers in medial opening wedge high tibial osteotomy: A systematic review. Knee Surg Sports Traumatol Arthrosc. 2016;24:3584-3598. doi: 10.1016/j.arthro.2022.11.039
- Song KY, Koh IJ, Kim MS, Choi NY, Jeong JH, In Y. Early experience of lateral hinge fracture during medial opening-wedge high tibial osteotomy: Incidence and clinical outcomes. Arch Orthop Trauma Surg. 2020;140:161-169. doi: 10.1007/s00402-019-03237-0
- Lee SJ, Kim JH, Baek E, Ryu HS, Han D, Choi W. Incidence and factors affecting the occurrence of lateral hinge fracture after medial opening-wedge high tibial osteotomy. Orthop J Sports Med. 2021;9:23259671211035372. doi: 10.1177/23259671211035372
- Seo SS, Kim OG, Seo JH, Kim DH, Kim YG, V IS. Complications and short-term outcomes of medial opening wedge high tibial osteotomy using a locking plate for medial osteoarthritis of the knee. Knee Surg Relat Res. 2016;28:289-296. doi: 10.5792/ksrr.16.028
- Röderer G, Gebhard F, Duerselen L, Ignatius A, Claes L. Delayed bone healing following high tibial osteotomy related to increased implant stiffness in locked plating. Injury. 2014;45: 1648-1652. doi: 10.1016/j.injury.2014.04.018
- Pape D, Dueck K, Haag M, Lorbach O, Seil R, Madry H. Wedge volume and osteotomy surface depend on surgical technique for high tibial osteotomy. Knee Surg Sports Traumatol Arthrosc. 2013;21:127-133. doi: 10.1007/s00167-012-1913-x
- Nyman JS, Roy A, Tyler JH, Acuna RL, Gayle HJ, Wang X. Age-related factors affecting the postyield energy dissipation of human cortical bone. J Orthop Res. 2007;25:646-655. doi: 10.1002/jor.20337
- Fan Z, Swadener JG, Rho JY, Roy ME, Pharr GM. Anisotropic properties of human tibial cortical bone as measured by nanoindentation. J Orthop Res. 2002;20:806-810. doi: 10.1016/S0736-0266(01)00186-3
- Mondal S, Ghosh R. A numerical study on stress distribution across the ankle joint: Effects of material distribution of bone, muscle force and ligaments. J Orthop. 2017; 14:329-335. doi: 10.1016/j.jor.2017.05.003
- Tuncer M, Cobb JP, Hansen UN, Amis AA. Validation of multiple subject-specific finite element models of unicompartmental knee replacement. Med Eng Phys. 2013;35:1457-1464. doi: 10.1016/j.medengphy.2013.03.020
- Morgan EF, Bayraktar HH, Keaveny TM. Trabecular bone modulus-density relationships depend on anatomic site. J Biomech. 2003;36:897-904. doi: 10.1016/s0021-9290(03)00071-x
- Du J, Li S, Silberschmidt VV. Remodelling of trabecular bone in human distal tibia: A model based on an in-vivo HR-pQCT study. J Mech Behav Biomed Mater. 2021;119:104506. doi: 10.1016/j.jmbbm.2021.104506
- Wu D, Isaksson P, Ferguson SJ, Persson C. Young’s modulus of trabecular bone at the tissue level: A review. Acta Biomater. 2018;78:1-12. doi: 10.1016/j.actbio.2018.08.001
- Sen A, Follet H, Sornay-Rendu E, Rémond Y, George D. Prediction of osteoporotic degradation of tibia human bone at trabecular scale. J Mech Behav Biomed Mater. 2023;139:105650. doi: 10.1016/j.jmbbm.2023.105650
- Wehner T, Claes L, Simon U. Internal loads in the human tibia during gait. Clin Biomech (Bristol, Avon). 2009;24:299-302. doi: 10.1016/j.clinbiomech.2008.12.007
- Mao S, Liu Y, Wang F, et al. Design and biomechanical analysis of patient-specific porous tantalum prostheses for knee joint revision surgery. Int J Bioprint. 2023;9:735. doi: 10.18063/ijb.735
- Somovilla-Gómez F, Lostado-Lorza R, Corral-Bobadilla M, Escribano-García R. Improvement in determining the risk of damage to the human lumbar functional spinal unit considering age, height, weight and sex using a combination of FEM and RSM. Biomech Model Mechanobiol. 2020;19:351-387. doi: 10.1007/s10237-019-01215-4
- McCartney W, MacDonald B, Andrei Ober C, Lostado-Lorza R, Somovilla Gómez F. Pelvic modelling and the comparison between plate position for double pelvic osteotomy using artificial cancellous bone and finite element analysis. BMC Vet Res. 2018;14:100. doi: 10.1186/s12917-018-1416-1
- Hara D, Nakashima Y, Sato T, et al. Bone bonding strength of diamond-structured porous titanium-alloy implants manufactured using the electron beam-melting technique. Mater Sci Eng C Mater Biol Appl. 2016;59:1047-1052. doi: 10.1016/j.msec.2015.11.025
- Maas S, Diffo Kaze A, Dueck K, Pape D. Static and dynamic differences in fixation stability between a spacer plate and a small stature plate fixator used for high tibial osteotomies: A biomechanical bone composite study. ISRN Orthop. 2013;2013:387620. doi: 10.1155/2013/387620
- Zimmermann EA, Schaible E, Bale H, Ritchie RO. Age-related changes in the plasticity and toughness of human cortical bone at multiple length scales. Proc Natl Acad Sci USA. 2011;108:14416-14421. doi: 10.1073/pnas.1107966108
- Hart NH, Nimphius S, Rantalainen T, Ireland A, Siafarikas A, Newton RU. Mechanical basis of bone strength: Influence of bone material, bone structure and muscle action. J Musculoskelet Neuronal Interact. 2017;17: 114-139.
- Calvo-Gallego JL, Gutiérrez-Millán F, Ojeda J, Pérez MA. The correlation between bone density and mechanical variables in bone remodelling models: Insights from a case study corresponding to the femur of a healthy adult. Mathematics. 2022;10:3367. doi: 10.3390/math10183367
- Nagaraja S. Microstructural Stresses and Strains Associated with Trabecular Bone Microdamage. Ph.D. thesis. Georgia Institute of Technology, Atlanta, GA, USA; 2006.
- Ertl JP, Barrack RL, Alexander AH, VanBuecken K. Triplane fracture of the distal tibial epiphysis: Long-term follow-up. J Bone Joint Surg Am. 1988;70:967-976.
- Rapariz JM, Ocete G, González-Herranz P, et al. Distal tibial triplane fractures: Long-term follow-up. J Pediatr Orthop. 1996;16:113-118. doi: 10.1097/00004694-199601000-00023
- Pape D, Lorbach O, Schmitz C, et al. Effect of a biplanar osteotomy on primary stability following high tibial osteotomy: A biomechanical cadaver study. Knee Surg Sports Traumatol Arthrosc. 2010;18:204-211. doi: 10.1007/s00167-009-0929-3
- Diffo Kaze A, Maas S, Waldmann D, Zilian A, Dueck K, Pape D. Biomechanical properties of five different currently used implants for open-wedge high tibial osteotomy. J Exp Orthop. 2015;2:14. doi: 10.1186/s40634-015-0030-4