The Molecular Docking Study of Potential Drug Candidates Showing Anti-COVID-19 Activity by Exploring of Therapeutic Targets of SARS-CoV-2
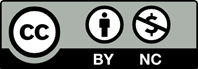
Objectives: The novel human coronavirus designated severe acute respiratory syndrome coronavirus 2 (SARS-CoV-2) first emerged in late 2019 in Wuhan, China. This virus spread rapidly around the globe, causing the respiratory illness called coronavirus disease 2019 (COVID-19). In view of the multiple threats and disorder posed by the pandemic, scientists around the world have been racing to understand SARS-CoV-2 and investigate the pathophysiology of this disease to find potential treatments and effective therapeutic drug candidates.
Methods: The virtual interaction of the COVID-19 main protease (Mpro) in complex with the inhibitor N3 (Research Collaboratory for Structural Bioinformatics Protein Data Bank [PDB] ID: 6LU7) with antiviral and antimalarial drugs was measured, as well as that of the SARS spike glycoprotein-human angiotensin-converting enzyme II (ACE2) complex (PDB ID: 6CS2) with antimalarial drugs currently on the market using the AutoDock Vina suite (O. Trott, The Scripps Research Institute, La Jolla, CA, USA).
Results: The binding energy result obtained from the docking of 6LU7 with ligands of oseltamivir, ritonavir, remdesivir, ribavirin, favipiravir, chloroquine, and hydroxychloroquine was found to be -4.7, -7.3, -6.5, -5.6, -5.4, -5.1, -5.3 kcal/mol, respectively. The binding energy from the docking of 6CS2 with ligands of chloroquine, and hydroxychloroquine was -7.1 and -6.8 kcal/mol, respectively. The docking results suggested drug molecules of oseltamivir, ritonavir, remdesivir, ribavirin, and favipiravir had a greater capability to inhibit SARS-CoV-2 since they demonstrated high affinity interactions with the COVID-19 Mpro in complex with the N3 inhibitor. Chloroquine and hydroxychloroquine also showed prominent binding interaction with the SARS spike glycoprotein-human ACE2 complex.
Conclusion: The results of this study suggest that these drugs are promising candidates for antiviral treatment with high potential to fight the SARS-CoV-2 strain.
1.Zhou P, Yang X-L, Wang X-G, et al. A pneumonia outbreak associated with a new coronavirus of probable bat origin. Nature 2020;579:270–3.
2. Wang C, Horby PW, Hayden FG, Gao GF. A novel coronavirus outbreak of global health concern. The Lancet 2020;395:470– 3.
3. Coronavirus disease 2019 https://www.who.int/emergencies/ diseases/novel-coronavirus-2019 Accessed: 2020-04-14.
4. Mcconkey B, Sobolev V, Edelman M. The performance of current methods in ligand-protein docking. Curr Sci;83.
5. Jorgensen WL. The many roles of computation in drug discovery. Science 2004;303:1813–8.
6. Bajorath J. Integration of virtual and high-throughput screening. Nat Rev Drug Discov 2002;1:882–94.
7. Langer T, Hoffmann RD. Virtual screening: an effective tool for lead structure discovery? Curr Pharm Des 2001;7:509–527.
8. Kitchen DB, Decornez H, Furr JR, Bajorath J. Docking and scoring in virtual screening for drug discovery: methods and applications. Nat Rev Drug Discov 2004;3:935–949.
9. Li G, De Clercq E. Therapeutic options for the 2019 novel coronavirus (2019-nCoV). Nature reviews. Drug discovery 2020;19:149–50.
10. Lim J, Jeon S, Shin HY, et al. Case of the Index Patient Who Caused Tertiary Transmission of COVID-19 Infection in Korea: the Application of Lopinavir/Ritonavir for the Treatment of COVID-19 Infected Pneumonia Monitored by Quantitative RTPCR. J Korean Med Sci 2020;35:e79.
11. Holshue ML, DeBolt C, Lindquist S, et al. First Case of 2019 Novel Coronavirus in the United States. N Engl J Med 2020;382:929–36.
12. Wang M, Cao R, Zhang L, et al. Remdesivir and chloroquine effectively inhibit the recently emerged novel coronavirus (2019-nCoV) in vitro. Cell Res 2020;30:269–71.
13. Yao X, Ye F, Zhang M, et al. In Vitro Antiviral Activity and Projection of Optimized Dosing Design of Hydroxychloroquine for the Treatment of Severe Acute Respiratory Syndrome Coronavirus 2 (SARS-CoV-2). Clin Infect Dis an Off Publ Infect Dis Soc Am [Epub ahead of print].
14. Lv Z, Chu Y, Wang Y. HIV protease inhibitors: a review of molecular selectivity and toxicity. HIV AIDS (Auckl) 2015;795–104.
15. Jin Z, Du X, Xu Y, et al. Structure of Mpro from COVID-19 virus and discovery of its inhibitors. Nature [Epub ahead of print].
16. Devaux CA, Rolain J-M, Colson P, Raoult D. New insights on the antiviral effects of chloroquine against coronavirus: what to expect for COVID-19? Int J Antimicrob Agents 2020;105938.
17. Kirchdoerfer RN, Wang N, Pallesen J, et al. Stabilized coronavirus spikes are resistant to conformational changes induced by receptor recognition or proteolysis. Sci Rep 2018;8:15701.
18. Lu R, Zhao X, Li J, et al. Genomic characterisation and epidemiology of 2019 novel coronavirus: implications for virus origins and receptor binding. Lancet (London, England) 2020;395:565–74.
19. Wan Y, Shang J, Graham R, Baric RS, Li F. Receptor Recognition by the Novel Coronavirus from Wuhan: an Analysis Based on Decade-Long Structural Studies of SARS Coronavirus. J Virol;94.
20. Li F, Li W, Farzan M, Harrison SC. Structure of SARS coronavirus spike receptor-binding domain complexed with receptor. Science 2005;309:1864–8.
21. Prajapat M, Sarma P, Shekhar N, et al. Drug targets for corona virus: A systematic review. Indian J Pharmacol 2020;52:56–65.
22. Belouzard S, Millet JK, Licitra BN, Whittaker GR. Mechanisms of coronavirus cell entry mediated by the viral spike protein. Viruses 2012;4:1011–33.
23. Li F. Structure, Function, and Evolution of Coronavirus Spike Proteins. Annu Rev Virol 2016;3:237–61.
24. Lindner HA, Fotouhi-Ardakani N, Lytvyn V, Lachance P, Sulea T, Ménard R. The papain-like protease from the severe acute respiratory syndrome coronavirus is a deubiquitinating enzyme. J Virol 2005;79:15199–208.
25. Jo S, Kim S, Shin DH, Kim M-S. Inhibition of SARS-CoV 3CL protease by flavonoids. J Enzyme Inhib Med Chem 2020;35:145– 51.
26. Shimamoto Y, Hattori Y, Kobayashi K, et al. Fused-ring structure of decahydroisoquinolin as a novel scaffold for SARS 3CL protease inhibitors. Bioorg Med Chem 2015;23:876–90.
27. Hilgenfeld R. From SARS to MERS: crystallographic studies on coronaviral proteases enable antiviral drug design. FEBS J 2014;281:4085–96.
28. Hsu M-F, Kuo C-J, Chang K-T, et al. Mechanism of the matu-ration process of SARS-CoV 3CL protease. J Biol Chem 2005;280:31257–66.
29. Barretto N, Jukneliene D, Ratia K, Chen Z, Mesecar AD, Baker SC. The papain-like protease of severe acute respiratory syndrome coronavirus has deubiquitinating activity. J Virol 2005;79:15189–98.
30. Liu X, Wang X-J. Potential inhibitors for 2019-nCoV coronavirus M protease from clinically approved medicines. bioRxiv [Epub ahead of print].
31. Han Y-S, Chang G-G, Juo C-G, et al. Papain-like protease 2 (PLP2) from severe acute respiratory syndrome coronavirus (SARS-CoV): expression, purification, characterization, and inhibition. Biochemistry 2005;44:10349–59.
32. Chang C, Lo S-C, Wang Y-S, Hou M-H. Recent insights into the development of therapeutics against coronavirus diseases by targeting N protein. Drug Discov Today 2016;21:562–72.
33. McBride R, van Zyl M, Fielding BC. The coronavirus nucleocapsid is a multifunctional protein. Viruses 2014;6:2991–3018.
34. Lin S-Y, Liu C-L, Chang Y-M, Zhao J, Perlman S, Hou M-H. Structural basis for the identification of the N-terminal domain of coronavirus nucleocapsid protein as an antiviral target. J Med Chem 2014;57:2247–57.
35. Chang C, Hou M-H, Chang C-F, Hsiao C-D, Huang T. The SARS coronavirus nucleocapsid protein--forms and functions. Antiviral Res 2014;10339–50.
36. Zhou B, Liu J, Wang Q, et al. The nucleocapsid protein of severe acute respiratory syndrome coronavirus inhibits cell cytokinesis and proliferation by interacting with translation elongation factor 1alpha. J Virol 2008;82:6962–71.
37. Cheung Y-K, Cheng SC-S, Sin FW-Y, Chan K-T, Xie Y. Induction of T-cell response by a DNA vaccine encoding a novel HLAA*0201 severe acute respiratory syndrome coronavirus epitope. Vaccine 2007;25:6070–7.
38. Kuo L, Hurst KR, Masters PS. Exceptional flexibility in the sequence requirements for coronavirus small envelope protein function. J Virol 2007;81:2249–62.
39. Venkatagopalan P, Daskalova SM, Lopez LA, Dolezal KA, Hogue BG. Coronavirus envelope (E) protein remains at the site of assembly. Virology 2015;47875–85.
40. Pervushin K, Tan E, Parthasarathy K, et al. Structure and inhibition of the SARS coronavirus envelope protein ion channel. PLoS Pathog 2009;5:e1000511.
41. Nieto-Torres JL, Dediego ML, Alvarez E, et al. Subcellular location and topology of severe acute respiratory syndrome coronavirus envelope protein. Virology 2011;415:69–82.
42. Schoeman D, Fielding BC. Coronavirus envelope protein: current knowledge. Virol J 2019;16:69.
43. Karpe YA, Lole KS. NTPase and 5’ to 3’ RNA duplex-unwinding activities of the hepatitis E virus helicase domain. J Virol 2010;84:3595–602.
44. Banerjee T, Aggarwal M, Sommers JA, Brosh RMJ. Biochemical and cell biological assays to identify and characterize DNA helicase inhibitors. Methods 2016;108130–141.
45. Three drugs fairly effective on novel coronavirus at cellular level - Xinhua | English.news.cn http://www.xinhuanet.com/ english/2020-01/30/c_138742163.htm Accessed: 2020-04-11.
46. Coronavirus outbreak: Cocktail of flu, HIV drugs appears to help fight virus, say Thai doctors - World News https://www. indiatoday.in/world/story/coronavirus-outbreak-cocktail-fluhiv-drugs-treatment-thai-doctors-1642783-2020-02-03 Accessed: 2020-04-11.
47. Singh AK, Singh A, Shaikh A, Singh R, Misra A. Chloroquine and hydroxychloroquine in the treatment of COVID-19 with or without diabetes: A systematic search and a narrative review with a special reference to India and other developing countries. Diabetes Metab Syndr [Epub ahead of print].
48. Warui DM, Baranger AM. Identification of specific small molecule ligands for stem loop 3 ribonucleic acid of the packaging signal Psi of human immunodeficiency virus-1. J Med Chem 2009;52:5462–473.
49. Cosconati S, Marinelli L, Trotta R, et al. Tandem application of virtual screening and NMR experiments in the discovery of brand new DNA quadruplex groove binders. J Am Chem Soc 2009;131:16336–7.
50. Trott O, Olson AJ. AutoDock Vina: improving the speed and accuracy of docking with a new scoring function, efficient optimization, and multithreading. J Comput Chem 2010;31:455– 61.
51. Pedretti A, Villa L, Vistoli G. VEGA-an open platform to develop chemo-bio-informatics applications, using plug-in architecture and script programming. J Comput Aided Mol Des 2004;18:167–73.